Antibiotic treatment alters the composition and metabolic function of the intestinal microbiota. These alterations may contribute to the pathogenesis of necrotizing enterocolitis (NEC) and antibiotic-associated diarrhea (AAD). Recent studies are beginning to unravel the contribution of specific groups of microbes and their metabolic pathways to these diseases. Probiotics or other microbiota-targeted therapies may provide effect strategies to prevent and treat NEC and AAD.
Key points
- •
Antibiotics induce microbial dysbiosis.
- •
Neonatal intestinal dysbiosis may contribute to necrotizing enterocolitis (NEC).
- •
Microbiome information may help predict risk for antibiotic-associated diarrhea (AAD) and NEC.
- •
Microbiome modulation may help prevent disease.
- •
Antibiotics induce AAD by disrupting microbiota’s metabolic functions.
Introduction
Antibiotics are commonly prescribed medications that have saved countless lives, yet their side effects pose significant health challenges. Antibiotics are the most frequently prescribed medications in children and constitute a significant amount in adults. Antibiotics function by either direct killing or inhibiting growth of bacteria. In either case, they work in conjunction with the host’s immune system to resolve infections.
Antibiotics and the Microbiome
The intestinal microbiome is a complex ecosystem in which there is tremendous interdependence and crosstalk between microbial species, and between the microbes and their host. Although antibiotics target specific types of microbes (eg, vancomycin and gram-positive organisms), their effects on the microbiome go beyond just those clinically targeted microbes. For example, removing certain species of bacteria opens niches for other microbes to expand, which, in turn, can result in microbiome disruptions or microbial dysbiosis, such as when treatment with the gram-positive microbe-targeted antibiotic vancomycin leads to loss of some gram-negative taxa. Not all antibiotics affect intestinal microbiota to the same degree. For example, vancomycin and metronidazole both drastically change the composition of the microbiota (in different ways) but the overall bacterial density is less following metronidazole treatment yet remains the same following vancomycin treatment. The route of exposure also matters because parenteral antibiotic treatment can affect the intestinal microbiome via biliary excretion of antibiotic into the intestinal lumen. Thus, although antibiotics are intended to target specific pathogenic microbes, their effects can be much more extensive, long-lasting, and unpredictable. Antibiotic-induced dysbiosis contributes in the shorter term to antibiotic-associated diarrhea (AAD) and is epidemiologically linked to a variety of longer-term health problems, including obesity, asthma, allergy, and inflammatory bowel disease.
Microbiome of the Neonate
Neonates face enormous challenges at parturition, including developing tolerance to their new microbiota while maintaining immunity against infection. The initial colonization of the gastrointestinal (GI) tract is an intricate balance between the colonization of commensal bacteria that leads to the establishment of tolerance and the prevention of infections secondary to the selective recognition of pathogenic microbes by the host. These host-microbial interactions are critical for the development and function of both the GI tract and the immune system. For example, the microbiota of the GI tract regulates angiogenesis, enterocyte proliferation, and proper crypt formation, along with development and function of gut-associated lymphoid tissue and the intestinal T cell populations that prevent intestinal inflammation. In a healthy neonate, this early crosstalk between commensal bacteria and the host leads to pathogen recognition, epithelial barrier maturation, immune system development, and development of tolerance to food antigens and commensal bacteria.
Microbial exposures early in ontogeny are associated with a range of diseases from atopy and autoimmune disorders to obesity and cancer. This process is thought to occur either through epigenetic epithelial and/or immune system changes or by providing a niche for specific microbial colonization that influences long-term health outcomes. However, exactly how the microbiome is established, and the impact of prenatal and postnatal exposures on the development of the microbiome, is only starting to be elucidated but offers great promise in predicting, preventing, and treating a variety of diseases.
The neonatal GI tract rapidly becomes colonized with microbiota. Newborn’s initial microbiota is acquired by vertical transmission of the maternal microbiome during delivery, although there is evidence for and against low-level microbial colonization of the placenta in utero. The mode of delivery, either via vaginal or caesarian section, influences the acquisition of most of the initial microbes. As such, prenatal factors that affect the maternal microbiome also influence the newborn’s microbiome.
The neonatal microbiome follows a general developmental process although significant interindividual variation is prominent. In the first month, facultative anaerobic microbes from the Enterobacteriaceae family, a large group of gram-negative bacteria that includes pathogens such as Escherichia coli along with nonpathogenic bacteria, dominates the neonatal microbiome. Over the next few months, the Enterobacteriaceae are succeeded by anaerobic bacteria, including the families Bifidobacteriaceae, Bacteroidaceae, Lachnospiraceae, and Ruminococaccea. Around the time of weaning, a varied mixture of bacterial families are present, including Clostridiaceae. One way to monitor the development of the microbiome is to use microbial ecology concepts, such as alpha diversity, that describe the number and distribution of species present in a given individual. For example, alpha diversity is lower in infants than adults, reflecting the higher number of microbial species in the adult microbiome. The microbiome of neonates and infants is rapidly changing but stabilizes into an adult-like microbiome by 3 years of age. A multitude of factors, such as the maternal microbiome, mode of delivery, diet, and antibiotic exposure, influence this process.
Moreover, murine models suggest that, not only is the fetus exposed to the maternal GI microbiome before delivery but that colonization of mice during gestation has direct effects on the development of the offspring’s immune system. These data raise the possibility that prenatal exposure to antibiotics or other means of altering maternal microbiomes can have profound implications for their offspring. In support of this, prenatal exposure to antibiotics has been associated with increased risk for obesity and asthma.
Mode of delivery (cesarean section vs vaginal) also shapes the neonatal microbiome. The microbiome of infants born vaginally is characterized by fecal resident microbes such as Bifidobacterium longum and Bifidobacterium catenulatum , whereas infants delivered by caesarian sections have environmental microbes as their predominant microbiota. This difference is long-lasting and can be seen even in older infants and children. Moreover, studies have suggested a link between the caesarian-section–associated microbiome and long-term outcomes, such as asthma, gastroenteritis, celiac disease, and diabetes.
Infant diet also contributes to the development and composition of the microbiome. The microbiomes of breastmilk-fed and formula-fed infants are quite distinct. Breastmilk is high in prebiotic compounds, such as human milk oligosaccharides, that enhance bacterial growth. It also contains live bacteria not found in formula that can influence the microbiome. The microbiome of neonates fed a breastmilk diet is more abundant in Bifidobacteria and Lactobacillus . Surprisingly, several studies have found that, although breastfed infants have higher bacterial counts, they have a lower species diversity than formula fed infants.
Antibiotic Effects on the Microbiome
Postnatal exposure to antibiotics is another important factor that shapes the microbiome. Antibiotic treatment decreases alpha diversity of the individual’s microbiome. For example, a seminal study in 3 healthy adults showed that 5 days of standard dose (500 mg twice a day) antibiotic treatment with ciprofloxacin had a significant effect on roughly one-third of the bacterial taxa identified in the study. However, most of these disturbances only lasted approximately 4 weeks, although some taxa were still missing at 6 months after treatment. Additional studies have corroborated these finding and demonstrated that some antibiotics have even more profound and long-lasting disruptions of the microbiome. Further, antibiotic exposure in infants and young children may have significant impacts on the microbiota during critical periods of development. For example, the microbiomes of infants exposed to ampicillin and gentamicin perinatally showed a decrease in Actinobacteria, Bifidobacterium and Lactobacillus and an increase in Proteobacteria at 4 weeks of age, and continued to have a decrease in the alpha diversity of these species even by 8 weeks of age. In a longer study that evaluated the microbiota of 43 infants form birth to age 2, Bokulich and colleagues observed that early antibiotic exposure led to a decrease in the microbiome’s alpha diversity and specific deficits in the Clostridium and Ruminococcus species. Moreover, they showed that early antibiotic exposure decreased stability and delayed the maturation of the intestinal microbiome. Similarly, another longitudinal cohort of children from birth to age 3 also showed a decrease in the alpha diversity of the microbiota of children exposed to antibiotics. Additionally, they showed that the species found in the microbiota of children exposed to antibiotics was dominated by a single strain rather than having multiple strains of the same species and, similarly to the previous study, had deficits in clostridium species. Finally, antibiotic-exposed microbiota had an expansion of antibiotics resistance genes ( Fig. 1 ).
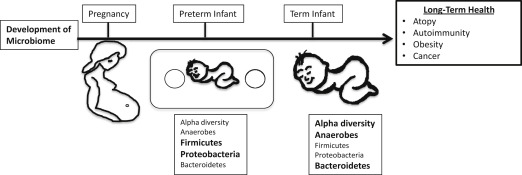
Microbiome of the Preterm Infant
Preterm infants face a more difficult challenge to maintain homeostasis with their developing microbiomes. They have immature immune and GI systems, commonly receive multiple courses of antibiotics, and have abnormal feeding patterns. Not surprisingly, the colonization patterns of the GI tract differ in preterm and term infants. The diversity of the premature infant’s microbiota is even more limited than in full-term neonates, where many of the detectable species in premature infants’ microbiomes are known neonatal pathogens. Additional differences include fewer anaerobes, increased abundance of Firmicutes and Proteobacteria, and decreased abundance of Bacteroides, as well as a substantial delay in bifidobacterial colonization when compared with full-term neonates.
Introduction
Antibiotics are commonly prescribed medications that have saved countless lives, yet their side effects pose significant health challenges. Antibiotics are the most frequently prescribed medications in children and constitute a significant amount in adults. Antibiotics function by either direct killing or inhibiting growth of bacteria. In either case, they work in conjunction with the host’s immune system to resolve infections.
Antibiotics and the Microbiome
The intestinal microbiome is a complex ecosystem in which there is tremendous interdependence and crosstalk between microbial species, and between the microbes and their host. Although antibiotics target specific types of microbes (eg, vancomycin and gram-positive organisms), their effects on the microbiome go beyond just those clinically targeted microbes. For example, removing certain species of bacteria opens niches for other microbes to expand, which, in turn, can result in microbiome disruptions or microbial dysbiosis, such as when treatment with the gram-positive microbe-targeted antibiotic vancomycin leads to loss of some gram-negative taxa. Not all antibiotics affect intestinal microbiota to the same degree. For example, vancomycin and metronidazole both drastically change the composition of the microbiota (in different ways) but the overall bacterial density is less following metronidazole treatment yet remains the same following vancomycin treatment. The route of exposure also matters because parenteral antibiotic treatment can affect the intestinal microbiome via biliary excretion of antibiotic into the intestinal lumen. Thus, although antibiotics are intended to target specific pathogenic microbes, their effects can be much more extensive, long-lasting, and unpredictable. Antibiotic-induced dysbiosis contributes in the shorter term to antibiotic-associated diarrhea (AAD) and is epidemiologically linked to a variety of longer-term health problems, including obesity, asthma, allergy, and inflammatory bowel disease.
Microbiome of the Neonate
Neonates face enormous challenges at parturition, including developing tolerance to their new microbiota while maintaining immunity against infection. The initial colonization of the gastrointestinal (GI) tract is an intricate balance between the colonization of commensal bacteria that leads to the establishment of tolerance and the prevention of infections secondary to the selective recognition of pathogenic microbes by the host. These host-microbial interactions are critical for the development and function of both the GI tract and the immune system. For example, the microbiota of the GI tract regulates angiogenesis, enterocyte proliferation, and proper crypt formation, along with development and function of gut-associated lymphoid tissue and the intestinal T cell populations that prevent intestinal inflammation. In a healthy neonate, this early crosstalk between commensal bacteria and the host leads to pathogen recognition, epithelial barrier maturation, immune system development, and development of tolerance to food antigens and commensal bacteria.
Microbial exposures early in ontogeny are associated with a range of diseases from atopy and autoimmune disorders to obesity and cancer. This process is thought to occur either through epigenetic epithelial and/or immune system changes or by providing a niche for specific microbial colonization that influences long-term health outcomes. However, exactly how the microbiome is established, and the impact of prenatal and postnatal exposures on the development of the microbiome, is only starting to be elucidated but offers great promise in predicting, preventing, and treating a variety of diseases.
The neonatal GI tract rapidly becomes colonized with microbiota. Newborn’s initial microbiota is acquired by vertical transmission of the maternal microbiome during delivery, although there is evidence for and against low-level microbial colonization of the placenta in utero. The mode of delivery, either via vaginal or caesarian section, influences the acquisition of most of the initial microbes. As such, prenatal factors that affect the maternal microbiome also influence the newborn’s microbiome.
The neonatal microbiome follows a general developmental process although significant interindividual variation is prominent. In the first month, facultative anaerobic microbes from the Enterobacteriaceae family, a large group of gram-negative bacteria that includes pathogens such as Escherichia coli along with nonpathogenic bacteria, dominates the neonatal microbiome. Over the next few months, the Enterobacteriaceae are succeeded by anaerobic bacteria, including the families Bifidobacteriaceae, Bacteroidaceae, Lachnospiraceae, and Ruminococaccea. Around the time of weaning, a varied mixture of bacterial families are present, including Clostridiaceae. One way to monitor the development of the microbiome is to use microbial ecology concepts, such as alpha diversity, that describe the number and distribution of species present in a given individual. For example, alpha diversity is lower in infants than adults, reflecting the higher number of microbial species in the adult microbiome. The microbiome of neonates and infants is rapidly changing but stabilizes into an adult-like microbiome by 3 years of age. A multitude of factors, such as the maternal microbiome, mode of delivery, diet, and antibiotic exposure, influence this process.
Moreover, murine models suggest that, not only is the fetus exposed to the maternal GI microbiome before delivery but that colonization of mice during gestation has direct effects on the development of the offspring’s immune system. These data raise the possibility that prenatal exposure to antibiotics or other means of altering maternal microbiomes can have profound implications for their offspring. In support of this, prenatal exposure to antibiotics has been associated with increased risk for obesity and asthma.
Mode of delivery (cesarean section vs vaginal) also shapes the neonatal microbiome. The microbiome of infants born vaginally is characterized by fecal resident microbes such as Bifidobacterium longum and Bifidobacterium catenulatum , whereas infants delivered by caesarian sections have environmental microbes as their predominant microbiota. This difference is long-lasting and can be seen even in older infants and children. Moreover, studies have suggested a link between the caesarian-section–associated microbiome and long-term outcomes, such as asthma, gastroenteritis, celiac disease, and diabetes.
Infant diet also contributes to the development and composition of the microbiome. The microbiomes of breastmilk-fed and formula-fed infants are quite distinct. Breastmilk is high in prebiotic compounds, such as human milk oligosaccharides, that enhance bacterial growth. It also contains live bacteria not found in formula that can influence the microbiome. The microbiome of neonates fed a breastmilk diet is more abundant in Bifidobacteria and Lactobacillus . Surprisingly, several studies have found that, although breastfed infants have higher bacterial counts, they have a lower species diversity than formula fed infants.
Antibiotic Effects on the Microbiome
Postnatal exposure to antibiotics is another important factor that shapes the microbiome. Antibiotic treatment decreases alpha diversity of the individual’s microbiome. For example, a seminal study in 3 healthy adults showed that 5 days of standard dose (500 mg twice a day) antibiotic treatment with ciprofloxacin had a significant effect on roughly one-third of the bacterial taxa identified in the study. However, most of these disturbances only lasted approximately 4 weeks, although some taxa were still missing at 6 months after treatment. Additional studies have corroborated these finding and demonstrated that some antibiotics have even more profound and long-lasting disruptions of the microbiome. Further, antibiotic exposure in infants and young children may have significant impacts on the microbiota during critical periods of development. For example, the microbiomes of infants exposed to ampicillin and gentamicin perinatally showed a decrease in Actinobacteria, Bifidobacterium and Lactobacillus and an increase in Proteobacteria at 4 weeks of age, and continued to have a decrease in the alpha diversity of these species even by 8 weeks of age. In a longer study that evaluated the microbiota of 43 infants form birth to age 2, Bokulich and colleagues observed that early antibiotic exposure led to a decrease in the microbiome’s alpha diversity and specific deficits in the Clostridium and Ruminococcus species. Moreover, they showed that early antibiotic exposure decreased stability and delayed the maturation of the intestinal microbiome. Similarly, another longitudinal cohort of children from birth to age 3 also showed a decrease in the alpha diversity of the microbiota of children exposed to antibiotics. Additionally, they showed that the species found in the microbiota of children exposed to antibiotics was dominated by a single strain rather than having multiple strains of the same species and, similarly to the previous study, had deficits in clostridium species. Finally, antibiotic-exposed microbiota had an expansion of antibiotics resistance genes ( Fig. 1 ).
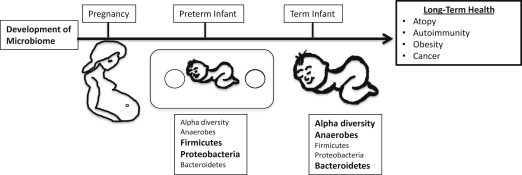
Microbiome of the Preterm Infant
Preterm infants face a more difficult challenge to maintain homeostasis with their developing microbiomes. They have immature immune and GI systems, commonly receive multiple courses of antibiotics, and have abnormal feeding patterns. Not surprisingly, the colonization patterns of the GI tract differ in preterm and term infants. The diversity of the premature infant’s microbiota is even more limited than in full-term neonates, where many of the detectable species in premature infants’ microbiomes are known neonatal pathogens. Additional differences include fewer anaerobes, increased abundance of Firmicutes and Proteobacteria, and decreased abundance of Bacteroides, as well as a substantial delay in bifidobacterial colonization when compared with full-term neonates.
Necrotizing enterocolitis
One of the most devastating emergencies of the premature infant is necrotizing enterocolitis (NEC), affecting up to 10% of all premature infants. Although the pathogenesis of NEC remains incompletely understood, there is growing appreciation that defects in the development of host-microbiome commensalism likely contributes. NEC is characterized by uncontrolled intestinal inflammation that can lead to tissue necrosis, perforation, and sepsis. It is associated with mortality rates as high as 30% and substantial short-term and long-term morbidity. Prematurity is the predominant risk factor for NEC. Interestingly, most cases of NEC occur at 31 to 32 weeks of corrected gestational age, independent of gestational age at birth, suggesting that host intrinsic developmental factors may affect the pathogenesis of NEC.
Microbiome and Necrotizing Enterocolitis
Murine models of NEC have suggested that bacterial colonization of the intestine is essential to the development of NEC; however, no specific bacterial species has been identified as the causative agent for NEC. Human cross-sectional studies have also failed to identify a causative bacterial agent for NEC. Longitudinal analyses of fecal microbiomes from premature infants are beginning to shed some light on the differences in microbiome composition and colonization patterns between infants who develop NEC and those who do not. Several small, longitudinal studies have implicated a variety of bacterial species. For example, Torrazza and colleagues showed that Proteobacteria and Actinobacteria were more abundant in stools of infants who later develop NEC, whereas Bifidobacteria and Bacteroidetes were less abundant in those infants. Fecal dysbiosis was also identified in a study by Morrow and colleagues that similarly showed an increased abundance of microbes from the Proteobacteria and Firmicutes phyla preceding the development of NEC. Two separate small studies associated increased abundance of Clostridia species and Gammaproteobacteria with NEC. In a cohort of 11 infants with NEC and 22 controls, Heida and colleagues showed that the meconium of infants who subsequently developed NEC was enriched for Clostridium perfringens and Bacteroides dorei species when compared with control infants. Moreover, the abundance of staphylococci species was negatively associated with NEC development. A recent large, multicenter study that analyzed stool samples from 166 infants of whom 46 developed NEC did not find any difference between the microbiome of the meconium in infants who developed NEC or controls. However, there were significant differences between groups by 1 month of age and, similarly to previous smaller studies, there was a higher abundance of Gammaproteobacteria before the diagnosis of NEC. Additionally, there was a reduction in strict anaerobes and alpha diversity that preceded the development of NEC in infants born less than 27 weeks of age. Taken together, these studies suggest that there is a clear dysbiosis with shifts toward increases in Gammaproteobacteria that precedes the development of NEC.
Antibiotics and Necrotizing Enterocolitis: Friend or Foe?
Although it remains controversial, prenatal and/or postnatal exposure to antibiotics might contribute to the dysbiosis preceding NEC. Several older randomized controlled trials (RCTs), as well as animal studies, have shown that prophylactic administration of enteral antibiotics can prevent this disease. Five RCTs have been conducted to evaluate the use of prophylactic enteral antibiotics (gentamicin, vancomycin, and kanamycin) for the prevention of NEC, all demonstrating significant reductions in rates of NEC, with a subsequent meta-analysis showing an almost 50% reduction in the rates of NEC and a 70% reduction in NEC-related deaths. However, these studies have limitations. The most recent study was conducted in 1998 (and the others more than 30 years ago) and there was limited adjusting for confounding, accounting for diet and feeding schedules, or reporting of harmful side effects of the drugs. Further, the standard of care in the neonatal intensive care unit (NICU) has changed dramatically since then, with implementation of standardized feeding protocols, earlier introduction of enteral feeds, and use of donor human milk when maternal breast milk is not available, all of which have significantly reduced the rates of NEC. As such, the applicability of these studies to the modern day NICU is uncertain. Additionally, there is concern that prolonged exposure to antibiotics will increase antibiotic resistance. Consistent with this, Boyle and colleagues found that enteral kanamycin treatment was associated with an increase in antibiotic resistant bacteria. Interestingly, enteral vancomycin treatment was not associated with increased antibiotic resistance in this study but was associated with significant changes in the microbiota with predominance of gram-negative bacteria and yeasts, a milieu that could be harmful to the premature host. Thus, before recommending empiric enteral antibiotic treatment of neonates at high risk for NEC, additional data are required to ensure that the potential benefits would outweigh the risks. Yet, these older RCTs, along with the new prospective characterization of the neonatal microbiome before the onset of disease, provide strong evidence for the importance of intestinal microbiota in the pathogenesis of NEC and raise enthusiasm for microbiota modulating therapy.
More recent studies have addressed whether parenteral antibiotics affect the risk for NEC. Premature neonates almost universally receive broad-spectrum antibiotics during their first 2 days of life and many receive more prolonged antibiotic courses for treatment of culture proven or culture-negative sepsis. Various retrospective studies have shown that prolonged antenatal and postnatal antibiotic exposure is associated with an increased risk of developing NEC. Specifically, a retrospective analysis of 97 matched pairs showed that prenatal exposure to ampicillin was significantly greater in infants with NEC. Similarly, empirical (culture negative) antibiotics exposure for greater than 5 days has been associated with the development of NEC in numerous studies.
This discordance in the effects of enteral versus parenteral administration of antibiotics on the effect of NEC suggests that it is not the use of the antibiotics per se that is detrimental but that alterations in the composition of the microbiome can either predispose to, or protect against, the development of NEC. Alternatively, residual confounding by indication might explain this association because patients who receive antibiotics may represent a sicker group with a higher risk for NEC that is independent of antibiotic exposure. Teasing this apart will require further study that explores NEC-associated pathogens such as Gammaproteobacteria in healthy and antibiotic-treated neonates.
Probiotics and Necrotizing Enterocolitis
Because intestinal dysbiosis is associated with the development of NEC, modulating the host’s intestinal microbiome could be a way of preventing or ameliorating the disease. Probiotics have been extensively studied and, in general, seem to reduce the incidence of NEC. A recent meta-analysis of 20 RCTs involving 5982 subjects, showed that the relative risk (RR) of NEC was reduced by almost 50% and overall mortality by 27% with the use of probiotics. However, these studies used various probiotics ( Lactobacillus , Bifidobacterium , or Saccharomyces spp) and dosages. Subgroup analysis identified that Lactobacillus or a mixture of Lactobacillus and Bifidobacterium were most beneficial in reducing NEC. However, probiotics have not been used routinely to prevent NEC in the United States, owing to a lack of a product approved by the Food and Drug Administration (FDA) for this age group and little knowledge of potential adverse outcomes and long-term data. Additionally, a recent phase III trial failed to show a benefit to using Bifidobacterium breve BBG-001 in preventing NEC, suggesting that this Bifidobacterium alone is not the optimal agent to use. Initially there was concern that the use of probiotics in premature infants would increase the risk of sepsis, which was extrapolated from case reports of probiotic-associated sepsis in immunocompromised and short bowel syndrome patients. However, the meta-analysis did not show an increase in sepsis in infants receiving probiotics. Thus, probiotics remain a promising intervention for preventing NEC, though the most effective and safe preparation has not been clearly identified.
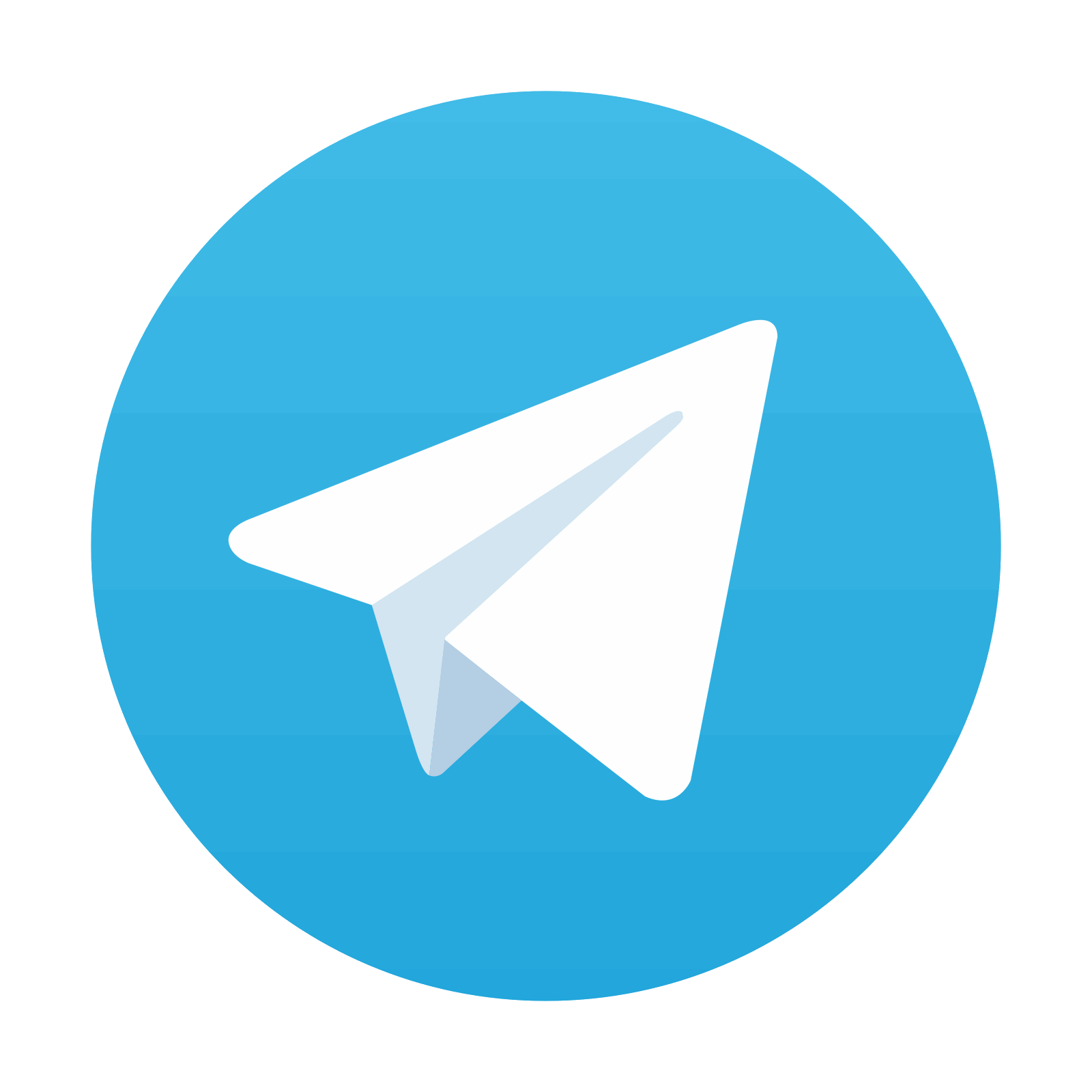
Stay updated, free articles. Join our Telegram channel

Full access? Get Clinical Tree
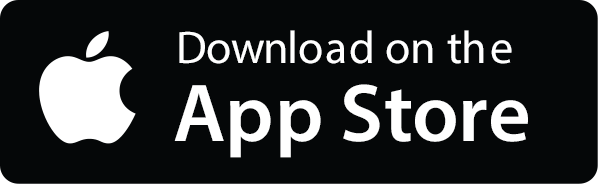
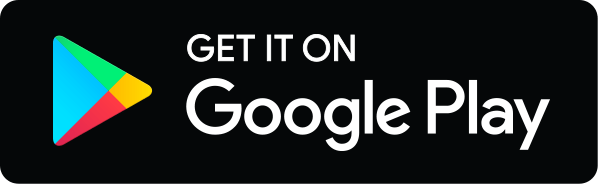