Primary TMA syndromes
Hereditary disorders
ADAMTS13 deficiency-mediated TMA (also called TTP)
Complement-mediated TMA
Coagulation-mediated TMA
Acquired disorders
ADAMTS13 deficiency-mediated TMA (also called TTP)
Shiga toxin-mediated TMA (also called ST-HUS)
Drug-mediated TMA (immune reaction)
Drug-mediated TMA (toxic dose-related reaction)
Complement-mediated TMA
Secondary TMA (common conditions associated with MAHA and thrombocytopenia)
Systemic infection
Malignancy
Preeclampsia, eclampsia, HELLP syndrome
Malignant hypertension
Autoimmune connective tissue disorders
Hematopoietic stem cell or solid organ transplantation

Fig. 6.1
Clinical algorithm for diagnosis of TMA-associated conditions
Pathological Findings of TMA
In general, the histologic features of TMA in the kidneys are similar and independent of the cause. Clinical history and laboratory data are critical when considering the possible etiologies. Cases of classic ST-HUS tend to have a characteristic clinical presentation and usually do not require a renal biopsy. Occasionally, TMA develops in the background of other pathologic abnormalities which may provide important histologic clues to the etiology. For example, features of lupus nephritis in combination with TMA might suggest SLE as the primary etiology. Similarly, inflammatory changes may be helpful in identifying cases of TMA associated with systemic infections and viral cytopathic changes may point to a specific viral etiology.
Gross Appearance
Gross evaluation typically occurs in fatal cases that require autopsy. Another context for gross assessment is in cases that progress to ESRD and also uncontrolled hypertension, which require bilateral nephrectomy for blood pressure control. In the early acute phase of the disease, the kidneys may be swollen and have areas of hemorrhage and cortical necrosis. Petechiae may be seen on the capsular surface and pelvic mucosa. In the late chronic stage, the kidneys can be reduced in size. Areas of old cortical necrosis may be recognized as retracted scars, which sometimes contain calcifications. Other chronic changes may include cystic degeneration and vascular changes of long-term dialysis.
Light Microscopy
Renal core biopsy is frequently used in the evaluation of TMA-associated conditions, particularly in cases where alternative diagnoses are being considered or the underlying etiology is not immediately apparent. Biopsy may also be useful in some chronic or relapsing cases to estimate the extent of chronic damage and ongoing active injury. Depending on the timing of the biopsy, the findings may be dominated by acute (early) or chronic (late) changes. Although these changes exist on a continuum, and significant overlap may be present in some cases, in general, acute changes are seen in biopsies taken within days to a couple of weeks after the disease onset, while chronic changes are more typical of biopsies performed weeks to months following the onset. Changes encountered in the autopsy specimens may be biased toward the severe end of the spectrum.
Early glomerular changes include capillary loop wall thickening and closure of the lumina by swollen endothelial cells. When prominent, this creates the characteristic appearance of bloodless glomeruli on the H&E stain, in which the tufts appear solidified and capillary loops cannot be easily distinguished from the mesangium (Fig. 6.2). Glomerular basement membrane (GBM) reduplication, which is best seen on PAS and silver stains, typically develops later in the disease. However, more severe cases may have rapid development of GBM double contours, which typically show irregular complex patterns (Fig. 6.3).



Fig. 6.2
Classic hemolytic uremic syndrome, ST-HUS. Endothelial cell swelling. The glomerulus sows closure of the capillary loops by endothelial cell swelling. Scattered endocapillary neutrophils are also seen (H&E)

Fig. 6.3
Classic hemolytic uremic syndrome, ST-HUS. Early glomerular basement membrane reduplication and mesangial edema. Subtle glomerular basement membrane double contours and more complex reduplication are seen. The mesangium appears swollen and spongiform (PAS)
Microthrombi within the glomerular capillary lumina are present in most cases of TMA, but the frequency and severity of this acute finding may vary significantly from case to case. In mild cases, focal fibrin accumulation may be seen along the internal aspect of the capillary walls in some of the loops (Fig. 6.4). More severe cases typically demonstrate large fibrin and platelet thrombi that may involve several capillary loops (Fig. 6.5). A common location for microthrombi formation is at the point of entry of the afferent arteriole into the glomerular tuft. These so-called infundibular thrombi may be quite large and create aneurysmal dilatation of the affected arterioles (Fig. 6.6). In some cases, such afferent arteriolar thrombi extend into the capillary walls or into the mesangium creating the appearance of fibrinoid necrosis. Karyorrhexis and small crescents with fibrin may also be present occasionally.




Fig. 6.4
Classic hemolytic uremic syndrome, ST-HUS. Endocapillary thrombi. The endocapillary thrombi appear as bright red (fuchsinophilic) aggregates along the inner aspects of the capillary loop walls on trichrome staining (Masson’s Trichrome)

Fig. 6.5
Classic hemolytic uremic syndrome, ST-HUS. Endocapillary thrombi. Large thrombi fill and expand the lumina of multiple glomerular capillary loops (H&E)

Fig. 6.6
Histologic changes of TMA. Infundibular thrombi. Two glomeruli show prominent infundibular thrombi (arrows). Glomerular capillary loop walls appear thickened, and occasional double contours are seen (H&E)
Glomerular capillary loop congestion by red blood cells is another common finding in TMA (Fig. 6.7), particularly in cases with significant extraglomerular arteriolar involvement. This finding is sometimes described as glomerular paralysis, because it is classically observed in combination with a thrombotic lesion in the afferent arteriole or terminal interlobular artery upstream of the involved glomerulus.


Fig. 6.7
Classic hemolytic uremic syndrome, ST-HUS. Glomerular congestion. The glomerular capillary loops are dilated and congested by red blood cells. Occasional endocapillary neutrophils are also present (H&E)
Fragmented red blood cells (schistocytes) may be a subtle finding in mild cases, but in most cases they are easily identified within the glomerular capillary loops, embedded in fibrin thrombi, and in the mesangium (Fig. 6.8).


Fig. 6.8
Histologic changes of TMA. Schistocytes. Fragmented red blood cells (schistocytes) are seen in some of the capillary loops and focally in the mesangium. Prominent endocapillary thrombi and apoptotic nuclear debris are also present (H&E)
Mesangial edema with spongiform mesangial appearance is sometimes present as an early finding and is usually not associated with mesangial hypercellularity (Fig. 6.3). Mesangiolysis with capillary loop microaneurysm formation is also occasionally observed, most commonly in more severe cases.
Arterioles, particularly afferent arterioles, and sometimes interlobular arteries may demonstrate luminal microthrombi with associated endothelial cell swelling, and subendothelial edema. In more severe cases, mural fibrinoid necrosis may be present (Fig. 6.9), but mural inflammation such as that seen in cases of leukocytoclastic vasculitis is not typically observed. With advancing chronicity, the arterioles and arteries develop prominent subendothelial intimal thickening due to proliferating smooth muscle and myointimal cells, with characteristic onion skin appearance (Fig. 6.10). Subendothelial accumulation of myxoid matrix, mucoid intimal hyperplasia, is also frequently present. In some cases, arteriolar glomeruloid lesions are detected, which resemble plexiform lesions of pulmonary arterial hypertension and are also thought to represent sequelae of prior thrombosis with subsequent recanalization.



Fig. 6.9
Histologic changes of TMA. Arteriolar thrombi and fibrinoid necrosis. Several arterioles demonstrate fibrin thrombi and mural fibrinoid necrosis (H&E)

Fig. 6.10
Histologic changes of TMA. Arteriosclerosis with mucoid hyperplasia in a chronic stage case. A terminal interlobular artery shows prominent medial thickening with “onion skin” appearance and mucoid intimal fibroplasia (H&E)
Chronic changes in the glomeruli vary depending on the severity of the acute injury and whether ongoing active disease is present. In cases without significant ongoing activity, the light microscopic findings become relatively nonspecific. Variable mesangial matrix accumulation with mild mesangial hypercellularity is usually present. GBM double contours on silver and PAS stains may be focal in mild cases or more widespread in more severe cases, resembling the pattern observed in membranoproliferative glomerulonephritis (Fig. 6.11). Focal segmental sclerosis may be observed as a sequela of prior glomerular injury. The adaptive (secondary) form of focal segmental glomerulosclerosis (FSGS) may develop in cases with significant chronicity. Ischemic glomerular changes are common and include thickening and wrinkling of the capillary walls, retraction of the tuft with widening of the Bowman’s space, thickening of the Bowman’s capsule, collagen “halos” inside the Bowman’s space, and periglomerular fibrosis. Significant hyaline arteriolosclerosis may develop as a result of arteriolar injury. Marked arteriosclerosis is also common, both in cases that develop hypertension as a consequence of renal failure and those with preexisting hypertension.


Fig. 6.11
Histologic changes of TMA. Glomerular basement membrane reduplication. The glomerular basement membranes show focal wrinkling, and reduplication can be seen in some of the loops (PAS)
Tubulointerstitial changes are largely nonspecific. In acute disease, variable amounts of red blood cells and red blood cell casts, and sometimes heme pigment can be seen in the tubules. This might be accompanied by mild tubular epithelial cell injury. Mild interstitial edema with sparse lymphocytic or lymphoplasmacytic inflammation may be present. Tubular epithelial protein resorption droplets may accompany cases with proteinuria. In severe cases, areas of cortical coagulative necrosis may occur (Fig. 6.12), which is thought to be ischemic in nature. Dystrophic calcifications are a common finding in such areas. Tubular atrophy and interstitial fibrosis develop with the progression of chronicity (Fig. 6.13).



Fig. 6.12
Histologic changes of TMA. Cortical necrosis. Wide area of cortical necrosis with infarct-like appearance is present (H&E)

Fig. 6.13
End-stage kidney disease following an episode of classic hemolytic uremic syndrome, ST-HUS. Subcapsular cortex is replaced by a thin layer of fibrosis with small obsolete glomerular “ghosts” still recognizable. A few severely ischemic glomeruli are seen deeper in the parenchyma. There is widespread tubular atrophy and interstitial fibrosis. Arteries and arterioles show prominent medial hypertrophy and intimal fibroplasia (H&E)
Immunofluorescence
The most prominent immunofluorescence (IF) finding in TMA is the presence of fibrinogen or fibrin staining in the areas that correspond to glomerular endocapillary microthrombi and thrombi in the extraglomerular vessels (Fig. 6.14). In mild cases, only focal linear fibrinogen staining along the endothelial aspect of the GBM may be detected. Conversely, more severe cases may feature segmental glomerular staining corresponding to large endocapillary thrombi and areas of fibrinoid necrosis. Extraglomerular arterioles and small arteries may show staining along the endothelium, within luminal thrombi, or transmural staining corresponding to areas of fibrinoid necrosis. Mesangial fibrinogen staining may also be observed.


Fig. 6.14
Clostridium difficile associated HUS. Fibrinogen immunofluorescence staining. Fibrinogen deposition is seen within the large endocapillary thrombi and in the mesangial areas (Courtesy of Dr. Tibor Nadasdy)
Variable staining for C3 and sometimes C1q complement proteins is usually observed in a distribution similar to fibrinogen. Nonspecific and usually focal staining with antibodies against immunoglobulins, particularly IgM, may also be observed, but significant staining for IgG, IgA, or kappa and/or lambda light chains is not a typical finding.
Several studies compared the composition of microthrombi in TMA cases associated with classic ST-HUS and TTP using IF staining with antibodies against fibrin or fibrinogen to detect fibrin thrombi and antibodies against vWF to detect platelet thrombi [12, 13]. Although more significant staining for fibrin products was reported in cases of HUS compared to TTP, and conversely vWF staining was more significant in cases of TTP compared to HUS, this approach has not found widespread application due to significant overlap in the staining patterns, particularly in cases where the etiology of TMA is uncertain.
Electron Microscopy
Ultrastructural evaluation is an important component in the diagnosis of TMA. It is particularly useful in chronic or subacute cases, where diagnostic features of acute injury may not be readily detected by light microscopy.
In acute phase, glomerular capillary loops demonstrate prominent endothelial cell swelling with diffuse endothelial cell separation from the glomerular basement membranes and subendothelial accumulation of electron-lucent flocculent or granular material (Fig. 6.15). The endothelial fenestrae often disappear because of endothelial swelling. The characteristic subendothelial widening is a consistent finding in TMA, which tends to persist beyond the early acute period and can be useful in cases lacking other morphologic features of TMA. With time, new GBM deposition by the endothelial cells results in easily identifiable GBM double contours. Complex multilayered reduplication may be encountered in some cases. Mesangial cell interposition, i.e., extension of the mesangial cell cytoplasm into the capillary loops along the glomerular basement membranes under the endothelial cells, develops soon after the initial injury and usually persists in chronic cases.


Fig. 6.15
Electron microscopy findings in TMA. Subendothelial widening. Endothelial cells show loss of fenestrae and endothelial cell cytoplasm separation from the glomerular basement membrane with accumulation of electron-lucent granular or flocculent material (arrow)
In the acute phase, electron-dense strands of fibrin are readily recognized within the capillary loops, often accompanied by variable numbers of platelets and fragmented red blood cells (Fig. 6.16). Mesangial rarefication with accumulation of granular material similar to that seen in subendothelial widening may be observed. Large dilated capillary loops may be visualized as a consequence of mesangiolysis. Podocyte foot process effacement is typically focal or patchy, not widespread.


Fig. 6.16
Electron microscopy findings in TMA. Endocapillary fibrin. Abundant irregular electron-dense fibrin strands are seen within a glomerular capillary loop (arrow). The capillary lumen is closed by swollen endothelial cells and necrotic/apoptotic cell debris. Significant podocyte foot process effacement is also present (arrowhead)
Findings in arterioles and small arteries resemble those seen in the glomerular capillary loops. These include endothelial cell swelling, endothelial separation from the basement membrane, and subendothelial accumulation of granular material. Endoluminal fibrin, platelets, and schistocytes may be identified. Basement membrane multilayering is sometimes observed in more chronic cases.
Shiga Toxin-Associated HUS (ST-HUS)
Epidemiology
In the 1980s, Shiga toxin-producing E. coli (STEC) emerged as the major etiologic factor in the development of classic HUS, first in two epidemic outbreaks reported by Riley et al. [14] and later in a series of sporadic cases described by Karmali et al. [15, 16]. Since then, several major epidemic outbreaks of STEC-associated HUS have been documented. In 1992–1993, a multistate outbreak in the United States was caused by STEC O157:H7 transmitted via contaminated hamburger meat at a fast food restaurant chain. Among 501 cases (median age 8 years; range 4 months–88 years), 45 developed HUS (9%), and 3 patients died [17]. Two large E. coli O157:H7 outbreaks occurred in Japan in 1990 [18] and in 1996 [19]. The 1996 outbreak in Osaka, Japan affected 12,680 school children with the median age of 7 years through contaminated school lunches. Among them, 121 patients developed HUS (0.9%), and 3 children died. Another outbreak occurred in the United States in 2008 among patrons of a buffet style restaurant in Oklahoma, which was caused by a non-O157 E. coli strain, STEC O111. This outbreak affected mostly adults (median age 44 years), with 26 cases of HUS (17%) and one death recorded among 156 confirmed cases [20]. A more recent outbreak involving an unusual hybrid strain of STEC O104:H4 in Germany in 2011 resulted in 3816 cases, 845 of which (22%) developed HUS (median age 42 years), and 54 patients died [21]. A retrospective analysis of STEC O157 outbreaks reported to the Centers for Disease Control and Prevention identified 350 outbreaks between 1992 and 2002 with 8598 cases total, among which 354 (4%) developed HUS [22]. Although data from North America and Western Europe indicate that STEC O157:H7 is responsible for the great majority (63–83%) of diarrhea-associated HUS cases [21, 23, 24], non-O157:H7 strains are responsible for a larger proportion of HUS cases worldwide [25, 26]. Additionally, emerging evidence from studies using direct detection of Shiga toxin in the stool indicates that non-O157:H7 STEC strains may account for 20–50% of all STEC infections in the United States [27]. The annual incidence of ST-HUS in the US, Canada, and Western Europe has been estimated at 1–20 cases per 1 million population [28–32], but is likely several fold higher in the regions where STEC strains are considered endemic [33].
Shigellosis is another well-established cause of classic HUS. Shiga toxin was originally characterized as an enterotoxin produced by Shigella dysenteriae serotype 1 strains [34]. It has been estimated that there are 165–250 million cases of Shigella infection causing 0.6–1.1 million deaths annually, with over 99% of the cases occurring in the developing countries where poor sanitation is thought to play a key role in the disease transmission [34, 35]. Although numerous Shigella species produce Shiga toxin and cause diarrheal illness, S. dysenteriae type 1 is responsible for a significantly higher proportion of cases with more severe clinical course and a higher proportion of cases of HUS [35, 36]. Most cases of Shigellosis and associated complications, including death, occur in children <5 years of age [35, 37, 38].
Clinical Course and Treatment
The average incubation time with STEC infection is 3–8 days, which varies depending on the strain [17, 19–21, 29]. Symptoms usually begin with abdominal cramps and diarrhea. Hemorrhagic diarrhea develops in 40–60% of epidemic cases, and up to 20% of cases progress to HUS (compared to <10% in sporadic cases) [39, 40]. Cases of hemorrhagic colitis not complicated by HUS are self-limiting and are not known to be associated with a long-term risk of hypertension or renal dysfunction [41]. Among STEC cases that do develop HUS, prodromal bloody diarrhea is present in about 70% of cases; fever occurs in 30% and vomiting in 30–60% of cases. Neurologic involvement, including stroke, seizures, or coma, is present in 25% of cases. Blood transfusions may be required in 70% of cases and dialysis in up 50% of cases [39, 42, 43]. STEC may be detected in the stool for several weeks after the symptoms resolve, particularly in children <5 years of age [44]. Risk factors associated with the development of HUS in STEC infections include bloody diarrhea, fever, elevated white blood count, vomiting, extremes of age, female gender, use of antimotility agents [39, 45]. In a meta-analysis study of 3476 patients with average follow-up of 4.4 years, patients who survived an episode of ST-HUS had an increased risk of long-term kidney dysfunction, including ESRD (12%) and significantly reduced GFR (25%) [43]. The outbreak of STEC O104:H4 in Germany in 2011 was characterized by unusually severe symptoms and outcomes compared to prior outbreaks, with 50% neurologic involvement, 20% frequency of seizures, 20% dialysis-dependent renal failure, and 6% mortality (vs. 1%) [46].
Treatment of ST-HUS is largely based on supportive management of anemia, renal failure, and fluid and electrolyte imbalances. Early intravenous fluid administration may limit the severity of renal dysfunction and need for dialysis [47]. Bowel rest is usually recommended in cases with bloody diarrhea, and antimotility agents should be avoided. Although an early study suggested that use of antibiotics in STEC infections may increase the risk of HUS by 17-fold [48], a meta-analysis of 26 studies failed to show a statistically significant correlation between antibiotic and HUS [49]. Nevertheless, with the exception of rare cases with bacteremia [50], antibiotics should be avoided in STEC colitis, as their use has not resulted in improved outcomes. Vigilant blood pressure control in patients with chronic kidney insufficiency following an episode of ST-HUS may be beneficial in slowing down the progression of renal function decline [51, 52]. An oral Shiga toxin-binding agent has been developed, but a prospective randomized clinical trial failed to demonstrate any clinical benefit of the therapy [53]. Plasma infusion, plasma exchange and intravenous IgG therapy have been used in critically ill patients on individual basis [54–57], but randomized studies are lacking to assess the efficacy of these interventions. Similarly, the use of anti-C5 monoclonal antibody eculizumab which showed promise in the initial trials [56, 58], has been put into question in follow-up studies [59], and a randomized prospective phase 3 trial is currently under way. Kidney transplantation should be considered in patients who progress to ESRD.
Presentation and clinical course of illness caused by S. dysenteriae type 1 are similar to those of STEC. An important exception might be a higher rate of bacteremia in Shigellosis (up to 6% vs. <1% in STEC) [60–62], and evidence that empiric antibacterial therapy in endemic regions shortens the duration of symptoms and reduces the incidence of complications [63, 64].
Pathologic Findings
Gross and histologic findings of ST-HUS are largely the same as those of other conditions characterized by TMA (see above). Although no specific histopathologic characteristics that distinguish ST-HUS from other TMA-associated conditions exist, a few typical observations are worth mentioning. Petechial subcapsular and parenchymal hemorrhages, which can be recognized both grossly and microscopically, are less common in HUS than in TTP [65]. Glomerular involvement is usually present. Capillary wall thickening is the earliest and most readily recognized abnormality. Mesangiolysis is present in most cases and may be subtle or very prominent in the more severe cases. Hilar and infundibular fuchsinophilic thrombi are common and are often surrounded by arteriolar fibrinoid necrosis. Focal crescents may be found in up to 5% of the cases [65]. Although cortical necrosis in HUS associated with STEC as well as S. dysenteriae type 1 is well documented in older autopsy series [1, 66], it likely develops only in a minority of cases with the most severe disease. Several studies have demonstrated that the presence of significant extraglomerular arterial involvement is an important prognostic factor associated with poor long-term clinical outcomes and more rapid progression to ESRD [67–70].
Mechanisms
The general underlying pathophysiological mechanisms that result in the development of TMA are thought to be shared by the different TMA-associated conditions and include endothelial cell damage and local activation of complement and coagulation pathways. In ST-HUS, Shiga toxin produces a multifactorial response mediated by both intrinsic properties of the toxin as well as host response mechanisms (Fig. 6.17).


Fig. 6.17
Mechanism of Shiga toxin-mediated hemolytic uremic syndrome (ST-HUS). Shiga toxin (Stx) binds to its cellular receptor Gb3 on the endothelial cells and induces endothelial cell injury through direct inhibition of translation and NF-ĸB dependent transcriptional changes. Direct ribosomal inhibition by Stx produces ribotoxic stress and leads to cell death. Activation of NF-ĸB induces expression of numerous cytokines and cellular adhesion molecules, which leads to local recruitment of inflammatory cells. Stx binding to endothelial cells is promoted by upregulation of Gb3 expression. Stx binds von Willebrand Factor (vWF) and induces formation of ultra-long vWF, which promotes platelet recruitment and aggregation. Concurrent activation of complement and coagulation pathways ultimately results in formation of thrombi
STEC strains produce two serotypically different types of Shiga toxin, Stx1 and Stx2, while Stx refers to the prototype Shiga toxin originally discovered in S. dysenteriae type 1. Stx and Stx1 are nearly identical with only a single amino acid difference in their protein sequence [71]. Stx1 and Stx2 amino acid sequences are only 56% identical, but the two toxins share the same domain structure and mechanism of action [72]. Multiple variants of the prototypical Stx1 (renamed Stx1a) and Stx2 (renamed Stx2a) have been identified, including Stx1c, Stx2c, Stx2e, Stx2f, and Stx2g, which share 84–99% sequence identity with the prototypes [73, 74]. Shiga toxins are encoded by diverse lambdoid bacteriophages, which are an important vehicle for horizontal gene transfer and rapid acquisition of pathogenicity in gram-negative bacteria. Stx genes encode two subunits, A and B, which assemble into AB5 configuration formed by a single A subunit which non-covalently inserts into a doughnut-shaped pentamer of B subunits [75, 76].
Both Stx/Stx1 and Stx2 interact with the same cellular receptor, glycosphingolipid globotriaosylceramide (Gb3, also known as CD77), on the surface of mammalian cells [77–79]. Binding of the B pentamer induces clathrin-independent endocytosis and retrograde transport of the toxin into the endoplasmic reticulum (ER), where the catalytic A subunit is released into the ER lumen [80, 81]. The A subunit is an RNA N-glycosidase that cleaves a specific adenine base in 28S ribosomal RNA (rRNA), which blocks elongation factor-dependent aminoacyl tRNA binding and inhibits translation [82–84]. In addition to the inhibition of protein biosynthesis, Stx-induced structural modification in the 3′ end of the 28S rRNA induces a stereotypic response pathway called ribotoxic stress, which results in activation of pro-apoptotic signaling cascades (including JNK1) and leads to cell death [71, 85].
It has also been shown that even at low concentrations of Shiga toxin with minor effects on protein biosynthesis, major signaling changes are induced in endothelial cells [86, 87]. These changes include upregulation of the NF-ĸB and TNFα pathways, which promote expression of numerous proinflammatory cytokines and chemokines (MCP-1, IL-8, IL-1, IL-6, CXCR4 and SDF-1) as well as various cell adhesion molecules (E-selectin, ICAM-1, VCAM-1, and PECAM-1). Together, these changes are thought to result in recruitment of inflammatory cells such as neutrophils, monocytes and macrophages, with further elaboration of proinflammatory cytokines and tissue factor (TF) leading to downstream activation of platelet adhesion, extrinsic coagulation pathway, and C3-dependent alternative complement pathway [88–94]. TNFα and IL-1 have also been shown to induce upregulation of the Stx glycolipid receptor, Gb3, in endothelial cells, which may further promote binding of Stx to the endothelial cells [95].
In vitro evidence suggests that Stx can directly interact with vWF and induce the formation of ultra-long vWF multimers (UL-vWF) [96]. Although the formation of UL-vWF is dependent on B subunits in both Stx1 and Stx2, the precise mechanisms by which Stx1 and Stx2 induce UL-vWF may be different [97, 98]. Independent of the mechanism, formation of UL-vWF promotes platelet aggregation and activation of the clotting cascade in a manner similar to TTP.
It has been noted that Stx2-producing STEC strains tend to produce severe disease, including neurological symptoms and HUS, more frequently than Stx1-producing strains [99]. These differences have been attributed to the differences in binding properties of the two toxins to their receptor. Stx1 and Stx2 use different epitopes on Gb3, and although Stx1 has a 10-fold higher affinity for the receptor, Stx2 has a 200-fold slower dissociation from the receptor [100, 101].
Streptococcus Pneumoniae-Associated HUS (SP-HUS)
Severe systemic infections caused by S. pneumoniae, including pneumonia and less frequently meningitis, are the most common cause of non-diarrheal (D−) HUS. A study from the United States estimated that SP-HUS accounts for up to 38% of D− HUS and up to 4.7% of all HUS cases [2]. SP-HUS affects predominantly children under 2 years and is associated with high morbidity. A recent and largest to date retrospective study from North America of 37 cases of SP-HUS occurring between 1997 and 2009 reported 3% mortality [102] compared to up to 25% mortality in older studies [103–105]. In that study, 95% of patients required admission to the intensive care unit, 73% required dialysis during hospitalization, 23% remained dialysis dependent after 6 months of follow-up, and 10% underwent renal transplantation. The clinical outcomes are strongly dependent on prompt initiation and the effectiveness of antibacterial therapy.
Several studies from the US, Canada, and the UK documented an increase in annual incidence of SP-HUS following the introduction in 2000 of the heptavalent pneumococcal conjugate vaccine (PCV-7) [106–108]. Following 2001, serotype 19 which was not included in PCV7 has emerged as the most prevalent serotype associated with invasive S. pneumoniae infections. The serotype replacement has been argued to have occurred as a result of the introduction of the vaccine and/or antibiotic selection [109–111]. The more recently introduced broader-coverage PCV-10 and PCV-13 have been estimated to produce a significant reduction in the incidence of invasive infections caused by vaccine serotypes [112]. However, serotype tracking data following the introduction of the 10 and 13-valent vaccines, also suggests rapid emergence of non-vaccine strains [113–117], including those with multidrug antibiotic resistance [113, 117].
The proposed mechanism of SP-HUS involves cleavage of sialic acid on the surface of the red blood cells, platelets, and renal endothelial cells by the bacterial neuraminidase to expose Thomsen–Friedenreich antigen (Gal-GalNAc, also known as T-antigen) [104]. Naturally occurring cold IgM antibodies cause erythrocyte agglutination in vitro, which is the reason for positive Coombs test in SP-HUS unlike other forms of HUS [104, 118, 119]. Although T-antigen is thought to be involved in the pathogenesis, the role of anti-T IgM antibodies has been questioned [120]. A recent study examined the role of complement in five SP-HUS patients and found significantly decreased levels of the classical and alternative complement pathway components during the acute phase of the disease [121], suggesting acute activation of the complement pathways and consumption of its components. Importantly, three of the five patients also showed evidence of genetic alterations in the complement genes, including a previously described variant of Factor I (P50A) and two novel variants in Factor H (R1149X) and Thrombomodulin (T44I). Although these results provide novel evidence of complement activation in SP-HUS, a mechanistic understanding of the events leading to this activation is currently lacking. Additionally, these findings provide further evidence that the pathogenesis of many forms of TMA is multifactorial and that abnormal activation of complement is an important component in many forms of TMA with various triggering etiologic factors.
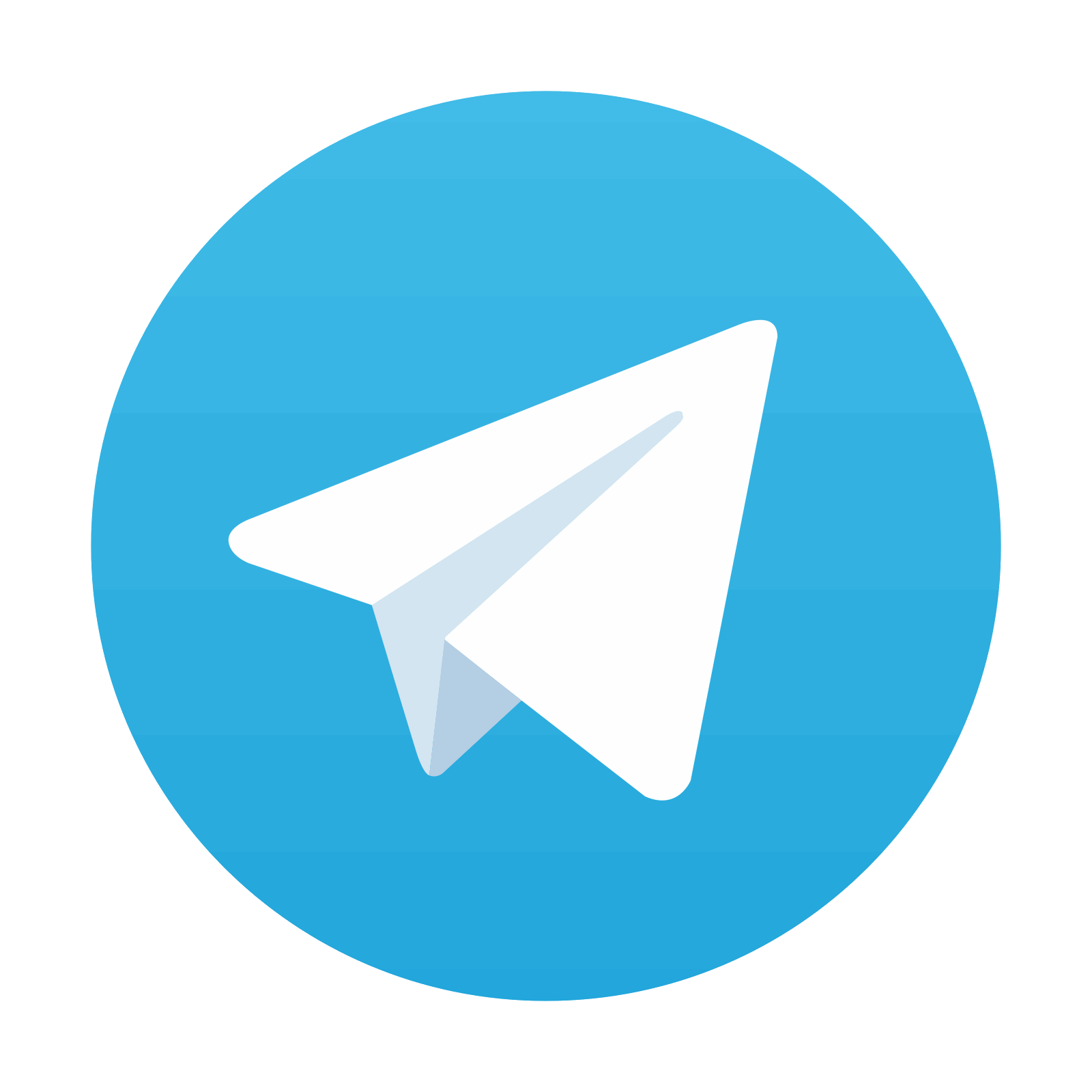
Stay updated, free articles. Join our Telegram channel

Full access? Get Clinical Tree
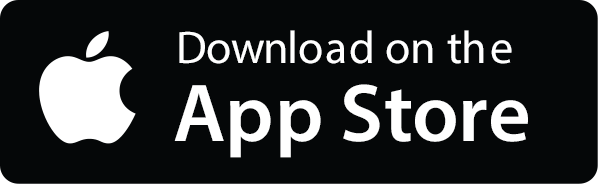
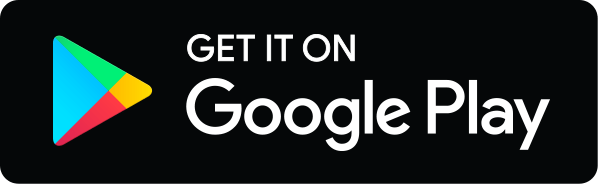