Death after traumatic injury has classically been described in terms of a trimodal distribution. Immediate and acute (<24 hours) deaths usually result from uncontrolled hemorrhage, but infections and multiple organ dysfunction syndrome, which often arise from infection, are responsible for a significant proportion of late deaths. Indeed, infection is responsible for most deaths in patients who survive longer than 48 hours after trauma.1 Trauma-related infections can be divided into those that result directly from the injury (eg, due to contamination that occurs in conjunction with the traumatic injury) and nosocomial infections that arise in the health care setting in conjunction with treatment of the injury. The pathogens involved can be exogenous or endogenous bacteria, depending on the mechanism of injury and/or the iatrogenic cause.
Most post-traumatic infections are polymicrobial, involving a mixture of aerobic and anaerobic organisms.2 In one series, 37–45% of all trauma patients experienced infectious complications during their initial hospitalization.3 In that same study, 80% of trauma patients who were in the intensive care unit (ICU) at least 7 days met the criteria for the systemic inflammatory response syndrome (SIRS). All caregivers need to understand the principles of surgical infections in the context of trauma patients. This chapter will review the following: factors that normally prevent infection, how trauma disrupts or overwhelms normal host defenses, recognition and treatment of the most common infectious complications after traumatic injury, principles of infection prevention, and how prophylaxis and prevention principles can be applied chronologically during the treatment of trauma patients.
Humans have evolved mechanisms to avoid infection despite the ubiquitous presence of bacteria in our environment and throughout our bodies. A recent review documented the magnitude and diversity of the human microbiota.4 Under normal circumstances there is a balance between our microbiota, intact environmental barriers, and host defenses (Fig. 18-1). Invasive surgical procedures or traumatic injury disrupts this balance and significantly increases the probability of developing an infection (Fig. 18-2). Microbes are abundant on the surface of the skin, within the oral cavity, and increase in numbers down the length of the gastrointestinal tract. Bacterial numbers differ at various locations, and the pathogenic species and their respective numbers at different anatomic sites are summarized in Table 18-1. Once inoculation of bacteria into normally sterile sites occurs, infection will ensue if bacteria proliferate faster than the host defense mechanisms eradicate them. Trauma generally results in a much greater disruption of normal barriers than occurs with elective surgery. Trauma may also have concomitant hypoperfusion (shock), devitalized tissue, and retained foreign bodies.
FIGURE 18-1
Under normal circumstances the determinants of infection, microbial factors, environmental factors, and host defenses interact such that there is no infection. (Adapted with permission from Meakins JL, et al. Host defenses. In: Howard RJ, Simmons RL, eds. Surgical Infectious Diseases. 2nd ed. Norwalk, CT: Appleton & Lange; 1988. Copyright © The McGraw-Hill Companies, Inc.)

FIGURE 18-2
(A) Under circumstances in which there is excessive microbial contamination, (B) serious disruption of environmental barrier, (C) impaired host defenses, or (D) all factors that ensure there will be an increased likelihood of developing infection (shaded intersection of determinants of infection).

Region | Microbes | Quantity |
---|---|---|
Skin (all areas) | Staphylococcus aureus and epidermidis Streptococcus (nonEnterococcal) | 102,3 |
Skin (infra-umbilical) | Streptococcus faecalis and faecium Escherichia coli S. aureus and epidermidis Streptococcus (nonEnterococcal) | 102,3,4,5 |
Oropharynx | Bacteroides (nonfragilis) Fusobacterium Haemophilus Peptostreptoccus S. aureus and epidermidis Streptococcus (nonEnterococcal) | 109,10,11 |
Stomach | Streptococcus (nonEnterococcal) Candida | 102,3 |
Proximal small intestine | Bacteroides fragilis and other Escherichia coli and other Enterobacteriaceae | 103,4,5,6,7 |
Distal ileum and Colon | Bacteroides (fragilis and other spp.) Escherichia coli and other Enterobacteriaceae Streptococcus faecalis and faecium | 105,6,7,8,9,10 |
Entry of microbes is normally limited by the integrity of environmental barriers, such as intact skin and the mucosa of the respiratory, gastrointestinal, and genitourinary tracts.5 The importance of intact skin is clearly evident when one considers the potential for microbial infection seen in burn patients or in patients with toxic epidermal necrolysis.2 Many traumatic injuries are associated with an alteration in the integrity of the skin. Even minor lacerations and abrasions have the potential to disrupt crucial environmental barriers. Interventions made in the process of caring for trauma patients, such as insertion of intravenous or urinary catheters, tube thoracostomy, etc., likewise disrupt the integument and may permit skin bacteria to access sterile sites. Studies have shown the quantitative number of microbes required to produce clinical infection is significantly decreased in the presence of foreign bodies, blood, or devitalized tissue, which are often present with traumatic wounds.2
The bacteria species responsible for clinical infections in surgical or trauma patients constitute a minority of the skin or gastrointestinal flora. These pathogenic bacterial species generally possess one or more virulence factors that facilitate infection and increase their pathogenicity. In contrast, the vast majority of endogenous and environmental bacteria are relatively nonpathogenic. For example, more than 99% of the colonic microbiota are nonpathogenic anaerobes that never cause clinical infections. Lactobacilli, which do not cause clinical infection, are the most abundant skin bacteria. In contrast, the most common pathogen associated with surgical site infections (SSI), Staphylococcus aureus, has numerous virulence factors that facilitate invasion and thwart host defenses. In the abdominal cavity, Escherichia coli and Bacteroides fragilis are the prototypical organisms associated with intra-abdominal infection, yet they account for only 0.01% and 1% of colonic bacteria, respectively. Under normal circumstances the overwhelming numbers of nonpathogenic bacteria constitute a robust “defense” against infection, because infection is proportionately less likely if >99% of the inoculum is incapable of producing infection. This concept of adherent resident bacteria preventing invasion has been termed colonization resistance.5 This is an important point because the skin and gastrointestinal microbial flora change considerably when trauma patients are hospitalized, both in terms of number and proportion of virulent bacteria and in terms of susceptibility to antibiotics, should an infection develop.
The skin microflora is relatively homogeneous, although bacterial numbers are higher in the axilla and groin areas. The endogenous skin bacteria are predominately gram-positive aerobic Staphylococcus and Streptococcus species, along with Corynebacterium and Propionibacterium.5 As noted above, S. aureus is the most common pathogen present on the skin, but increasingly isolates from trauma patients and community-acquired infections are methicillin resistant S. aureus (MRSA).6,7 This fact needs to be taken into account in terms of appropriate empiric or prophylactic antibiotic selection for these patients.7
The oral and nasopharynx harbor large numbers of bacteria, most frequently streptococcal species. Much smaller numbers of bacteria, typically 102–103 CFU/mL, are present in the normal stomach, because the normal acid pH of the stomach inhibits bacterial growth. Gastric bacterial numbers increase in the absence of gastric acid, as in patients receiving proton pump inhibitors. Bacterial numbers are much higher in the small intestine and the density of bacteria increases from the duodenum to the terminal ileum. Bacterial counts in the proximal gastrointestinal tract are in the range of 104–105 CFU/mL, whereas numbers in the terminal ileum are close to colonic densities (108–1010 CFU/mL). Bacterial numbers in the colon are even higher (1011–1012 CFU/mL) although most colonic bacteria are nonpathogenic. The presence of such large numbers is associated with very low oxygen tension, and 99.9% of bacteria present are anaerobes. The urogenital, biliary, pancreatic ductal, and distal respiratory tracts are “sterile” in healthy individuals.5
Host defense refers to endogenous factors that counteract microbial invasion. In addition to the environmental factors and colonization resistance described earlier, crucial humoral and cellular host defense mechanisms exist that eliminate bacteria within sterile spaces. Initially, several primitive and relatively nonspecific host defenses including proteins such as lactoferrin, fibrinogen, and complement begin to act against invading microbes. Lactoferrin sequesters the critical microbial growth factor iron, thereby limiting microbial growth. Fibrinogen within the inflammatory fluid has the ability to trap large numbers of microbes during polymerization into fibrin.5 Complement is activated on contact with bacteria and viruses, from tissue damage, or when IgG/IgM antibodies recognize microbial agents. Activation of complement releases C3a and C5a, which are potent chemotaxins that recruit neutrophils and macrophages. These components enhance endothelial adhesiveness and increase vascular permeability. Complement activation can directly destroy microbial agents via formation of a membrane attack complex (composed of complement proteins C5–C9) and enhance microbial phagocytosis by way of C1q and C3bi subunits. In vitro studies have shown that 50–70% of a moderate inoculum is eliminated prior to the influx of phagocytic host cells.
Many different tissues also contain resident innate immune cells. These include macrophages, dendritic cells, Kupffer cells, glial cells, mesangial cells, and alveolar macrophages.8 These innate immune cells express a wide variety of pathogen-associated molecular pattern (PAMP) receptors on their surface.9,10,11 The best known examples of PAMPs are the toll-like receptors (TLRs) of which there are now many, well-described receptor molecules.11 TLRs bind to ligands on bacteria (or damaged host tissue), and TLR binding results in activation of these cells. Activated macrophages secrete an array of substances in response leading to amplification and regulation of the acute proinflammatory response (Fig. 18-3). Sequential release of protein cytokines, including tumor necrosis factor-alpha (TNF-alpha), interleukin-1 (IL-1), IL-6, IL-8, and interferon-gamma (INF-γ), follows. These mediators produce the signs and symptoms that we associate with infection (fever, tachycardia, tachypnea, leukocytosis, etc.). IL-8 is a very potent chemoattractant for neutrophils, the innate immune cells primarily responsible for ongoing microbial phagocytosis and intracellular microbial killing. Unfortunately, the same process that recruits neutrophils and stimulates phagocytosis and oxidative killing may also contribute to damage of host tissues. Simultaneous with this proinflammatory response there is also production of anti-inflammatory mediators, such as IL-10 and transforming growth factor-β (TGFβ).12 Some of these mediators may contribute to the immune “hypo-responsiveness of trauma” over the ensuing days (Table 18-2).
FIGURE 18-3
Schematic depiction of a refined paradigm for pro- and anti-inflammatory responses after trauma or inflammation based on empirically observed alterations in gene expression after injury. (A) The previous paradigm had invoked an initial proinflammatory response followed by an anti-inflammatory response. (B) The updated paradigm demonstrates that both pro- and anti-inflammatory responses take place simultaneously, beginning at the time of the initial injury or insult. Patients who go on to develop complications and MODS have a different temporal response, with prolongation of both responses, but they do not have qualitative or fundamental differences in the nature of the inflammatory responses. (Figure from Xiao W, Mindrinos MN, Seok J, et al. Genomic storm. J Exp Med. 2011 Dec 19; 208(13):2581-90. doi: 10.1084/jem.20111354. Epub 2011 Nov 21. Used with permission.)

Type of cell and action | Change from normal |
---|---|
T and B lymphocytes | |
CD4+/CD8+ ratio | Decreased |
TGF-β production | Increased |
Th17 phenotype | Increased |
Immunosuppressive Treg phenotype | Increased |
Monocytes/macrophages | |
Immunocompetent activation | Decreased |
HLA-DR expression | Decreased |
Antigen-presenting capability | Decreased |
PGE2 production | Increased |
IL-12 production | Decreased |
IFN-γ release | Decreased |
Common characteristics of T cells and monocytes | |
Proportion of Th2 phenotype | Increased |
Trauma-induced apoptosis | Increased |
Polymorphonuclear neutrophils (PMN) | |
Chemotaxis | Decreased |
Phagocytic capacity | Decreased |
Release of elastase | Increased |
O2 radical release | Increased |
β-integrin expression | Decreased |
Leukotrien B4 production | Decreased |
Apoptosis | Delayed |
Specific anatomic locations have additional unique factors that defend against infection.13 For example, the peritoneal cavity has lymphatic channels on the undersurface of the diaphragm that facilitate removal of bacteria.14 The sub-diaphragmatic surface is a lower-pressure area due to the effect of respiratory excursion and this serves to move free fluid within the peritoneal cavity to this location. Movement of the diaphragm “pumps” this fluid into the thoracic duct and from there it gains rapid access to the systemic circulation. Experimental studies show that labeled bacteria inoculated into the peritoneal cavity appear in the thoracic duct within 6 minutes and in the bloodstream within 12 minutes.13 The respiratory tract has unique host defenses that help to ensure the sterility of the lung parenchyma, as well. Goblet cells within the respiratory mucosa secrete mucin that helps to trap bacteria. Ciliated respiratory epithelial cells move the mucus centrally where it, and the bacteria trapped within it, can be expectorated by coughing. The presence of endotracheal tubes, smoking, inhaled toxins, and some anesthetic agents interfere with mucocilliary clearance mechanisms, and this may predispose to pneumonia. Bacteria or other microbes that gain access to the alveoli are normally phagocytized by alveolar macrophages, although the macrophage activation that may accompany this process has been proposed as one possible pathogenic mechanism for acute lung injury (ALI) or adult respiratory distress syndrome (ARDS).15,16,17
To a very large extent the microbial agents responsible for infections or infectious complications after trauma are the same agents that cause most other surgical or ICU-associated infections. Table 18-1 shows the most common infectious agents that cause trauma-associated infections at various anatomic sites. Generally, Staphylococcus spp. and Streptococcus spp. are the most common pathogens responsible for infections in which the traumatic injury or operative intervention needed to treat the injury did not transgress a mucosal surface. For traumatic injuries that involve the aerodigestive tract the most common isolates are E. coli (43.4%), S. aureus (18.9%), Klebsiella pneumoniae (14.4%), and Enterococcus faecalis (5.6%).1 Hospitalized trauma patients develop nosocomial bacterial infections from the usual ICU-associated pathogens (Table 18-3). A few infectious agents that can be associated with trauma are seldom encountered in other settings including rabies virus, Clostridium tetani, and Vibrio spp. We will also discuss some of the unique challenges and concerns that have been brought to the attention of surgeons during the Ebola outbreak of 2014.
Organism/Class | % Total |
---|---|
Lactose-fermenting gram-negative bacillus | |
Escherichia coli | 8.1% |
Klebsiella sp. | 11.1% |
Enterobacter sp. | 7.6% |
Morganella sp. | 0.5% |
Citrobacter sp. | 1.0% |
Serratia sp. | 1.5% |
Lactose-non-fermenting gram-negative bacillus | |
Stenotrophomonas sp. | 2.0% |
Acinetobacter sp. | 8.6% |
Pseudomonas sp. | 13.6% |
Staphylococcus aureus | 31.8% total |
Methicillin-sensitive | 22.2% |
Methicillin-resistant | 9.6% |
Community pathogens | |
Streptococcus pneumonia | 2.0% |
Haemophilus sp. | 3.0% |
Other pathogens | |
Polymicrobial species | 3.0% |
Fungus | 1.5% |
Rabies is a rare, potentially fatal, clinical disease caused by the rabies virus. Rabies is an RNA virus present in the saliva of mammals and transmission to humans generally occurs following a bite from a rabid animal. Prior to the development of a vaccine by Louis Pasteur, bites from a rabid animal were uniformly fatal. In North America, raccoons, skunks, bats, foxes, coyotes, and bobcats are the primary reservoirs. Most patients with rabies have no documented exposure to a rabid animal, and the majority of these are associated with bat bites. Many victims underestimate the importance of a bat bite and a substantial portion do not even recall being bitten. Bats (Carnivora and Chiroptera) represent the ultimate zoonotic reservoir for the virus, as well. The rabies virus is highly labile and can be inactivated readily by ultraviolet radiation, heat, desiccation, and other environmental factors.
The word “rabies” derives from the Latin rabere meaning “to rage” and refers to the clinical manifestations of the disease that include hyperactivity, disorientation, hallucinations, and bizarre behavior. The rabies virus is neurotropic and causes an acute encephalitis. Other hallmarks of the disease include an intense fear of suffocation (eg, hydrophobia and aerophobia) secondary to intense laryngeal and pharyngeal spasm. Once the patient begins manifesting symptoms death is nearly certain. With increased vaccination and postexposure prophylaxis (PEP) over the past 50 years, the clinical disease is becoming increasingly uncommon. According to the Centers for Disease Control and Prevention (CDC) 49 cases of human rabies were reported in the United States between 1995 and 2011, with only 3 survivors. That said, it is important for the practitioner of emergency medicine/surgery to be knowledgeable about rabies since animal bites are encountered frequently in clinical practice.
Humans are not routinely vaccinated against rabies, while domestic animals receive routine rabies vaccinations. If a human is bitten by a rabid animal, rabies can be prevented by PEP before the virus enters the central nervous system during the incubation period. The diagnosis of rabies can be made rapidly by identification of rabies virus in the brain of a potentially infected animal. This procedure can be performed most expeditiously by euthanizing the suspected animal. If the rabies test is negative, then no postexposure vaccination or prophylaxis is needed. The incidence of positive rabies tests ranges from as high as 6–10% in wild animals down to levels of ~1% in domestic pets. An acceptable alternative approach, if the suspected source is a domestic pet (dog, cat, ferret, etc.), is for the offending animal to be quarantined and observed for 10 days. If the animal exhibits signs of rabies, the exposed person should begin PEP immediately and the animal should be euthanized and its brain tissue tested for rabies. If the animal is confirmed to have rabies, PEP must be completed; however, if the test results are negative PEP can cease.
Immediate measures that should be taken to decrease the risk of rabies transmission include thorough washing of bite and scratch wounds with soap and water, followed by application of povidone–iodine or alcohol. Human rabies immune globulin (HRIG) and rabies vaccine should be given in all cases except in persons who have been immunized previously.18 Immune globulin should never be delivered in the same syringe as the vaccine, as this will cause precipitation. The Advisory Committee on Immunization Practices (ACIP) of the CDC and the American Academy of Pediatrics recommend a single dose (20 IU/kg) of HRIG be given to provide protection for the first 2 weeks until the vaccine elicits an antibody response. Detailed and up-to-date information for rabies exposure is available on the CDC’s Web site (http://www.cdc.gov/rabies/index.html), and this site should be consulted for the latest information. The ACIP recommends a regimen of human diploid cell vaccine (Imovax®) for PEP on days 0, 3, 7, 14, and 28 along with a single dose of HRIG on day 0. Once initiated, rabies prophylaxis should not be interrupted or discontinued because of local or mild systemic reactions to the vaccine.
Tetanus is a rare, life-threatening condition that is caused by toxins produced by Clostridium tetani, a spore-forming, gram-positive bacillus.19 Clostridial spores can survive indefinitely, and they are ubiquitous in soil and feces. Under anaerobic conditions the spores can germinate into mature bacilli that elaborate the neurotoxins tetanospasmin and tetanolysin. Tetanospasmin produces most prominent clinical symptoms by interfering with motor neuron release of the inhibitory neurotransmitters gamma-aminobutyric acid (GABA) and glycine. The resulting loss of inhibition produces muscle spasm (usually spasm of the masseter muscle) and severe autonomic over-activity manifested by high fever, tachycardia, and hypertension. Historically, tetanus was highly fatal, but intensive medical therapy with neuromuscular blockade, mechanical ventilation, and ICU monitoring has lowered the case fatality rate to 11–28%. According to the CDC there were a total of 233 tetanus cases reported in the United States between 2001 and 2008, with 71.7% arising from acute trauma. Clinicians and trauma surgeons must remain alert for the potential of Clostridial contamination and provide appropriate, timely tetanus prophylaxis.19
The diagnosis of tetanus is made on clinical grounds alone, as there are no laboratory tests that can diagnose the condition or rule it out. Tetanus immunization is accomplished as a component of standard early childhood immunizations (diphtheria–pertussis–tetanus [DPT]), with administration of tetanus toxoid (TT) every 5–10 years to maintain immune memory (see, http://www.cdc.gov/tetanus/vaccination.html). No deaths have been reported in individuals who have been fully immunized. The CDC recommendations for tetanus prophylaxis depend on the wound characteristics and the prior immunization status of the patient. A wound with extensive contamination, one that is poorly vascularized, or with extensive soft tissue trauma is considered to be a “tetanus-prone wound.” A tetanus booster should be administered to patients who have received primary immunization, but who have not received TT during the past 10 years, or the past 5 years for tetanus-prone wounds.18 In patients who have never undergone primary immunization, human tetanus immune globulin (HTIG) should be administered along with TT at a different site. Antitetanus antibody binds to exotoxins and neutralizes their toxicity. High-risk groups such as the elderly, individuals with human immunodeficiency virus (HIV), and intravenous drug users (IVDU) who had received primary vaccination may not have tetanus antibodies, and more liberal use of HTIG should be considered in these groups.18
Vibrio vulnificus is a gram-negative rod present in seawater that can result in atypical, necrotizing soft tissue infections when traumatic injuries occur in the ocean.20,21,22 V. vulnificus is common in warm seawater and thrives in water temperatures greater than 68°F (20°C). The organism is not associated with pollution or fecal waste. Approximately 25% of V. vulnificus infections are caused by direct exposure of an open wound to warm seawater containing the organism. Exposure typically occurs when the patient is participating in water activities such as boating, fishing, or swimming. Infections are occasionally attributed to contact with raw seafood or marine wildlife. The risk of developing Vibrio infection is much higher in immunocompromised patients or patients with preexisting hepatic disease or diabetes mellitus.21 Established infection with V. vulnificus can be highly invasive with mortality rates of 30–40% and a mortality greater than 50% in immunocompromised patients. A published report from 2009 documented a 37% mortality rate even after implementation of a specific treatment guideline for necrotizing Vibrio infections.20
Patients with wound infections caused by V. vulnificus develop painful cellulitis that progresses rapidly.21,22,23 Physical examination will often reveal marked swelling and painful, hemorrhagic bullae surrounding traumatic wounds. In some cases, there can be rapid progression and associated systemic symptoms. Marked local tissue swelling with hemorrhagic bullae is characteristic. Systemic symptoms include fever and chills, and bacteria are present in the bloodstream in more than 50% of patients. Hypotension or septic shock may be an early symptom, and alterations in mental status occur in approximately one-third of patients. Table 18-4 summarizes clinical symptoms present in patients with Vibrio infection. It is important for trauma surgeons to be aware of the potential for Vibrio infections in the appropriate clinical setting, because antibiotic treatment is distinctly different from the agents typically employed for trauma patients. Aggressive surgical debridement, incision and drainage of purulent collections, and even amputation may be crucial adjuncts for management of these often severe soft tissue infections.21 Recent experience in 30 patients with documented Vibrio infection found that fasciotomy was needed in all patients, and 17% required amputation.20 Recommended antibiotics include doxycycline (100 mg iv/po bid), ceftazidime (2 g q 8 hours), cefotaxime (2 g q 8 hours), or ciprofloxacin (750 mg po bid or 400 mg iv q 12 hours).21,24
Traumatic injuries that occur in freshwater conditions may develop infections from Aeromonas hydrophila.23 A. hydrophila is a gram-negative anaerobic rod that is a common pathogen of fish and amphibians. Cutaneous inoculation of the organism can result in cellulitis, abscesses and, occasionally, necrotizing soft tissue infections. Like the situation with Vibrio infections, patients with hepatic disease and immune-compromise have a greater risk of developing generalized disease. A. hydrophila can be recovered from the bloodstream in a significant proportion of patients and this fact, along with a history of injury in fresh water, will aid in alerting clinicians to the correct diagnosis. Antibiotic agents active against A. hydrophila include third-generation cephalosporins, fluoroquinolones, doxycycline, or trimethoprim–sulfamethoxazole.23
On September 30, 2014, in the midst of the worst ebola virus disease (EVD) outbreak on record,25 the Centers for Disease Control (CDC) confirmed the first documented case of EVD in the United States in Dallas, Texas. Within 2 weeks a nurse who cared for that initial patient was also diagnosed with the disease. While EVD is not a surgical disease per se, surgeons knowledge and skills in resuscitation, critical care, and disaster management may be invaluable in the treatment of EVD. Therefore all surgeons should be familiar with the fundamentals of EVD management.
Ebola virus is a zoonosis belonging to the Filoviridae family. Five species have been identified as follows: Zaire, Sudan, Ivory Coast, Bundibugyo, and Reston.26 Ebola is a single-stranded RNA virus that sporadically spreads to humans from a presumptive animal or bat reservoir in the wild. Human spread only occurs through direct contact with infected bodily fluid, and the most infectious sources are blood, feces, and vomit.27 Ebola virus can remain viable on surfaces for 1–6 days, although the risk of transmission from a surface contact is considered very low.28,29 Humans are only contagious while they are symptomatic. Blood from patients with untreated late EVD harbor 109 virus particles per mL, making it highly infectious. This value can be compared to the much lower viral loads in patients with untreated HIV (105/mL blood) or Hepatitis C (5–20 × 106/mL blood), both of which are recognized as being highly transmissible.30 EVD is not an airborne pathogen, but, since it can be transmitted via large droplets, health care providers in close contact with symptomatic patients should take necessary droplet precautions (See Prevention).31
Ebola virus enters the body through breaks in the skin, mucus membranes or ingestion and then infiltrates cells, especially lymph tissues, liver, and spleen. The virus continues to replicate until cells become necrotic and lyse, spilling more viral particles into the circulation. Ebola virus elicits a profound proinflammatory cytokine and chemokine response producing a vigorous SIRS reaction.32,33 Significant endothelial injury ensues, with loss of vascular tone and increased vascular permeability leading to hypotension and shock.34,35 At the end stages of disease the virus is found in all body fluids and skin, making handling of bodies of EVD victims extremely hazardous. In contrast to prevailing biases, bleeding does not occur in all patients and only manifests late in the disease process as bleeding from skin, gums, and the gastrointestinal tract.36 Mortality rate remains high at 50–90% overall with a poorer prognosis seen in those with older age, diarrhea, hemorrhagic conjunctivitis, shortness of breath, confusion/coma, and hemorrhage.37
Patients in early stages of the disease present with the acute onset of flu-like symptoms. Fever is the most common symptom (present in 87%), with fatigue (76%), abdominal pain (44%), and nausea and vomiting (65%) frequently seen, also.37,38,39 Late symptoms are representative of a fulminant infection and SIRS with symptoms of severe vomiting and diarrhea, both of which may be bloody. Other late signs include a coagulopathy identified by diffuse oozing from mucosal surfaces and sites of intravenous lines. Death is often the result of recalcitrant multiorgan system failure.25
Currently there is no vaccine against or pharmacologic treatment for EVD, and the mainstay of therapy is supportive care. Many patients will have severe diarrhea, losing up to 15 L/day and require aggressive resuscitation efforts focused on replenishing circulatory volume. Significant electrolyte abnormalities are common, and correction of these must be done in order to prevent cardiac dysrhythmias. In the early stages of the disease patients can often tolerate oral fluids, antiemetics, and antidiarrheals. Patients with protracted vomiting or in those later stages of the disease should have intravenous fluids administered. Blood transfusions may be necessary, especially for those with hemorrhagic symptoms, and should follow current recommendations for transfusion triggers. Respiratory support in the form of mechanical ventilation may be necessary, but caution should be exercised with noninvasive support, as it may lead to aerosolization.40 Some novel antivirals known to have in vitro or animal anti-Ebola activity were used during the 2014 outbreak, but data regarding their effectiveness are inconclusive at this time. Experimental therapies used during the 2014 EVD outbreak included plasma from convalescent or immune patients and experimental monoclonal antibodies, but it is not clear that either method provided a survival benefit.41,42 Several vaccines are currently undergoing accelerated human trials, but none as yet are commercially available.43
The 2014 EVD outbreak clearly demonstrated how globalization has enhanced the capacity for a previously isolated tropical disease to rapidly spread. Prevention relies on maintaining a level of preparedness of providers and facilities that can receive patients with EVD, provide quality critical care, protect the providers, and assuage the fears of the community. Preventing EVD transmission is divided into mitigating transmission of the primary disease and screening for early identification of the disease as part of planning for a disaster.
Strict adherence to protocols for use of personal protective equipment (PPE) and adoption of practices to limit exposure to body fluids constitute the mainstays of primary prevention. Practiced use of full PPE, as described by the CDC (http://www.cdc.gov/vhf/ebola/healthcare-us/ppe/guidance.html) that is donned and doffed in the presence of a trained observer, is paramount when treating a patient with EVD.44 All practitioners who will come into contact with a patient with EVD should be trained and certified in their ability to properly wear full PPE prior to their first encounter with the patient. The body should be covered from head to toe with single use hood, gown, double gloves, gown (or coveralls), impermeable boot covers, and a respirator or N95 mask. Ideally extended cuff cloves, gowns with thumb hooks, and/or tape securing the gowns and gloves should be used. During contact with the patient, the practitioner should limit contact with surfaces with body fluid, keep their hands away from their face, and employ frequent alcohol-based scrubs on their gloved hands. There should be no rush or shortcuts taken while placing the PPE, so time consideration should be given if plans for procedures or intubation are required. Total time spent in the care of the patient while wearing PPE should be monitored because providers can become fatigued and dehydrated. Elective surgical procedure should be postponed in a patient with EVD and nonoperative alternatives should be strongly considered for urgent conditions (ie, appendicitis, perforated ulcer, etc). A risk-benefit analysis considering the risk of exposure to the operative team must be considered prior to operating for an emergent condition bearing in mind that, in late EVD disease, the patient is unlikely to survive.
Health care preparedness for a real or potential Ebola outbreak mandates the presence of an effective screening procedure that will capture any potential carrier of EVD. A robust program that contains multiple contingency plans and has been rehearsed by all the team members is essential, as disaster leaders should be prepared to operate without significant assistance for at least 72 hours. A simple screening method that can be utilized at any first encounter with a patient (including clerical areas) should focus on the following: (1) recent travel (last 21 days) to an affected area; (2) recent (21 days) direct contact with a patient infected with Ebola.45
Positive responses to these simple questions lead to an escalation of care with an immediate separation and isolation of the patient. After proper isolation, a more focused history identifying exposure risk can be performed. Once a diagnosis of EVD is confirmed, the CDC recommended plans should be in place to ensure immediate isolation is available which includes a private room with a door, a private bathroom, and separate areas for donning and doffing PPE. Other pragmatic plans for handling of lab specimens, environmental waste management, and restriction of nonessential personnel must be in place.46 The institutional management of threatened or actual Ebola outbreak requires trained, professional emergency managers and disaster management experts to be involved in incident command. Incident control should designate a team of site managers who have a constant presence to oversee implementation of safety precautions, monitor adequacy of necessary supplies, and evaluate care in isolation areas.
The high infectious risk and mortality rate of EVD evokes strong emotions of fear, both in healthcare workers and the general public. The incident command should remain the voice of calm and reason during the disaster and encourage constant communication between the front-line providers, the incident commander, and the community.
As in all other aspects of surgical care, it is preferable to try to prevent infections wherever possible. A number of interventions and practices have been demonstrated to be highly effective in preventing infections after elective operations, and many of those techniques have specific application in the care of injured patients. In this section, current evidence-based interventions that are applicable to trauma patients to prevent infection will be reviewed.
Prophylactic antibiotics are intended to prevent development of infection. The concept of prophylaxis presupposes that infection is not present. The decision to use prophylactic antibiotics and the choice of agents are based on the risk of developing a SSI. There are very good data regarding SSI rates for elective surgery, and the incidence of SSI by wound class for elective operations is shown in Table 18-5. Traditionally, Class I or clean wounds are those that do not violate the respiratory, alimentary, or genitourinary tracts. The wound infection rate is approximately 2%. Class II, or clean-contaminated wounds, refers to elective operations on potentially contaminated organs, such as the gastrointestinal tract, biliary tract, genitourinary tract, and respiratory tree (the procedure will violate a mucosal surface, which can never be completely sterile). The usual incidence of infection for these types of wounds is 5–10%. Contaminated wounds (Class III) differ from Class II wounds by the degree of spillage, with an incidence of infection of 15–30%. Finally, Class IV or dirty-infected wounds are characterized by frank pus or extensive and prolonged contamination. These wounds are characterized by an infection rate of >30% if primary closure is attempted.47 A recent report using ACS-NSQIP risk-adjusted data suggested substantially lower superficial and deep SSI rates, although the definitions used for class III and IV wounds are questionable.48 Emergent operative interventions increase the wound class by one step, so it is clear that higher rates of wound infection will be encountered in dealing with patients who have acute traumatic injuries that require operative intervention.
Wound class | Characteristics | Examples | SSI rate (%) |
---|---|---|---|
Clean (I) | Uninfected, no inflammation, no mucosal surface transected | Mastectomy Thyroidectomy Vascular bypass CABG | < 2% |
Clean/Contaminated (II) | Uninfected, no inflammation, no mucosal surface transected | Colectomy Cholecystectomy Laryngectomy Urologic procedures Pulmonary Lobectomy | 5–15% |
Contaminated (III) | Open accidental wounds, break in sterile conditions, spillage, stomas | Appendicitis Diverticulitis Small bowel GSW | 15–30% |
Dirty/Infected (IV)$ | Infection, perforation, devitalized tissue | Incise and drain abscess Peritonitis Enteric fistulas Remove infected implant | >30% |
In trauma surgery the majority of wounds encountered will be Class III or IV, and the luxury of a preinoculation dose of antibiotics, as recommended by the Surgical Care Improvement Project (SCIP), is usually unavailable.49 With this in mind, it is prudent to administer a single dose of an agent with activity against community-acquired aerobic and anaerobic pathogens as soon as possible for all patients requiring operation in the thorax or abdomen. Evidence-based guidelines for antibiotic prophylaxis of other surgical interventions or different anatomic sites are summarized in Table 18-6.
Intervention/injury | Agent/route/duration | Reference |
---|---|---|
Chest tube | First-generation cephalosporin prior to chest tube insertion. <24-h duration of antibiotic | (194, 195) |
Intra-ventricular pressure monitor | First-generation cephalosporin prior to insertion. Data unclear regarding duration of prophylaxis and impact on development of ventriculomeningitis. Prophylaxis may be continued in presence of CSF leak or concurrent extracranial infection | (196, 197) |
Basilar skull fractures | Evidence does not support prophylactic antibiotics to decrease risk of meningitis in presence of rhinorrhea or otorrhea | (198) |
Burns | Prophylactic systemic parenteral antibiotics are strongly discouraged | (199) |
Hand/tendon repairs | Single dose of first-generation cephalosporin may be beneficial. No evidence to support >24-h duration | (200) |
Mandibular fractures | No benefit to prolonged postoperative antibiotics | (201) |
Open globe Injuries | Recommendation to administer intravitreal and intravenous antibiotics to reduce endophthalmitis at the discretion of clinician | (130) |
Penetrating brain Injury | First-generation cephalosporin for 5 days | (202) |
Closed fractures | First-generation cephalosporin at time of ORIF, if needed, for no longer than 24 h | (203) |
Open fractures | Timing of debridement does not impact incidence of infection | (121) |
First-generation cephalosporin for 72 h or 24 h after wound closed | (204) | |
Consider addition of gram-negative antimicrobial coverage for Grade III fractures | (93) | |
No benefit to routine use of antibiotic beads | (93) | |
Combat injuries | Point of injury/delayed evacuation: Moxifloxacin 400 mg PO × 1 dose. Ertapenem 1 g IV or IM if penetrating abdominal injury, shock, or unable to tolerate PO medications | (205) |
Extremity Injury: Cefazolin 2 g iv q 6–8 h for 24–72 h | ||
Abdominal Injury: Cefazolin 2 g iv q 6–8 h plus metronidazole 500 mg iv q 8–12 h for 24 h after definitive washout |
The issue of postoperative continuation of prophylactic antibiotics in penetrating abdominal trauma has been investigated extensively. The Eastern Association for the Surgery of Trauma (EAST) has published guidelines derived from an evidence-based review.50 A single preoperative dose of a prophylactic antibiotic with broad-spectrum aerobic and anaerobic coverage is recommended for trauma patients sustaining penetrating abdominal wounds. Absence of an injury to a hollow viscus requires no further administration. If, however, a hollow viscus injury is present, there are sufficient Class I and Class II data to recommend continuation of prophylactic antibiotics for only 24 hours. Timely discontinuation of prophylactic antibiotics is important because the practice of prolonged administration has been linked to increased rates of subsequent nosocomial infections with resistant organisms.51 To maintain adequate tissue and serum levels of antibiotics in the face of ongoing hemorrhage and vasoconstriction, the administered dose may be increased 2- or 3-fold and repeated after every 10 units of transfusion of blood products, although there is not strong evidence to support this practice.
For many years povidone–iodine scrub was the standard disinfectant used for preparation of surgical sites and preoperative scrubbing by the surgical team. This dominance has been challenged by several well-designed studies performed in elective surgery showing significantly lower SSI rates with the use of chlorhexidine–alcohol compared with iodine (9.5% vs 16.1%).52 The fact that chlorhexidine–alcohol begins bacterial killing immediately on contact and does not require drying for antimicrobial effectiveness makes it potentially attractive for use in emergent surgery. One caveat regarding use of alcohol-based disinfectants is that it is imperative that the solutions be dry if electrocautery is used during surgical procedures to avoid intraoperative fires.
Glove perforation is an underappreciated phenomenon that may adversely impact the sterility of an operative procedure. Microperforation rates as high as 16% have been reported.53 When two pairs of gloves are used, inner glove perforation rate is substantially lower. In addition to patient outcome, the surgeon must consider personal safety. The CDC estimates that more than 2.7 million US citizens (~1%) have chronic HCV (http://www.cdc.gov/hepatitis/HCV/HCVfaq.htm) and that 1.2 million Americans are living with HIV (http://www.cdc.gov/hiv/basics/livingwithhiv/index.html). One epidemiologic study has reported the prevalence of HIV or hepatitis C to be as high as 20–65% and 10–45%, respectively, in an urban university hospital population for patients undergoing lymph node biopsy or drainage of a soft tissue infection.54 More recently, Brady et al.55 reported that the seroprevalence of undiagnosed hepatitis C virus (HCV) infection was 7.9% with another 7.8% of the population having preexisting HCV infection.
Hypothermia has been shown to be a strong prognostic indicator of poor outcome when considered in the context of the trauma “triad of death” (hypothermia, acidosis, coagulopathy).56 Hypothermia slows enzymatic activity needed for blood clotting and can induce an acquired coagulopathy. Hypothermia can also adversely impact SSI. In elective colorectal surgery a prospective, randomized study compared a group in whom intraoperative normothermia (36.6°C) was maintained to a control group with mild hypothermia (34.7°C). The normothermic group had a significantly lower rate of SSI (6% vs 19%) and a 20% shorter hospital stay.57 The precise mechanism for the beneficial effects of normothermia remains unclear, but may relate to tissue perfusion and improved host defense. Therefore, every reasonable effort should be made to maintain normothermia in all surgical patients.
The role of oxygen in development of SSI was examined in another prospective randomized study in patients undergoing elective colorectal operations. The authors observed a significantly lower SSI rate when 80% versus 30% inspired oxygen was delivered during the operation.58 It is noteworthy that other studies have failed to replicate these results59 and that this intervention has never been studied in a trauma population; however, an alternative interpretation of this study in elective patients is that it is best to avoid lower intraoperative FiO2.
There have now been several randomized controlled trials demonstrating a decreased incidence of SSI when antibiotic-coated sutures were employed for facial closure. The most rigorous study has involved triclosan-coated sutures, where a 30% decrease in SSI was seen in a large meta-analysis.60 Sutures decrease the inoculum of bacteria needed to establish infection and can serve as a foreign body within potentially infected wounds, so there is a sound physiologic basis for a potential benefit. An unanswered additional consideration is whether this intervention is cost-effective.
Autologous blood transfusion can be lifesaving for an exsanguinating patient, but numerous authors have reported worse infectious complications with increased blood utilization both in the immediate resuscitation61,62,63,64 and when used in a delayed fashion.65,66,67 Transfusion results in a multitude of immunosuppressive effects, including the following: (1) decreased CD3+, CD4+, and CD8+ cells; (2) overall reduced T-cell proliferation to mitogenic stimuli; (3) decreased natural killer cell activity; (4) defective antigen presentation; and (5) impaired cell-mediated immunity.68 The increased risk of infection associated with blood transfusion appears to be dose dependent,67,69 and logistic regression analyses report that the risk of infection increases 13% per unit transfused.70 Taylor et al.71 reported that for each unit of packed red blood cells (PRBCs) transfused, the odds of developing a nosocomial infection were increased by a factor of 1.5. The age of the transfused blood is an additional risk factor for infectious complications.72,73,74 As blood ages in the blood bank, it undergoes predictable changes that affect its ability to deliver oxygen. This “storage lesion” includes the following: (1) an increased affinity of hemoglobin for oxygen and reduced oxygen release to tissues; (2) depletion of 2,3-diphosphoglyerate (2,3-DPG) with resultant inadequacy of oxygen transport by red blood cells; (3) reduction in deformability, altered adhesiveness, and aggregability; and (4) accumulation of bioactive compounds with proinflammatory effects. In trauma patients, Offner et al. estimated that each transfused unit of RBC older than 14 days increased the risk of major infection by 13%.74
To minimize infectious risk blood transfusion in nonbleeding patients should be avoided. A large multicenter randomized study reported the safety of a restrictive transfusion strategy (trigger of 7.0 g/dL hemoglobin) compared to a liberal strategy (trigger of 10.0 g/dL). In fact, mortality rates patients who were younger (age <55) and less sick (APACHE <20) were more than double with liberal transfusion. When the subgroup of trauma patients (n = 203) was reviewed in a secondary analysis, McIntyre et al. confirmed the safety of the restrictive strategy.75 Additionally, practices to be avoided include transfusing multiple units of blood in stable nonbleeding patients, using blood as a volume expander, and transfusing blood preemptively in anticipation of future operative blood loss. Advanced age should not be used as a sole criterion to transfuse a patient. Several recent guidelines describe transfusion of autologous RBCs in trauma patients in the postresuscitation period.76,77
The timing, adequacy, and route of administration of nutrition to trauma patients have definite implications for infectious complications. Adequate nutrition is essential for patient recovery, healing of traumatic wounds, and post-trauma rehabilitation. This is because trauma causes increased metabolism and protein turnover that results in a catabolic state characterized by breakdown of skeletal muscle, impaired healing, and immunosuppression. Once acute resuscitation is complete, nutritional support should be instituted and the enteral route is preferred. Numerous trials have compared enteral nutrition (EN) with parenteral nutrition (PN). Advantages of the enteral route include lower cost, maintenance of function of the gut mucosal barrier, and more physiologic delivery of nutrients. The only real advantage of PN is the consistency of adequate calorie provision when the enteral route is unavailable. Based on numerous high-quality studies, the evidence strongly favors the use of EN over PN in regards to infectious complications.78,79,80 Traditional markers of nutrition (albumin, transferrin, and retinal-binding protein) are also restored better using EN.81 An additional factor that has been implicated in infectious complications is the issue of glycemic control. Current recommendations relating to glycemic control82 are in flux, but it appears clear that results are improved when hyper- and hypoglycemia are avoided. Enthusiasm for “very tight” glucose control has waned after several studies showed no benefit and increased complications with attempts to maintain blood glucose <110 mg/dL.83,84,85 Newer consensus documents favor continuous glucose monitoring and dynamic insulin protocols to optimize glucose control.86
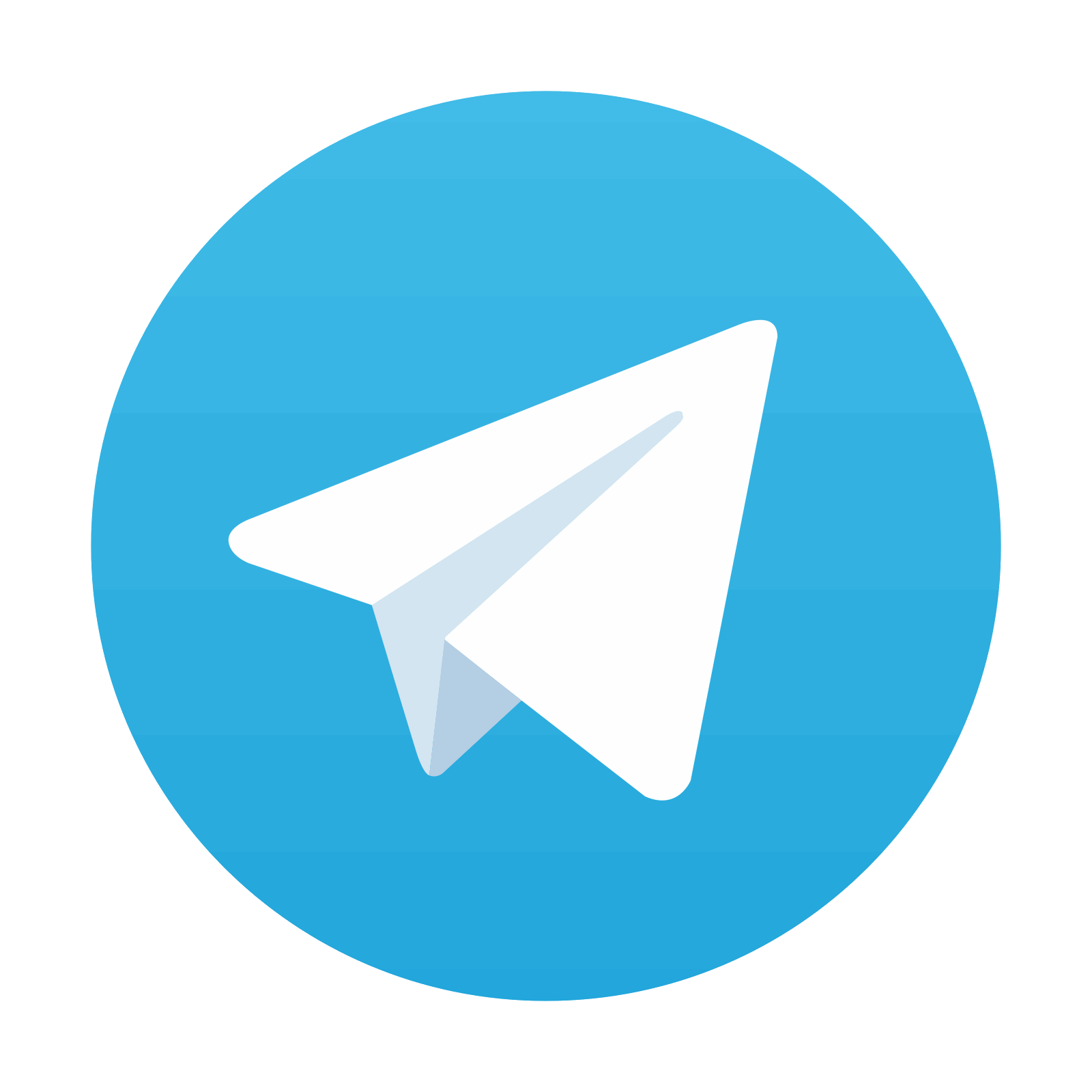
Stay updated, free articles. Join our Telegram channel

Full access? Get Clinical Tree
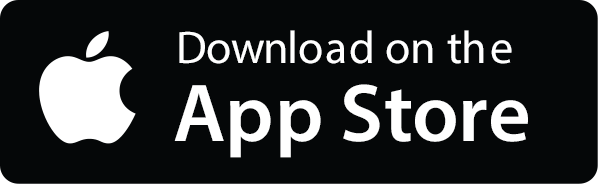
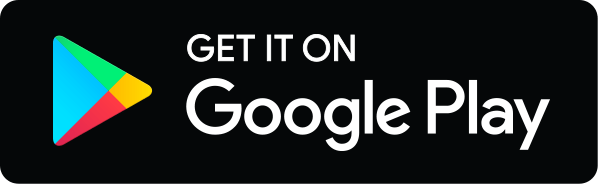