Fig. 5.1
The hypothetical maladaptive mechanisms likely involved in the etiopathogenesis of obesity-related alterations in GH/IGF-1 axis. The elevation of circulating FFA, adipokines, and cytokines induced by increased visceral adipose tissue depot responsible for insulin resistance at the liver, skeletal muscle, and pancreatic levels is also able to markedly affect the normal feedback control system operating in GH/IGF-1 axis. The “functional” low GH/IGF-1 axis, in turn, might act on body composition by inducing unfavorable changes in body composition similarly to those observed in GHD, thus contributing to worsen the insulin resistance state and the associated metabolic sequelae. SS somatostatin, GHRH growth hormone-releasing hormone, GH growth hormone, IGF-1 insulin-like growth factor-1, FFA free fatty acids
5.4 The Challenge to Diagnose Low GH Status in Obesity
It is of great practical importance to differentiate organic GHD versus obesity-related decrease in GH secretion as body fat and nutritional status are the main confounding factors in the interpretation of biochemical tests commonly used in the diagnosis of GHD [18]. Considering the pulsatile nature of GH secretion, the diagnosis of GHD would require multiple sampling to obtain a 24-h integrated GH profile. IGF-1 and IGFBP-3 are nonpulsatile GH target hormones, and IGF-1 levels <2 standard deviation below the age-matched mean, in a well-nourished adult with pituitary disease, are a strong evidence for significant GHD. However, a provocative test of GH reserve is required for many adult patients with suspected GHD. The assessment of the GH secretion is commonly performed using pharmacological challenges such as the insulin tolerance test (ITT) or the GHRH+arginine. The latter, using the effect of arginine in potentiating the response to GHRH via inhibition of hypothalamic release of somatostatin, is currently considered the favorite diagnostic tool due to its high specificity and sensitivity, as well as tolerability. While BMI specific cutoff points for the diagnosis of severe GHD has not yet been available for ITT, GHRH+arginine is the only test for which BMI-dependent variability of GH responsiveness has been investigated [19]. In particular, appropriate GH cutoff in adults with BMI >30 kg/m2 is considered 4.2 μg/L [20]. Using this new cutoff value of the GHRH+arginine test, about one third morbidly obese individuals presented with a low peak GH [2]. In addition, waist circumference adds per se predictive information to the determination of GH response, independent of BMI [21]. The adipose tissue effects on GH secretion have also been confirmed in healthy adolescents with normal stature during transition period [22], although appropriate cutoff values for overweight/obese adolescents are still missing. In Table 5.1 are reported cutoff values of peak GH after different stimulation tests according to BMI. The main criticism to GH stimulation testing in obesity is that this functional hyposomatotropism is fully reversed by weight loss and might cause misleading diagnosis of GHD [23], especially in view of a possible expensive unjustified treatment with GH. Whatever might be the central and peripheral mechanisms linking adipose tissue and low GH status, this condition, albeit functional, serves as a vicious circle that is reinforced with further accumulation of visceral adipose tissue. Thus, beyond the issue to erroneously classify obese individuals’ low peak GH as really GH deficient, a low status of GH/IGF-1 axis, especially when the low GH secretion is paralleled by low IGF-1 levels, is however responsible for unfavorable changes in body composition and increased cardiovascular risk profile, similarly to those observed in organic GHD [24]. This hypothesis is in line with recent reports suggesting that low IGF-1 levels are linked to the pathogenesis of type 2 diabetes, metabolic syndrome, cardiovascular disease, and chronic inflammation [25, 26].
Table 5.1
Cutoff values of peak GH after GHRH +arginine according to BMI
First author (Ref.) | Age | BMI | Cutoff values (μg/L) |
---|---|---|---|
Corneli et al. [20]a | Adults | <25 kg/m2 | <11.5 |
25–30 kg/m2 | <8.0 | ||
>30 kg/m2 | <4.2 | ||
Colao et al. [19]a | Adults | <25 kg/m2 | 11.8 |
25–30 kg/m2 | 8.1 | ||
>30 kg/m2 | 5.5 | ||
Perotti et al. [22] | Transition period | >85th percentile | <19 |
5.5 Metabolic Consequences of Low GH/IGF-1 Status in Obesity
The low GH/IGF-1 axis status is associated with a different obesity phenotype. In particular, obese individuals with blunted peak GH showed a significantly different body composition compared with normal responder counterpart, with the highest values of BMI, waist circumference, waist-to-hip ratio, and fat mass, and the lowest fat-free mass [1, 2, 12]. A similar association is found in patients with Prader-Willi syndrome (PWS), the most common known genetic cause of marked obesity characterized by multiple endocrine dysfunctions, including a reduced GH secretory capacity, who presented with changes in body composition that are worse than those observed in obese subjects with comparable BMI. In this respect, it is conceivable that the unfavorable changes in body composition associated with the low GH/IGF-1 status contribute to worsen the obesity-related metabolic sequelae, amplifying the cardiometabolic risk profile in the subset of obese individuals with low GH status. Accordingly, obese individuals with reduced GH secretion have increased cardiovascular disease risk factors, including dyslipidemia, chronic inflammation, and increased carotid intima-media thickness, and increased prevalence of metabolic syndrome [1, 27]. In addition, low peak GH has also been reported to be associated with increased intramyocellular and intrahepatic lipid content in premenopausal obese women, further suggesting the contribution of low GH status in the development of insulin resistance and other complications of the metabolic syndrome [28]. The cardiovascular risk profile increases when there is concordance between reductions in peak-stimulated GH and low IGF-1 levels in the same patient [17]. Thus, there may be different degrees of impairment of GH/IGF-1 axis in obesity. Further evidence on the effect of the low GH status in obesity is provided by the studies evaluating the effectiveness of GH treatment in obese individuals.
5.6 Effectiveness of GH Treatment in Obesity
A number of clinical trials have assessed the efficacy of recombinant human (rh) GH as adjuvant therapy in association with a standard weight loss program for unselected obese adults [1]. The major issues in evaluating rhGH treatment in obesity are the limited number of participants, the lack of placebo control, the short duration of the treatments, and the doses used. A recent comprehensive meta-analysis by Mekala et al. [29] has evidenced that rhGH treatment leads to decrease in total fat mass, visceral adiposity, and increase in lean body mass, even if without resulting in significant weight loss. Along with these little, albeit significant, changes in body composition, plasma lipid profile is improved, with decreases in total cholesterol and LDL cholesterol. In addition, no significant changes in markers of adiposity, such as leptin or adiponectin, are reported. In short-term studies, fasting plasma glucose and insulin increase transiently during the first few weeks of GH treatment, whereas improvements in glucose metabolism are observed only in long-term studies. In particular, significant beneficial effects on fat mass and glucose metabolism are observed in the studies reporting a longer mean duration of treatment (>12 weeks) compared to the short-term studies. This evidence is likely due to the longer lap of time required to evidence the insulin-sensitizing effects due to the reduction in visceral adipose tissue and the increase in IGF-1 levels. Accordingly, similar beneficial effects of GH treatment have been reported in obese type 2 diabetic patients with poor glycemic control [30]. Alternatively, GH treatment might act through decreased 11βHSD1 activity in visceral adipose tissue, increased glucose transport in the skeletal muscle, the major site of glucose disposal mediated via the IGF-1 receptor, increased proportion of insulin-sensitive type I muscle fibers, or increased capillarization in the skeletal muscle. There is no general agreement on the effective dose of rhGH in obese individuals. Many trials used relatively higher doses of rhGH compared with those recommended for adult GHD. Indeed, low doses that normalize age-related IGF-1 levels are more likely to minimize the number of dose-related side effects and the unfavorable effects on glucose metabolism, such as GH-induced hyperinsulinemia, which may oppose the lipolytic effect of GH. This observation suggests that a careful investigation of GH/IGF-1 axis might be mandatory in the evaluation of obese individuals to be assigned to rhGH treatment. In this respect, it should be conceivable to limit the therapy to only subjects with reduced GH secretory capacity, in relation to their worse cardiometabolic risk profile, and for a period of time sufficient to obtain the spontaneous recovery of GH/IGF-1 axis, if any, along with weight loss. The adverse events most commonly reported are hypertension, arthralgia, paresthesia, and peripheral edema; more recently, an increased severity of obstructive sleep apnea in abdominally obese men during GH treatment likely due to the GH-induced increase of measures of neck volume has been reported [31]. Of interest, either the beneficial effects on body composition and cardiovascular risk or the side effect of altered glucose tolerance returns to pretreatment levels after GH withdrawal. Finally, GHRH analogues increasing endogenous GH secretion, such as tesamorelin [32], have recently evidenced to be efficacious in improving body composition without adversely affecting glucose metabolism, while studies on GH fragments with predominant antilipolytic activity have not yet yielded convincing results.
5.7 GH/IGF-I Axis and Bariatric Surgery
Bariatric surgery currently provides the only long-term control of obesity, resulting in major weight loss and weight maintenance. Up to now, very few well-designed clinical trials have been performed to scientifically investigate the GH/IGF-1 axis following bariatric procedures. Bariatric surgical techniques can be separated in malabsorptive procedures, such as biliopancreatic diversion (BPD), and restrictive procedures, such as laparoscopic adjustable gastric banding (LAGB) or sleeve gastrectomy, with a growing variety of procedures using one or both of these techniques over the years, such as the Roux-en-Y gastric bypass (RYGB). Among the postsurgical endocrine changes, those related to the GH/IGF-I axis have been poorly investigated. Stimulated GH secretion significantly increases after BPD [33]. Partial recovery of GH secretion is also observed after RYGB [34]. Although with some discrepancy [35, 36], postoperative IGF-1 secretion shows a long-lasting impairment, similarly to that showed after nonsurgical weight loss, presumably linked to the catabolic state induced by BPD [37] and RYGB [38]. Yet, in a prospective study 6 months after LAGB, when the initial catabolic state should have already been overcome, about 20 % obese women are found to be both GH and IGF-1 deficient and another fifth have IGF-1 levels still below the normal range calculated according with age normative ranges [13]. Interestingly, excess of weight loss (EWL) and fat mass loss are higher in patients who normalize their GH/IGF-1 axis after surgery than in those who do not [39]. Thus, the question arises as whether persistent failures of the GH/IGF-1 axis might negatively influence the outcome of bariatric surgery. Indeed, percent decrease of waist circumference, EWL, and fat mass is greater in obese individuals with normal preoperative GH secretion and IGF-1 levels, suggesting that the evaluation of GH/IGF-1 axis might be useful to predict the individual postoperative outcome [39].
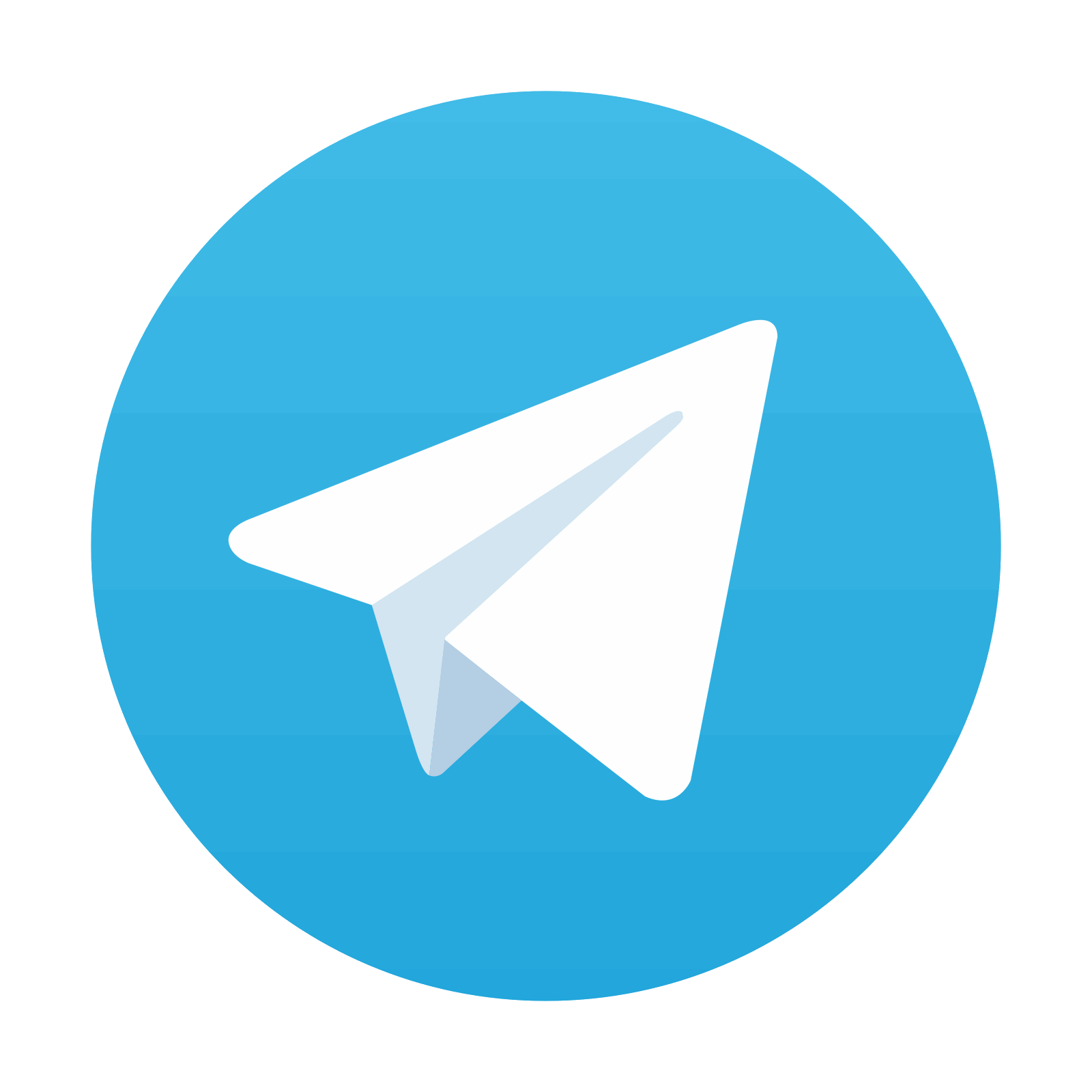
Stay updated, free articles. Join our Telegram channel

Full access? Get Clinical Tree
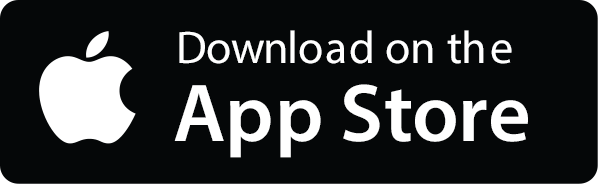
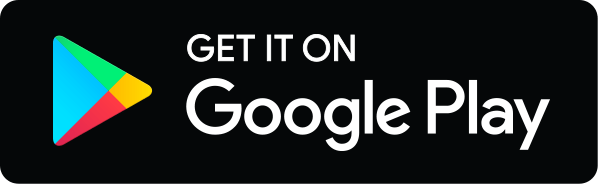