Fig. 4.1
CT-scan of a 38-year-old female patient with synchronous hepatic metastases from a rectosigmoid carcinoma before (left) and after (right) FOLFIRI/cetuximab chemotherapy showing a partial radiologic response
Taking into account intermetastatic and intrametastatic genetic differences between and within lesions in a single patient [30], the heterogeneity in response to chemotherapy has been shown to be a significant prognostic factor that could be assessed radiologically and, thus, preoperatively [31]. For each lesion individually, the increase or decrease in diameter was measured in terms of percentages, and the maximum difference in response taken as a continuous variable. Based on the differences between the best and the least responding lesion, the response was classified as heterogeneous or mixed response (MR) with >30% difference, but all lesions showing similar behavior, true mixed response (TMR) with at least two lesions behaving differently (at least ±10%), or homogeneous response (HR). This being applied to patient groups according to RECIST, partial responders with a heterogeneous response pattern had a significantly poorer survival compared to patients with a homogeneous partial response [31].
Preoperative Radiological Assessment of Pathologic Response
A complete pathologic response (CPR) after preoperative chemotherapy was observed, in a cohort of 767 patients treated between 1985 and 2006, in 4% of cases [32]. In this study, a low-risk subset of patients younger than 60 years with metastases smaller than 3 cm and low CEA levels experienced CPR in 31% of cases, and high 10-year overall and disease-free survival rates of 68% and 69% respectively. None of the patients with CPR had evidence of complete radiologic response. Instead, only two out of 767 patients experienced a complete radiologic response but were found to have small remnant lesions intraoperatively [32]. Although more recent studies indicate a better diagnostic performance of gadoxetic-enhanced MR and diffusion weighted imaging in identifying patients with a complete pathologic response [33], the correlation of preoperative radiologic and postoperative pathologic response is far from being firm at this point, so patients with a radiologically assumed complete pathologic response should still be recommended resection of their hepatic lesions.
Complete Radiologic Response or the Disappeared Liver Metastasis
With the increasing use of highly efficient chemotherapeutic and biological agents in the treatment of colorectal liver metastases, a growing number of patients are experiencing a complete radiologic response—a phenomenon also called vanishing or disappearing liver metastases [34]. As mentioned earlier, reports differ to what extent lesions exhibiting a complete radiologic response were postoperatively found to have a CPR. While some authors have demonstrated only little correlation between response to chemotherapy on imaging and pathological examination [35, 36], others found a CPR in up to two thirds of disappeared metastases [37, 38].
Disappeared metastases can become a surgical challenge when neither intraoperative ultrasound (IOUS) nor digital palpation are able to localize the lesion. The latter aspect is a particular concern for deeply located metastases as well as during laparoscopic liver resections. Strategies to cope with disappeared metastases are [1] early restaging during preoperative chemotherapy after a few cycles and prompt resection before complete disappearance [34], marking of lesions with coils and resection after completion of preoperative chemotherapy [39], or pre-therapy imaging-based computer-assisted 3D-navigated open or laparoscopic resection [40] (Fig. 4.2a–c).


Fig. 4.2
A synchronous colorectal liver metastasis a, having completely disappeared after chemotherapy b. c 3D-fusion imaging
Functional Resectability
Assessment of the Functional Liver Remnant
The major cause of morbidity and mortality of a liver resection procedure is postoperative liver failure. In livers without underlying parenchymal disease, resections of up to 75–80% of the total liver volume are considered safe in most centers [41, 42]. In patients with compromised livers secondary to alcoholic or non-alcoholic steatohepatitis, viral hepatitis, or parenchymal alterations after hepatotoxic chemotherapy (steatosis, sinusoidal dilatation, etc.) removal of lower liver volumes can severely impact postoperative liver function, and a remnant volume of at least 40% is considered acceptable [43]. Size, parenchymal quality, and adequacy of the arterial and portal blood supply, as well as the venous and biliary drainage of the future liver remnant, are the factors that need to be considered in assessing functional resectability and eventually surgical decision-making.
Volumetric Assessment of the Liver
CT volumetry as initially proposed by Heymsfield [44] is the current gold standard, and allows a relatively precise calculation of the total liver volume, tumor volume, and volume of the future liver remnant (FLR) [45]. Since the size of the presumed FLR is only a surrogate marker of postoperative liver function, discrepancies between CT volumetry, liver function, and postoperative outcome can occur [46]. Calculating a standardized future liver remnant by correlating the size of the FLR with the estimated total liver volume can help to personalize volumetric measurements and better evaluate the risk of resection [47]. Total liver volume estimation has been described by Urata [48] in an Asian or by Vauthey [49] in a Western population. Alternatively, risk assessment can be achieved by correlating FLR and body weight [50], and either method has proven its ability to predict the postoperative outcome after extended hepatic resections [51].
Enzymatic Liver Function Testing
Enzymatic liver function tests such as the indocyanine-green-(ICG)-clearance or LiMAx-test can help assess global hepatic function, and have been evaluated as a useful additional tool in the preoperative work-up and risk assessment of hepatic resection [52]. With a special regard to liver resection for colorectal liver metastases and the high number of patients pretreated with chemotherapy, an additional estimation of hepatic functional impairment after preoperative chemotherapy could potentially increase surgical safety.
The role of ICG-clearance seemed to be of limited value in assessing chemotherapy-associated liver injury [53]. In contrast, LiMAx has demonstrated a significant drop in enzymatic hepatic function after an oxaliplatin-based chemotherapy and a subsequent recovery within an average of 8 weeks after cessation of therapy. However, the pace of regeneration and time to functional recovery were highly different among patients [54]. Further studies are needed to corroborate the role of enzymatic liver function tests in liver surgery for colorectal metastases but could eventually impact surgical decision-making and timing of the procedure.
Imaging-Based Liver Function Testing
Imaging-based liver function tests were first developed in nuclear medicine and, compared to laboratory and enzymatic tests, have the advantage of additionally displaying the spatial distribution of liver function [55]. Using 99mTc-mebrofenin or 99mTc-galactosyl tracer scans, liver function can be assessed by planar scintigraphy. Single photon-emission computed tomography CT (SPECT-CT) offers hepatic function analysis in conjunction with 3D-volumetry, as does Gd-EOB-DTPA-enhanced MRI but featuring a higher temporal and spatial resolution than SPECT. MRI-based liver function assessment measures either biliary signal intensity during biliary elimination or the parenchymal contrast agent behavior over time by a region of interest analysis (see Fig. 4.3). It could be integrated seamlessly into the routine MRI-work-up at a relatively low expense [55].


Fig. 4.3
Gd-EOB-enhanced MRI 4 weeks after right portal vein embolization, T1-VIBE sequence after 20 min with flip angle of 30°. MRI with an increased excitation angle clearly shows the different signal intensities of the embolized and the non-embolized liver segments corresponding to a different function ratio (from [55] with permission)
The spatial distribution of liver function offered by imaging-based assessment could prove particularly helpful in the setting of hypertrophy induction following portal vein ligation or embolization, or as an intermittent assessment in between ALPPS steps (associating liver partition and portal vein ligation for staged hepatectomy).
Computer-Assisted Virtual Resection Planning in the 3D Liver Model
Ongoing development in CT imaging-based computer assistance has enabled an optimal visualisation of the intrahepatic vascular branching in a virtual 3D liver model, providing an individual territorial liver mapping as well as volume calculation of the corresponding vascular territories. Thus, liver resections can be planned with regard to the individual intrahepatic vascular anatomy and segmentation [56]. Resection planning is either fully automatic by the computer, with variable and freely selectable safety margins, or manually “free-hand” by the surgeon within the three-dimensionally reconstructed virtual liver [57]. The advantages of computer-assisted resection planning refer to a better assessment of functional resectability, since areas at risk for either devascularization or impaired venous drainage can be identified and precisely characterized preoperatively. Computer-assisted virtual resection planning, potentially combined with navigation, may evolve into a routine clinical practice in cases of complex and repeated liver resection for colorectal metastases in the future [40, 58–60] Fig. 4.4.


Fig. 4.4
Computation of volumetric risk for devascularization. a and b Transfer of the resection planned in Fig. 4.4a–c to the results of anatomic image evaluation as seen in Fig. 4.1. Portal vein, magenta; hepatic veins, cyan; plane of resection, yellow; remnant (remaining liver volume after resection planning in 2-dimensional computed tomography), green; and resected parenchyma, red. c Portal venous territories within remnant liver tissue. The red-bordered territory has been supplied by the dissected segment V branch and is exposed to devascularization after resection (accounting for 8% of the remaining tissue). d Territories within the remnant draining into the right hepatic vein (blue), and devascularized area (accounting for 6% of the remaining tissue) draining into a dissected small subbranch of the middle hepatic vein (red bordered). e Combined territories at risk for devascularization (accounting for 13% of the remaining tissue) for both blood supply and drainage (red bordered). Portal risk territory, magenta; risk for hepatic veins, cyan. Remaining functional liver volume after computer-assisted risk analysis (green), 679 ml. f Resection specimen after extended left hepatectomy. Ruler is in centimeters (from [61] with permission)
References
1.
2.
Garancini M, Gianotti L, Delitala A, Romano F, Degrate L, Giardini V. Intra-operative ultrasound: a review on its role in liver surgery for primitive and metastatic tumors. Minerva Chir. 2015;71(3):201–13.PubMed
3.
Cervone A, Sardi A, Conaway GL. Intraoperative ultrasound (IOUS) is essential in the management of metastatic colorectal liver lesions. Am Surg. 2000;66(7):611–5.PubMed
4.
5.
6.
Sahani DV, Bajwa MA, Andrabi Y, Bajpai S, Cusack JC. Current status of imaging and emerging techniques to evaluate liver metastases from colorectal carcinoma. Ann Surg. 2014;259(5):861–72.CrossrefPubMed
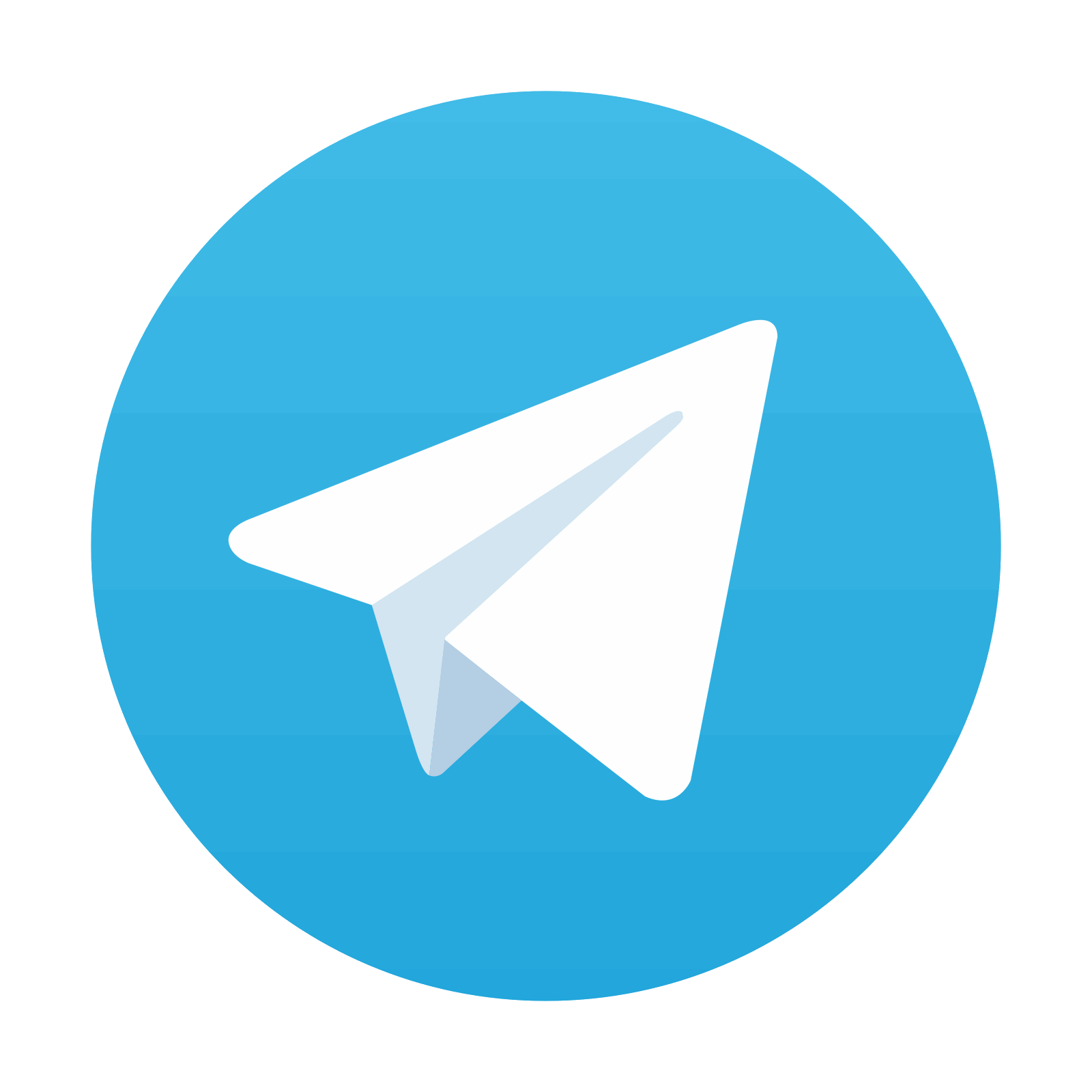
Stay updated, free articles. Join our Telegram channel

Full access? Get Clinical Tree
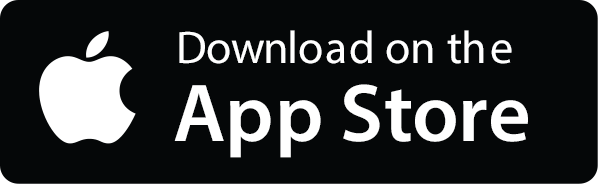
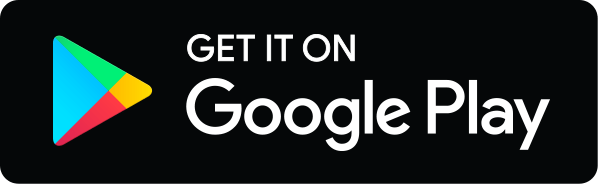