Developmental period
Dose range (mGy)
Teratogenic effects
Any
<50
None
Preimplantation (0–2 weeks after conception)
50–100
All-or-none effect resulting in either embryonic demise or no effect
Organogenesis (2–8 weeks)
200–250
Congenital anomalies and growth restriction
Fetal period: 8–15 weeks
60–310
High risk for neurological deficits (microcephaly, intellectual deficit, or severe intellectual disability) related to accelerated neuronal proliferation and migration during this period
Fetal period: 16–25 weeks
250–280
Lower risk for severe intellectual disability as neurons become progressively neuroresistant
Teratogenic effects from radiation exposures during this period generally manifest with radiation-induced malformations and general growth restriction. The central nervous system is particularly sensitive to ionizing radiation during this period and extending to the 15th week of pregnancy, as neuronal stem cells with high mitotic activity migrate along their pathways from the ventricular zones to the cortical mantle [1, 12]. This probably explains the relative frequency of diminished IQ, mental restriction , and microcephaly found in patients exposed to sufficient fetal doses during susceptible developmental periods. Cells of the central nervous system become progressively more radioresistant beyond the 25th week of gestation, after which there are very little, if any, neurological effects. As these malformations are deterministic effects, the threshold dose necessary to induce these effects ranges from 100 to over 200 mGy [1].
Examinations in which the fetus is positioned directly within the radiation field (e.g., abdominopelvic CT) warrant consideration of the potential risks of fetal exposure (Table 4.2), whereas CT examinations where the fetus is not directly irradiated (i.e., scatter radiation from chest, head, or neck CT) pose less of a risk [1, 14]. Direct measurement of fetal dose is not possible due to variations in beam attenuation related to maternal size (anteroposterior dimension), fetal depth, the scanned region, and CT scanner parameters (filtration, tube voltage [kV, kilovolt], current [mAs, milliampere-second], pitch, rotation time, detector configuration, and number of acquisitions) [4, 14, 15]. Estimates of absorbed fetal dose are derived from phantom models [15, 16]. Based on these and a few clinical models, estimated fetal doses from single-acquisition abdominopelvic CT have ranged from 10 to 15 mGy, well below the threshold dose associated with fetal malformations [14].
Table 4.2
Estimated maternal and fetal exposures to ionizing radiation during abdominal and pelvic imaging
Modality | Fetal dose (mGy) | Maternal dose (mSv) |
---|---|---|
Radiography | ||
Abdominal | 0.1–0.3 | 0.01–1.1 |
Pelvis | 1.1–4 | |
Fluoroscopy | ||
Intravenous pyelography | 5–10 | 0.7–3.7 |
Small bowel follow-through | 1.0–20 | 2.0–18.0 |
Barium enema (double contrast) | 7 | 3.0–7.8 |
CT | ||
Abdomen | 1.3–35 | 3.5–25 |
Pelvis | 10–50 | 3.3–10 |
Abdomen & Pelvis without contrast (renal stone protocol) | 10–11 | 3–10 |
Abdomen & Pelvis with contrast | 13–25 | 3–45 |
Nuclear medicine | ||
99mTc DTPA | 0.35–9 | 1.39–3.79 |
99mTc MAG3 | 0.58–13.5 | 1.32–9.0 |
99mTc MDP | 1.8–7.87 | 1.8–4.7 |
99mTc RBC in vivo | 2.51–5.95 | 2.5–6.0 |
Stochastic effects of in utero exposures (namely carcinogenesis) are probably of higher concern for the patient and clinician, as these are not predictable and may occur at any dose or gestational age, with increasing probability with higher or more frequent exposures [1, 11, 12]. The risk of developing childhood cancer from in utero exposure varies from negligible to low depending on whether the exposure was a one-time, high-dose event or repeated low-dose (≥10 mGy) exposures . Comparing the lifetime risk of development of childhood malignancy (e.g., leukemia) in exposed fetuses to unexposed controls (rate 0.2–0.3%), the relative increase in risk of developing radiation-induced cancer was minimal (rate 0.3–0.7%) [1, 13].
Radiography , Fluoroscopy, Interventional Radiology and Nuclear Medicine
Although radiography remains the most common radiation-producing imaging examination performed in pregnant patients, the total average absorbed fetal dose from this modality is far less than that of CT [6]. If exposure of the fetus to radiation from a fluoroscopic or interventional radiology procedure is necessary, ultrasonography should be used for guidance, when possible, and dose-minimizing techniques employed by the operator, including optimization of patient positioning and shielding, limiting fluoroscopic time, reducing the number of images, utilizing last-image hold technique, lowering the frame rate, applying tight collimation, and limiting use of magnification [5, 17].
In general, most nuclear medicine procedures may be delayed until the postpartum period. However, most achieve radiation exposures much below threshold doses believed to induce fetal harm [5, 13, 18, 19] (Table 4.2) For example, technetium 99 m used for most procedures delivers less than 5 mGy to the fetus. Adequate hydration and bladder catheterization may be useful to expedite the renal excretion of radiotracers, thereby limiting fetal exposures [5].
Ultrasonography
Ultrasonography has long been established as a safe modality for the first-line evaluation of both fetal and maternal wellbeing. It is preferred in the evaluation of pregnant patients as it avoids the use of ionizing radiation, is inexpensive, and easily accessible. However, limitations of ultrasonography relate to the skill of the operator (sonographer) and patient body habitus [20]. Ultrasonography operates on deposition of energy in the form of sound waves though tissues, measuring tissue composition and movement as reflections and attenuation of the sound beam. Mechanical and thermal energy produced by the ultrasound pulse waves may theoretically affect the developing fetus, although no deleterious effects have been documented in humans. Nonetheless, the Food and Drug Administration (FDA) has established limits for mechanical and thermal indices of less than 1 and energy exposure to less than 94 mW/cm2 (milliwatts per square centimeter) [5, 20, 21]. As color, power, and spectral Doppler techniques utilize higher intensity acoustic output, these modalities are generally used only when needed throughout pregnancy and avoided in the early 1st trimester. Fetal heart rate is instead evaluated with lower energy M-mode ultrasonography [5, 21].
Computerized Tomography
In general, CT is to be avoided in pregnancy , as scans of the abdomen and pelvis deliver the most radiation to the fetus. Nevertheless, its use in pregnancy is increasing at a rate more than twice that of other imaging modalities [6, 22]. Despite the continued increase in abdominopelvic CT utilization in pregnancy, the percentage of scans positive for acute pathology has not changed [6]. The benefits of information obtained from a scan must outweigh the inherent risks to the fetus. However, these perceived fetal risks should not deter a clinician from using CT as a diagnostic tool should acquisition of adequate information about potentially life-threatening diagnoses be delayed or suboptimal by other means [1, 2, 12, 23]. CT offers the added benefit of detecting unanticipated masquerading causes of acute abdominal pain [21]. It is generally preferred to exhaust nonionizing imaging modalities (US and MR) before CT or radiography when possible. Uniquely, CT is considered a first-line modality in the evaluation of pregnant trauma patients, following initial rapid triage with focused abdominal sonography for trauma (FAST) scan to evaluate for free intraperitoneal fluid, pleural effusions, and pericardial fluid [3, 5, 21, 23, 24].
Magnetic Resonance Imaging
MRI offers the diagnostic benefits of nonionizing, cross-sectional, high soft tissue resolution evaluation of abdominopelvic pathology. MR imaging at ≤3 tesla strength is possible and safe at all stages of development [1, 2, 5]. To date, no studies have demonstrated harmful effects to the fetus from MR. However, theoretical risks to the fetus exist related to inherent properties of the magnet. These include the static magnetic field strength, applied gradient magnetic fields, and radio frequency (RF) pulses. High magnetic field strengths are hypothesized to affect fetal neuronal migration and proliferation during early pregnancy but remain theoretical [5].
Energy deposition in tissues from the RF pulses may lead to tissue heating. This is known as the specific absorption rate (SAR) and is quantified as watts per kilogram (W/kg), with federally mandated and internally monitored upper limit of 4 W/kg. This limit is associated with a 0.6 °C increase in maternal body temperatures after 20–30 min of imaging [5]. Fetal injury may occur with maternal temperature elevations of 2–2.5 °C for 30–60 min, but the maximum SAR is deposited in the maternal surface tissues with fetal heating considerably less than the proposed detrimental levels [5, 25].
MR sequences that employ rapid gradient switching (particularly in single-shot fast spin-echo [SSFP] sequences commonly utilized in fetal imaging) lead to higher energy deposition, as well as acoustic noise in the scanner. Use of such sequences should be kept brief, as theoretical damage to the developing fetal ear may result exposure to ≥90 dB (decibel) [5].
Utilization of Intravenous Contrast in Pregnancy
In addition to the risks of ionizing radiation, intravenous contrast material utilized in diagnostic imaging poses additional risks to fetal wellbeing. Both iodine- and gadolinium-based contrast agents are known to cross the blood–placenta barrier and enter the fetoamniotic circulation [26].
Iodinated Contrast
To date, no adverse fetal or neonatal effects have been documented from administration of low-osmolality iodinated contrast media (e.g., iodinated contrast used in CT) and are thus classified by the United States FDA as Category B (no adverse effects in animal studies but controlled studies in pregnant patients do not exist) [5]. The American College of Radiologists (ACR) , therefore, recommends against withholding iodinated contrast agents in pregnant patients if it is necessary for diagnosis [26]. Concerns over fetal and neonatal hypothyroidism after in utero exposure to iodinated contrast have not been validated , and neonates are generally screened for hypothyroidism at birth if there is a pertinent history [5, 26].
Gadolinium-Based Contrast
Gadolinium-based contrast agents have been labeled as Category C medications by the FDA because of documented fetal demise and malformations associated with in utero exposure in animal studies, yet these effects have not been documented in retrospective human studies [5]. Therefore, these agents should be administered with caution in pregnant patients, and their use is generally avoided [2, 26]. The ACR does indicate that use of gadolinium-based contrast in pregnancy should occur only after carefully documented consensus decision by the radiologist, clinician, and patient, when the information obtained from its use outweighs the purported risks to the fetus from exposure to free gadolinium ions [2, 5, 26, 27]. In such cases, agents with tightly bound gadolinium-chelate complexes are advised, administered at the lowest dose, including gadobenate dimeglumine (MultiHance; Bracco Diagnostics, Monroe Township, NJ) or gadoteridol (ProHance; Bracco Diagnostics) [5]. Informed consent should be obtained from the patient and documented in the medical record prior to gadolinium administration.
Screening for Pregnancy
In allowable clinical settings, all women of potential childbearing age (defined as ages 12 through 50 years) should undergo a full determination of pregnancy status to include menstrual and surgical history (tubal ligation or hysterectomy), contraceptive use, and qualitative urine human chorionic gonadotropin (hCG) or quantitative serum human chorionic gonadotropin (β-hCG) testing, prior to the use of direct ionizing radiation [1, 2, 21]. In some patients, pelvic US may be indicated to assess the status of the pregnancy. Pregnancy status can be assessed by interview of the patient as to her likelihood of pregnancy (e.g., not sexually active, on effective birth control, or biologically incapable of pregnancy) or that she has recently completed her menstrual period within the last 4 weeks prior to imaging [1]. Pregnancy test results should not be the sole screening measure, and patients must undergo the standard interview procedure, as negative pregnancy tests do not necessarily exclude recent conception [5]. A positive pregnancy test will prompt the imaging technologist to follow appropriate pregnancy-tailored protocols. For procedures expected to deliver high doses of radiation to the uterus (e.g., pelvic interventional procedures), a pregnancy test should be obtained within 72 h proceeding [1]. The ACR and ACOG both emphasize that when pregnancy status cannot be verified in the emergent setting, a waiver should be included in the patient’s medical record documenting the critical need for the study [1, 2].
Counseling the Pregnant Patient About Imaging Procedures
Patients may report anxiety surrounding the exposure of their fetuses to ionizing radiation and must be appropriately counseled. It should be emphasized in a positive light that for most imaging modalities, the risk of pregnancy loss, birth defects, mental restriction, and subsequent childhood malignancy are small [1, 21]. The risk of incurring detrimental effects from exposure to ionizing radiation of imaging examinations must be considered in light of generalized background risks associated with pregnancy, such as birth defects (3%), miscarriage (15%), prematurity (4%), growth restriction (4%), and mental restriction or neurological deficits (1%) [1, 2, 20, 22]. At the relatively low fetal doses encountered in abdomen and pelvic imaging (10–35 mGy for abdominopelvic CT), threshold limits are extremely unlikely to be met to cause deterministic effects . The main concerns therefore are those of carcinogenesis in later life, which, for examinations involving less than 50 mGy fetal exposures, are less than 0.8% with attributable lifetime risk of approximately 2% [1, 2, 5]. More clearly stated, there is a 98–99% likelihood that the fetus will be unaffected by the radiation. Useful comparisons with background radiation may highlight that differences in topographic elevation between women in Denver, Colorado, and those living at sea level result in a slight relative excess exposure to radiation levels (about 0.6 mSv, millisievert), which only theoretically place fetuses at risk for an additional attributable cancer in every 5000 births [1]. Average background radiation is estimated at 1.0–2.5 mGy. There exists no single, wellmonitored diagnostic radiology procedure which will result in a radiation dose significant enough to affect the wellbeing of the fetus. Therefore, pregnancy termination is not justified for fetal exposures to a single examination (< 100 mGy) [1, 2, 5, 11].
Nongynecological Causes of Abdominal Pain
Appendicitis
Acute appendicitis is the leading cause of acute abdominal pain in pregnancy requiring surgical intervention and complicates 1 in 2000 pregnancies [3, 21, 28, 29]. First-line imaging of the pregnant patient with right lower quadrant pain is ultrasound with graded compression. However, despite being the first-line modality for the evaluation of appendicitis, ultrasonography has a low yield, allowing visualization of the appendix only 1/3 of the time. Another study showed that the sensitivity and specificity of US in the assessment of appendicitis is only up to 78% and 83%, respectively [20]. Knowledge of variation in appendiceal location in the gravid abdomen may increase diagnostic yield. Detection of a blind-ending, tubular, nonperistaltic, noncompressible structure arising from the cecal base, with luminal diameter > 6 mm establishes the imaging diagnosis (Fig. 4.1). Use of color Doppler may reveal associated hyperemia and surrounding inflammation of the periappendiceal fat [20, 21, 28, 30]. In the setting of acute appendicitis, the patient is often exquisitely tender with direct transducer pressure over the appendix, which can help confirm the diagnosis. When these findings are variably present, equivocal, or when the appendix is not discretely visualized, imaging with other modalities is typically warranted.


Fig. 4.1
US of acute appendicitis: acute appendicitis in a 31 y/o pregnant patient at 21 weeks’ with right lower quadrant abdominal pain . (a) Gray scale image of the right lower quadrant demonstrates a longitudinal view of a dilated, blind ending tubular structure arising from the cecum consistent with the appendix (arrows). Echogenic, shadowing appendicolith (arrowhead) is seen within the lumen toward the base. Gray scale transverse images through the appendix without (b) and with compression (c) demonstrate an appendiceal diameter of 10 mm with no change with compression. Appendicitis was confirmed at surgery
Delay in diagnosis and surgical management may lead to perforation, which is associated with up to 30% fetal loss, and occurs most frequently in later stages of pregnancy when sonographic evaluation of the appendix is technically difficult from progressive displacement of the appendix by the gravid uterus [28]. MR is a highly sensitive (100%) and specific (94%) modality for the diagnosis of appendicitis in pregnancy after targeted ultrasound examinations fail to demonstrate a normal appendix [25, 27, 31, 32]. The extremely high negative predictive value of MR (100%) is helpful in triaging patients who may potentially have a surgical abdomen [31]. As with the other modalities, the variable position of the appendix may complicate interpretation. A normal appendix is defined as one with a luminal diameter of ≤6 mm, or an air- or contrast-filled lumen (using either rectal saline or oral ferumoxil oral suspension admixed with barium sulfate) [31]. A positive exam demonstrates an enlarged, thickened appendix (>7 mm) with fluid-filled lumen with or without periappendiceal inflammation, most apparent on T2-weighted images as ill-defined increased signal in the periappendiceal fat (Fig. 4.2). Indeterminate results include luminal size between 6 and 7 mm and no surrounding inflammation [27, 31]. MR also offers the added benefit of detecting potential alternate diagnoses for the patient’s complaints [32].


Fig. 4.2
MRI of acute appendicitis: acute appendicitis in a 29 y/o pregnant patient at 31 weeks’ with right lower quadrant abdominal pain. Axial (a) and coronal (b) T2 HASTE images demonstrate a dilated, thick walled, fluid-filled appendix (arrows) measuring 9 mm in diameter with periappendiceal inflammation. Appendicitis was confirmed at surgery
If ultrasonography and MRI are inconclusive or in the absence of available MRI, abdominopelvic CT with contrast may be used to evaluate for appendicitis [3]. However, dose reduction techniques should be considered [8]. Lazarus and colleagues reported CT to have a 92% sensitivity and 99% specificity for the diagnosis of appendicitis, with overall negative predictive value of 94% [29]. Furthermore, CT can detect additional pertinent diagnostic information, such as small bowel obstruction and urinary calculi, among others [29].
Urolithiasis and Urinary Tract
Urolithiasis and urinary tract infections constitute the most common painful nonobstetric causes of abdominal or pelvic pain in pregnancy [21]. Urolithiasis affects as many as 1 in every 1500 pregnancies, and coexisting pyelonephritis or urinary tract infection frequently leads to hospitalization. Ultrasound is the first-line modality for evaluation of suspected renal colic in pregnancy [3, 5, 21, 22, 28, 33]. Renal sonography readily detects the presence of hydronephrosis, but a distinction must be determined between physiological hydronephrosis of pregnancy (more commonly right sided and a result of mechanical compression of the gravid uterus and hormone-related ureteral relaxation) and obstructive urolithiasis (more frequent in pregnancy due to increased urine production and stasis) [21, 28, 33]. Renal pelvis diameter measuring up to 25 mm on the right and 10 mm on the left up to 40 weeks of gestation may be physiological [34]. Smooth tapering of the distal ureter as it enters the pelvis and the absence of luminal filling defects help confirm the diagnosis. Ultrasonography is highly sensitive and specific (90% and 98%, respectively) for hydronephrosis, increased in the presence of a stone [33].
Ultrasonographic sensitivity for detection of stones is highly variable, ranging from 34–95% [35, 36]. Renal or ureteral stones manifest as foci of hyperechogenicity often with accompanying acoustic shadowing (Fig. 4.3). When color Doppler is applied, a “twinkling artifact” may result (up to 86% of urinary calculi), increasing diagnostic confidence [30, 34]. When possible, imaging should be performed with a distended bladder to better evaluate distal ureters. Transvaginal (TV) ultrasound can be performed to evaluate the presence of distal ureteral stones, distal ureteral dilatation, and ureteral jets in patients with inconclusive transabdominal examinations. In late pregnancy, ureteral jets may not be visualized, and their absence does not necessarily indicate obstructive urolithiasis. Reimaging the patient in the contralateral decubitus position may elicit a ureteral jet or confirm its absence [33]. Despite conflicting evidence, differences in renal vascular resistive indices (RI) between each kidney can help to differentiate these entities, with no difference in RI between the kidneys in physiological hydronephrosis whereas RI greater than 0.70 suggests obstructive pathology, particularly if unilateral [33]. The rate of spontaneous passage of urinary stones in pregnant women reportedly ranges from 48% to 81% and persistent obstructive stones although conservative management may undergo further treatment with nephrostomy tube placement or ureteral stent placement [33]. Nephrostomy tube placement may be accomplished with ultrasound guidance to further limit exposure of the patient and fetus to fluoroscopy [17].


Fig. 4.3
US of obstructive uropathy : 35 y/o pregnant patient at 33 weeks’ with right flank pain. (a) Longitudinal gray scale image of the right kidney (arrows) demonstrates moderate right hydronephrosis (asterisk). (b) Gray scale image at the level of the distal right ureter and bladder (asterisk) shows an echogenic shadowing calculus (arrow) in the distal right ureter at the ureterovesicular junction. This initially was not seen when the patient had a decompressed bladder. After hydration and reimaging, the calculus was able to be visualized. The patient was admitted and passed the stone the following day
Low-dose noncontrast CT is a sensitive and specific test for detection of obstructive uropathy in the pregnant patient and should be considered if ultrasound is inconclusive [36, 37]. The likelihood of spontaneous stone passage is dependent on stone width, with decreasing likelihood of passage above 5 mm and a more proximal ureteral location [38]. Stone size can be readily measured by CT and is less reliable on MRI. Retained or impacted stones place the patient at risk for pyelonephritis and pyonephrosis, which can lead to untoward pregnancy complications. Thus, low-dose, noncontrast CT should be used in the setting of suspected obstructive uropathy, even in pregnant patients [37].
MR and MR urography (MRU) utilizing heavily T2-weighted images may serve as helpful adjunct modalities in evaluation of suspected urolithiasis, especially when coexistent physiological hydronephrosis and hydroureter confound US findings in the third trimester [20, 21, 25, 35, 39] (Fig. 4.4). Although, when compared to low-dose CT, MRU suffers from poor spatial resolution and lower sensitivity for detecting calcified stones, with an overall sensitivity of 80% [20, 39]. Flow-related artifacts may mimic calculi, resulting in false positive examinations [21]. Findings of obstructive uropathy on MR include renal enlargement, increased signal (edema) in the renal parenchyma on T2-weighted images, and perirenal fluid [25, 35, 39]. Calculi appear as focal filling defects within the ureteral lumen, usually at a point of abrupt caliber change . MRU may identify distal ureteral calculi and manifest the “double-kink sign” – a column of urine in the distal ureter in association with ureteral constriction at the pelvic brim and vesicoureteral junction [35].


Fig. 4.4
MRI of obstructive uropathy: 28 y/o pregnant patient at 29 weeks’ with right flank pain and hematuria. Renal US demonstrated right hydronephrosis but no obvious ureteral calculus (not shown). (a) Axial T2-weighted MR image of the abdomen demonstrates mild right hydronephrosis, perinephric-free fluid (arrowheads), and a 4 mm hypointense filling defect in the proximal ureter compatible with ureteropelvic junction calculus (arrow). Partial visualization of IUP anteriorly (asterisk). (b) Coronal T2 FIESTA fat-saturated image through the abdomen demonstrates mild right-sided hydronephrosis (asterisk), perinephric-free fluid (arrowhead), and filling defect in the proximal ureter compatible with ureteral calculus (arrow). Right nephroureteral stent was placed after stone extraction
Biliary Disease
Hormones of pregnancy lead to hypersecretion of biliary cholesterol and gallbladder stasis, predisposing a patient to cholelithiasis and subsequent risk for acute cholecystitis , the second-most common surgical abdominal emergency affecting pregnant women [25, 28]. Ultrasonography is routinely used as a first-line evaluation of acute right upper quadrant pain and suspected hepatobiliary pathology. The presence of shadowing gallstones (95% sensitivity), gallbladder wall thickening (>3 mm), distention (>5 cm transverse diameter), pericholecystic fluid, and the presence of a sonographic Murphy sign may be used to diagnoses acute cholecystitis [28] (Fig. 4.5). Dilation of the biliary tree may suggest the presence of choledocholithiasis. However, direct detection of common bile duct stones may be possible in as few as 20% of cases, as bowel gas may obscure the distal duct and 10% of stones may not produce characteristic shadowing [20].


Fig. 4.5
US of acute cholecystitis : acute cholecystitis in a 26 y/o pregnant patient at 19 weeks’ with right upper quadrant pain. Longitudinal (a) and transverse (b) images through the gallbladder demonstrating a distended gallbladder with echogenic intraluminal sludge (asterisks), small layering shadowing echogenic gallstones (arrows) and gallbladder wall thickening (5 mm). Gangrenous cholecystitis was found at time of surgery and confirmed at pathology
Findings of acute cholecystitis on MRI reflect those of US, with gallbladder wall thickening and edema (increased signal on T2-weighted images), pericholecystic fluid, and low signal filling defects within the lumen of the gallbladder reflecting gallstones [25, 40]. In addition, choledocholithiasis is well evaluated by MR cholangiopancreatography (MRCP) [40]. The positive predictive value of MR is 100% for diagnosis of biliary obstruction. Thin-section (1 mm) overlapping MRCP images depict small-rounded hypointense filling defects in T2-hyperintense lumen of the biliary tree, indicating choledocholiths (Fig. 4.6). Common bile duct dilation is present when the distance between the outer walls of the common bile duct measures greater than 7 mm [41].


Fig. 4.6
MRI of choledocholithiasis: Choledocholithaisis in a 19 y/o pregnant patient at 25 weeks’ with epigastric pain and elevated bilirubin. (a) Coronal T2 HASTE image demonstrates a dilated common bile duct (arrow), which measured up to 14 mm, and intrauterine pregnancy (asterisk). (b) Coronal oblique T2 HASTE fat-saturated image from MRCP demonstrates an intraductal-rounded T2 hypointense filling defect in the distal common bile duct measuring 4 mm compatible with choledocholith (arrow). Patient subsequently underwent ERCP with stone removal and stent placement
Although US and MRI are the preferred modalities for evaluation of the biliary system, CT with dose reduction techniques may be utilized when there are contraindications to MRI or when other means for rapid diagnosis are not available [40]. Despite being slightly less sensitive than MR for detecting small noncalcified stones , CT has similar performance to even noncontrast MR in detecting the presence of biliary ductal dilation and choledocholithiasis [42].
Pancreatitis
Pancreatitis is a rare cause of abdominal pain in pregnancy, occurring most frequently in the third trimester and usually as a result of gallstones [43]. Pancreatitis may affect 1 in every 3300 pregnancies [20, 28]. Ultrasonography is limited in the evaluation of the pancreas in the acute setting, as inflammation contributes to generalized ileus, causing bowel loops to interdigitate between the transducer and the pancreatic parenchyma, obscuring its evaluation though bowel gas shadowing [43]. However, US is frequently used as first-line imaging to evaluate for the presence of gallstones. When seen by US, the pancreas may appear enlarged and hypoechoic (edematous), with or without peripancreatic fluid, which may become organized into a collection [34].
As in nonpregnant patients, contrast-enhanced CT depicts changes of pancreatic parenchymal edema, peripancreatic inflammation and fluid, and associated complications such as pancreatic necrosis (parenchymal nonenhancement), peripancreatic fluid collections, ductal dilation, or vascular complications (pseudoaneurysm, venous thrombosis, or hemorrhage) [44]. In general, CT evaluation of pancreatitis requires multiphasic technique, which would subject the patient and fetus to high doses of radiation. Therefore, noncontrast MR is typically utilized in the evaluation of acute pancreatitis in pregnancy, discussed subsequently.
MR findings of acute pancreatitis include parenchymal edema and peripancreatic fluid with or without pancreatic ductal dilation . Complicated pancreatitis may result in peripancreatic fluid collections, which manifest as variable signal on T2-weighted images. As gadolinium-based contrast is typically contraindicated in pregnancy, evaluation for pancreatic necrosis or vascular complications may be limited but can manifest as fluid signal areas surrounding the pancreas on T2-weighted images in the former and lack of flow voids in the setting of splenic vein thrombosis [44]. Diffusion-weighted sequences: diffusion-weighted imaging (DWI) and apparent diffusion coefficient (ADC) , are highly sensitive for early pancreatic inflammation. Furthermore, the presence of hemorrhage may be suggested as heterogenous areas of T1-hyperintense signal within the pancreatic bed [34]. MRCP may be helpful in evaluation of the pancreatic and biliary ductal system, identifying obstructive lesions or variant anatomy [44].
Hepatic Disease
HELLP syndrome (hemolysis, elevated liver enzymes, and low platelets) occurs in up to 12% of pregnant patients with severe hypertension . Findings on ultrasound may be the first signs of the disease, occurring even before biochemical changes, such as hepatic edema (hypertrophy, periportal hypoechoic “halos,” a “starry sky” appearance of the liver with echogenic portal triads admixed among the edematous parenchyma, capsular thickening, or scattered areas of increased echogenicity) [44]. The syndrome may result in hepatic hemorrhage, rupture, or infarction. US diagnosis of hemorrhage can be made by visualization of a heterogeneously echogenic, nonvascular, complex cystic mass with or without lacy internal echoes representing fibrin strands. Serial US examinations should document progressive involution of the hematoma and exclude an underlying mass. Both adenomas and hemangiomas can enlarge during pregnancy, with increased risk of hemorrhage [44]. Although these findings are nonspecific, other causes of hepatic hematoma must be excluded, such as hemorrhagic adenoma, trauma, or intrahepatic vascular abnormalities [20]. Hepatic infarcts manifest as peripheral, geographic, or wedge- shaped hypoechoic areas with diminished vascularity [40].
Pregnancy induces a hypercoagulable state, which predisposes patients to thrombosis [20]. Budd-Chiari syndrome , manifesting clinically as portal hypertension and liver failure, may result from thrombosis of hepatic venules, veins, or the inferior vena cava. Depending on the age of thrombus, venous thrombus may appear hypo- or hyperechoic, with associated expansion of the affected vessel and lack of color signal on color Doppler imaging [45]. A thin fibrotic echogenic cord may be seen within a retracted vessel lumen with chronic occlusion, or as treatment resolves the clot. Color Doppler may also depict extrahepatic or intrahepatic collateral vessels, an indirect, though specific sign of Budd-Chiari syndrome. Hepatic congestion leads to portal hypertension, with dampened or reversal of flow within the portal veins with color and spectral Doppler. A secondary sign of portal hypertension is ascites, visualized on US as simple peritoneal free fluid [45].
Magnetic resonance findings in HELLP syndrome reflect those of sonography. The areas of increased echogenicity on US may demonstrate increased signal on T2-weighted images, indicating edema and early hepatic necrosis [25, 40]. MR appearance of hepatic hematomas varies on the acuity of the blood products but will appear heterogeneous on T1 and T2-weighted images, causing mass effect, with or without capsular rupture, manifest as focal irregularity of the capsule and adjacent sentinel clot [40].
Acute fatty liver of pregnancy (AFLP) most often manifests in the third trimester of nulliparous women with a diffusely hyperechoic hepatic parenchyma on US, a nonspecific qualitative finding [20, 40]. Acute fatty liver of pregnancy on MR appears as hepatomegaly with diffuse increased signal on T1-weighted in-phase images with signal dropout on opposed phase images (representing intracellular triglycerides), or signal dropout on fat-saturated images (revealing the presence of macroscopic hepatic fat) [25].
Budd-Chiari syndrome may manifest on MR as morphological changes in liver contour with subacute and chronic stages (particularly caudate lobe hypertrophy) but diffuse hepatomegaly with smooth contours in the acute stage [45]. Evaluation of hepatic venous patency may be suboptimal without contrast. However, a filling defect within the hepatic vein or inferior vena cava flow voids may suggest the presence of a thrombus. On contrast-enhanced examinations, variations in hepatic perfusion are a prominent feature in Budd-Chiari syndrome, a manifestation of intrahepatic and extrahepatic collateralization. MR may reveal the presence of multiple 1–4 cm regenerative nodules with iso- to hyperintense signal on T1 sequences and iso- to hypointense signal on T2-weighted images, with arterial enhancement and retention of contrast on the portal venous phase [40, 45]. However, given the contraindication of gadolinium in pregnancy, the imager may have to rely on noncontrast features.
Gastrointestinal Disease
Bowel obstruction is another leading cause of abdominal pain, during pregnancy, most often occurring in the second or third trimester [28]. Intestinal obstruction represents the third leading cause for surgical intervention for acute abdominal pain in pregnancy [44]. Most commonly, the obstruction is due to adhesions, which generally cannot be visualized on imaging. Following initial examination with abdominal radiography, CT is typically the next modality utilized in the evaluation of suspected intestinal obstruction in the nonpregnant patient. However, this algorithm is often altered in pregnancy to avoid the use of ionizing radiation by utilizing US or MRI.
The use of sonography has been advocated as a first-line modality for the evaluation of suspected small bowel obstruction in pregnant patients, both because of the lack of ionizing radiation, and targeted evaluation of the bowel, when a gravid uterus in the third trimester may displace the bowel, making typical patterns of bowel obstruction on radiography less reliable [34, 44]. US findings include dilated (>3 cm), hyperperistaltic bowel loops with swirling of intraluminal contents involving long segments (>10 cm) of bowel [46]. Identification of these findings should prompt further evaluation with MRI or CT to identify the transition point and associated complications of obstruction [34].
Findings of small bowel obstruction on CT and MRI include the presence of dilated fluid-filled bowel loops measuring >2.5–3.0 cm in diameter, intraluminal air-fluid levels, “fecalization ” of bowel contents from delayed small bowel transit, mesenteric fluid and haziness, and decompressed bowel distal to the site of obstruction indicated by “beaking” of the stenosed bowel segment [25, 34, 47, 48]. Closed-loop obstructions manifest with clustered small bowel loops tethered in one quadrant of the abdomen and twisting of mesenteric vessels. Bowel wall thickening, mesenteric edema, and hypoperfusion of bowel segments (seen on contrast-enhanced CT as hypoenhancing bowel walls) suggest strangulation [47, 48].
Other etiologies of intestinal obstruction in pregnancy may be secondary to underlying inflammation or fibrosis from active or chronic changes of inflammatory bowel disease (IBD), particularly Crohn’s disease or less likely ulcerative colitis [44]. Although fluoroscopic examinations (namely small bowel follow through) have been traditionally used for evaluation of the small bowel, cross-sectional imaging with CT or MRI is typically preferred as it offers evaluation of extramural disease extent [44]. CT (and particularly CT enterography) findings include mural thickening and enhancement, luminal narrowing, mesenteric or mural inflammation, and fibrofatty proliferation of chronically affected segments [20]. Other complications of IBD are readily depicted on CT and include abscess, fistula, and sinus tract formation. Typically, however, pregnant patients with IBD are imaged with MRI [34].
MR and MR enterography findings mirror those of CT, with increased sensitivity of depicting early bowel wall inflammation in the setting of IBD as increased signal on T2-weighted images. Sensitivity and specificity of MR for detecting these features may be as high as 98% and 100%, respectively [20].
Vascular
Various vascular complications of pregnancy may occur. Pregnant patients are predisposed to ovarian venous thrombusthat is believed to be secondary to the relative hypercoagulable state of pregnancy and venous stasis from compression of the gonadal vein at the pelvic brim by the gravid uterus. As a rare complication of pregnancy, affecting nearly 0.2% of pregnancies, mortality may exceed 60%. [34]. The retroperitoneal location of the ovarian vein often precludes complete visualization by US. The reported sensitivity of US for depicting ovarian venous thrombosis is just over 50% [34, 49].
MR venography (with time-of-flight sequencing) may offer the highest sensitivity and specificity (100%) for diagnosis of ovarian venous thrombosis, manifesting as an area of signal void within the vein. T1- and T2-weighted sequences may reveal intermediate to high signal of the intraluminal clot, depending on the acuity [25, 34, 49]. Therefore, noncontrast MR venography is recommended as the next-line evaluation for patients with inconclusive findings at US [49].
Contrast-enhanced CT with adequate bolus timing and opacification of the gonadal veins may depict the low-attenuation intraluminal filling defects within dilated retroperitoneal tubular structures, indicating thrombus [49].
Splenic artery aneurysms affect approximately 10% of the general population, exhibit a distinct association with pregnancy, and may be complicated by rupture in up to 25% of cases [34, 50]. Several risk factors exist for splenic artery aneurysms, including pregnancy itself. Splenic artery aneurysms may be diagnosed using gray scale and spectral Doppler US as hypo- or anechoic dilations of the native artery near the splenic hilum and a weak, turbulent pulsatile flow pattern. In the presence of mural thrombus or calcification, no flow may be seen.
CT may show a peripherally calcified mass in association with the splenic vessels, most frequently near the splenic hilum. Most measure less than 2.5 cm in size [34]. Aneurysm rupture may be noted as dense fluid in the region of the spleen. MR of splenic aneurysms may show a rounded dark flow void next to the splenic hilum in the presence of flow. Otherwise, thrombosed or calcified aneurysms would appear as iso- to hyperintense masses on T1- or T2-weighted images, near the native vessel [34].
Physiological adaptations to pregnancy include increased circulating blood volume and hormonal changes of the aortic wall, which may predispose to aortic dissection, particularly in the third trimester [51]. Underlying connective tissue disorders, such as in the Marfan syndrome, contribute to inherent weakness of the aortic media , making these patients particularly susceptible to aortic dissection with a fivefold increased risk (4.4%) [52]. Older patient age (>32 years) and ascending aortic lumen diameter > 4.2 cm are both associated with increased risk of dissection and rupture [52, 53]. Contrast-enhanced CT angiography is first employed in the setting of suspected acute aortic dissection, as it is rapid and the extent of the dissection and associated complications can be readily defined. CT findings include the presence of a displaced intimomedial flap which divides the true and false lumens [54]. Complications of branch vessel occlusion, thrombosis, or vessel rupture may also be detected. While not typically used in the acute setting, MRI may provide not only structural information of abdominal aortic aneurysms, but also dynamic and flow-related information [55].
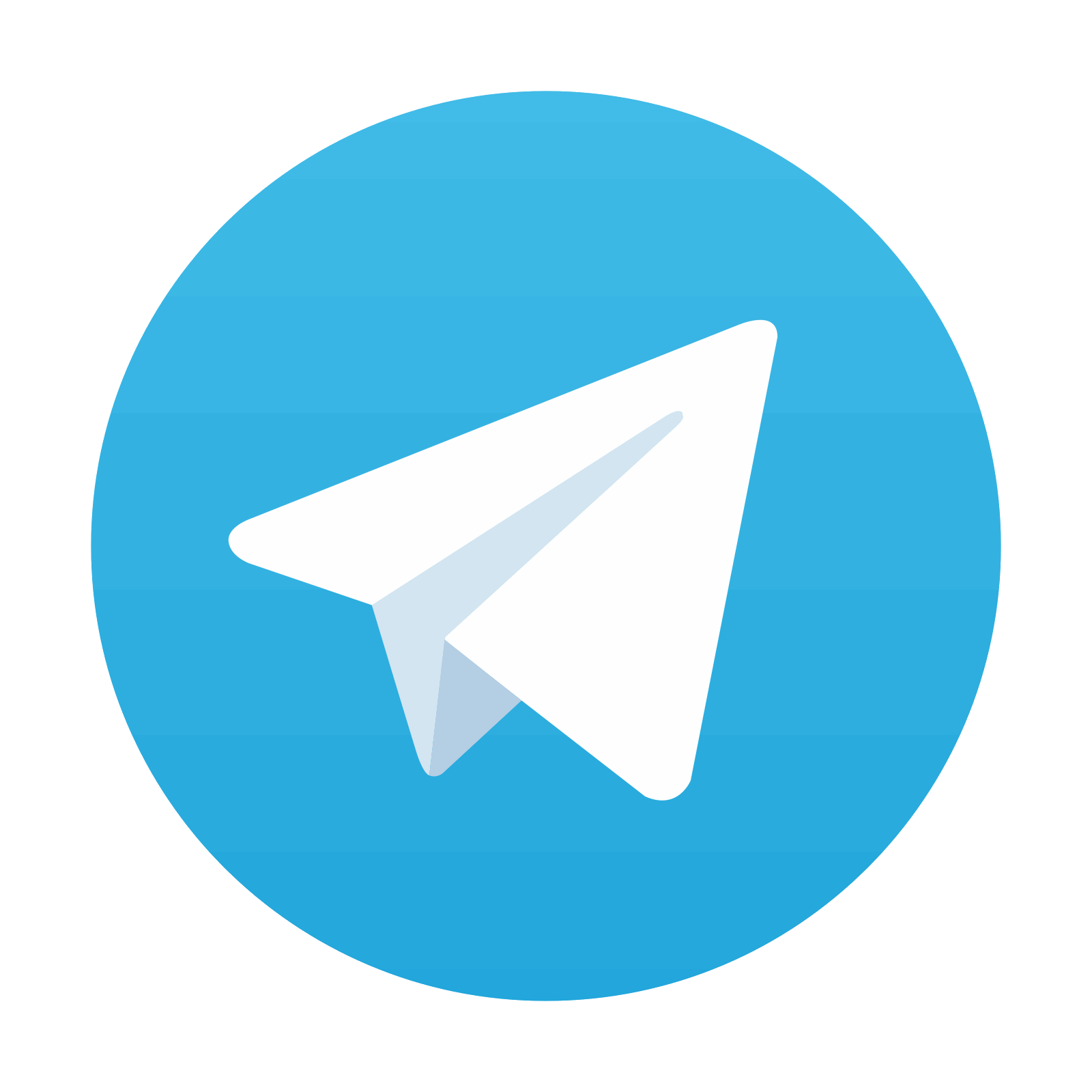
Stay updated, free articles. Join our Telegram channel

Full access? Get Clinical Tree
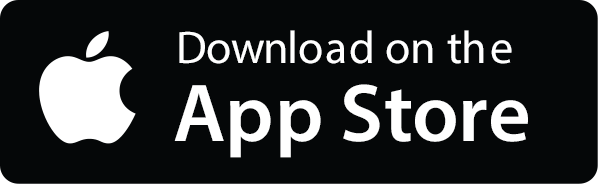
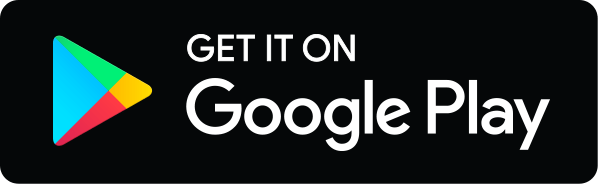