Abstract
The serum sodium concentration is the ratio of sodium to water in the extracellular fluid compartment. It is determined by the relationship among total body sodium, potassium, and water, the latter of which typically is the main determinant. This chapter focuses on the etiology and management of hypernatremia, a hyperosmolar state. Hypernatremia itself is not a disorder but rather a laboratory abnormality caused by an underlying pathologic process. To simplify the management of hypernatremia, this chapter considers the balance of total body water and sodium separately. The goal is to determine appropriate, timely therapies for a disorder that is associated with a high degree of morbidity and mortality.
Keywords
hypernatremia, hyperosmolarity, hypertonicity, water balance, sodium balance, dehydration, antidiuretic hormone
Definitions
Normal serum [Na + ] is 135 to 145 mEq/L. This range is generally maintained despite large individual variations in salt and water intake. Hypernatremia is always a water problem and sometimes also a salt problem. There is no predictable relationship between serum [Na + ] (a measure of osmolality and tonicity) and total body salt or volume status. More specifically, although hypernatremia confirms the presence of a relative water deficit, the isolated laboratory finding of a serum [Na + ] greater than 145 mEq/L does not reveal anything about a person’s volume status. As a result, hypernatremia may occur in the setting of hypovolemia, euvolemia, or hypervolemia, as indicated in Fig. 8.1 .
Dehydration and Volume Depletion
Comparing the terms “dehydration” and “volume depletion” illustrates the distinction between water and sodium balance. Although dehydration is commonly used to describe a person’s volume status, this use is inaccurate. Dehydration does not equal volume depletion. Rather, dehydration is a description of water balance, whereas volume depletion refers to a person’s sodium balance. Although these two clinical scenarios may coexist, they should not be confused. It is important that the two terms are not used interchangeably and that a patient’s water and sodium balance be considered independently when addressing dysnatremias clinically.
Hyperosmolality and Hypertonicity
Hypernatremia always reflects a hyperosmolar state, whereas the reverse is not always true. For example, hyperosmolality may also be a consequence of severe hyperglycemia or elevated blood urea nitrogen, as is seen in kidney failure. Furthermore, hyperosmolality does not necessarily mean hypertonicity. For example, uremia is a hyperosmolar but not a hypertonic state. Urea is an ineffective osmole because it can freely cross cell membranes, unlike sodium; therefore urea contributes to osmolality but not to tonicity. In contrast to urea, sodium, which is unable to cross cell membranes freely, is an effective osmole and is the primary cation that affects plasma osmolality (P osm ). Hypernatremia is a hypertonic state in which water will flow from the intracellular to the extracellular space, resulting in cellular dehydration and shrinkage.
Background
Hypernatremia is all about water. A more accurate term for hypernatremia might be “hypoaquaremia” because it literally means a state in which there is too little water in the intravascular space and therefore too little water in the intracellular space. To begin any discussion of hypernatremia (or hyponatremia), it is important to understand that dysnatremias are actually disorders of water homeostasis.
Water distributes throughout all body compartments, with two-thirds in the intracellular and one-third in the extracellular compartment. Three-quarters of the water in the extracellular compartment is located in the interstitial space, and one-quarter is in the intravascular space. Water is lost (or gained) in the same proportions as it is distributed throughout all body compartments. Pure water loss does not affect plasma volume status or hemodynamics significantly until very late because of the normal distribution of water throughout all body compartments. For example, for every 1 L of water deficit, only approximately 80 mL is lost from the intravascular (plasma) compartment.
Epidemiology
The incidence of hypernatremia in all hospitalized patients ranges from less than 1% to approximately 3%. However, in critically ill patients, the overall prevalence of hypernatremia ranges between 9% and 26% and is hospital acquired in approximately 80% of these cases. Hypernatremia present at the time of hospital admission is primarily a disease of older adults and of those with mental illness or impaired sensorium. Most patients with hypernatremia on admission to the hospital have concomitant infections. Hypernatremia that is present on hospital admission is generally treated earlier than hypernatremia that develops during the hospital course, most likely because of increased attention paid to individual laboratory values and volume status on hospital admission.
In contrast, hospital-acquired hypernatremia is typically seen in patients who are younger than those with hypernatremia on admission, with an age distribution similar to that of the general hospitalized population. Hospital-acquired hypernatremia is largely iatrogenic from inadequate and/or inappropriate fluid prescription and therefore is largely preventable. It results from a combination of decreased access to water, disease processes that may increase insensible losses or interfere with the thirst mechanism, and administration of loop diuretics. Approximately half of patients with hospital-acquired hypernatremia are intubated and therefore have no free access to water. Of the remaining 50%, most have altered mental status.
Patients at highest risk for hospital-acquired hypernatremia are those at the extremes of age (infants and older adults), those with altered mental status, and those without access to water (i.e., intubated or debilitated patients). In addition to the impaired thirst and decreased urinary concentrating ability that accompany advanced age, older patients have a lower baseline TBW content, making smaller changes in water balance more clinically relevant.
Clinical Manifestations
Symptoms
Clinical symptoms related to hypernatremia can be attributed to cellular dehydration and shrinkage due to the loss of intracellular water. Loss of intracellular water occurs throughout the body, but the primary symptoms are neurologic. The severity of neurologic symptoms is more dependent on the rate of rise in serum [Na + ] than on the absolute value. Polyuria and polydipsia are frequently the presenting symptoms of diabetes insipidus (DI), with or without the presence of hypernatremia.
Neurologic symptoms comprise a continuum that begins with fatigue, lethargy, irritability, and confusion and progresses to seizures and coma. Additional symptoms of hypernatremia include anorexia, nausea, vomiting, and generalized muscle weakness. Altered mental status can be both a cause and an effect of hypernatremia and consequently can be difficult to distinguish clinically. In addition, cellular dehydration and shrinkage can lead to rupture of cerebral veins because of traction, which results in focal intracerebral and subarachnoid hemorrhages; this occurs more often in infants than in adults.
Signs
Signs of hypernatremia depend, in part, on its cause and severity. Abnormal subclavicular and forearm skin turgor and altered sensorium are commonly found in patients with hypovolemic or euvolemic hypernatremia, whereas patients with hypervolemic hypernatremia typically have classic signs of volume overload, such as elevated neck veins and edema.
Pathophysiology
A sound understanding of the normal physiology of water and salt balance is integral to the understanding and management of dysnatremias. The intracellular and extracellular body compartments exist in osmotic equilibrium. The development of hypernatremia is most commonly the result of increased water losses combined with inadequate intake. Rarely does hypernatremia occur as a consequence of excessive sodium intake.
Regulation of plasma [Na + ] is determined by the regulation of P osm , of which plasma [Na + ] is the primary determinant. Normal P osm is between 285 and 295 mOsm/kg. If the P osm varies by 1% to 2% in either direction, physiologic mechanisms are in place to return the P osm to normal. In the case of hypernatremia or hyperosmolality, receptor cells in the hypothalamus detect increases in P osm ; in response, they stimulate thirst to increase water intake and simultaneously stimulate antidiuretic hormone (ADH) release to limit renal water losses (by increasing water reabsorption in the collecting duct). Under normal conditions, the body is able to maintain the serum osmolality under tight control. The goal of “normonatremia” is to avoid changes in cellular volume and thereby prevent potential disruptions in cellular structure and function. The body’s normal physiologic defense against hypernatremia is twofold: an endogenous thirst stimulus and renal conservation of water.
As with other electrolyte disturbances, the pathophysiology of hypernatremia can be easily categorized into two phases—an initiation phase and a maintenance phase. Simply stated, the initiation, or generation, phase must be caused by a net water loss or, less commonly, a net sodium gain. For hypernatremia to exist as anything more than a transient state, there must be a maintenance phase, defined necessarily by inadequate water intake.
Water metabolism is primarily controlled by arginine vasopressin (AVP) or ADH, as it is commonly termed. ADH is produced in the hypothalamus (supraoptic and paraventricular nuclei) and is stored in and secreted by the posterior pituitary. ADH release can be stimulated by either increases in P osm or decreases in mean arterial pressure or blood volume. In the setting of hypernatremia, the primary stimulus for the release of ADH comes from osmoreceptors located in the hypothalamus. ADH acts on the vasopressin type 2 (V 2 ) receptors in the collecting duct to cause increased water reabsorption from the tubular lumen via insertion of aquaporin-2 channels.
The kidney’s primary role in hypernatremia is to concentrate the urine maximally, preventing further loss of electrolyte-free fluid. For the kidney to do so, the following must be present: (1) a concentrated medullary interstitium, (2) ADH to insert aquaporin-2 channels into the apical membranes of the collecting duct, and (3) the ability of collecting duct cells to respond to ADH.
In a steady state, water intake must equal water output. Obligatory renal water loss is directly dependent on solute excretion and urinary concentrating ability. If a person has to excrete, for example, 700 mOsm of solute per day (primarily Na + , K + , and urea), and the maximum urinary osmolality (U osm ) is 100 mOsm/kg, then the minimum urine output requirement will be 7 L. However, if the kidneys are able to concentrate the urine to a U osm of 700 mOsm/kg, urine output would need to be only 1 L.
Thirst, on the other hand, is an ADH-independent mechanism of defense against hypertonicity. Like ADH release, thirst is triggered by osmoreceptors located in the hypothalamus. The intense thirst stimulated by hypernatremia may be impaired or absent in patients with altered mental status or hypothalamic lesions and in older adults. It is important to note that patients with moderate to severe increases in electrolyte-free water losses may maintain eunatremia because of the powerful thirst mechanism. For example, a patient with nephrogenic DI may maintain a normal serum [Na + ] if given unlimited access to water but may develop marked hypernatremia in situations in which access to water is restricted (e.g., altered mental status, mechanical ventilation, nothing by mouth status during hospitalization).
Although ADH activity is a pivotal physiologic defense against hyperosmolality, only an increase in water intake can replace a water deficit. An increase in ADH activity in collecting tubules can only help to decrease ongoing water losses but cannot replace water that has already been lost. Therefore both ADH-dependent and ADH-independent mechanisms are integral to the body’s efforts to protect against hypernatremia or hyperosmolality.
The brain has multiple defense mechanisms designed to protect it from the adverse effects of cellular dehydration. As the serum [Na + ] rises, water moves from the intracellular to the extracellular space to return the serum osmolality to the normal range. Almost immediately, there is an increase in the net leak of serum electrolytes (primarily Na + and K + ) into the intracellular space, which increases intracellular osmolality. In addition, there is an increased production of cerebrospinal fluid, with movement into the interstitial areas of the brain. Within approximately 24 hours, brain cells generate osmolytes or idiogenic osmoles (e.g., amino acids, trimethylamines, myoinositol) to increase intracellular osmolality to draw water intracellularly. This process restores intracellular volume over a period of days, thereby decreasing the adverse clinical impact of hypernatremia (i.e., cellular dehydration). The increase in transcellular transport of electrolytes is somewhat transient because over time it interferes with normal cellular function. Idiogenic osmoles clearly serve a protective role, but their removal is also slow (days) when isotonicity has been reestablished. The clinical implication of the slow removal of these idiogenic osmoles is that correction of hypernatremia (hypertonicity) must be gradual to avoid cellular swelling or cerebral edema.
Pathogenesis and Diagnostic Approach
Hypernatremia most commonly results from the combination of increased water loss and decreased water intake. Any clinical condition associated with increased water loss or decreased water intake predisposes to hypernatremia. In general, for hypernatremia to occur, the rate of water excretion must exceed that of water intake. Hypernatremia secondary to sodium loading, which is less common, is an exception to this basic principle.
Water losses occur via the genitourinary and gastrointestinal tracts, as well as via the skin and respiratory tract as insensible losses. DI and osmotic diuresis result in excess urinary loss of water, and excess water is lost via stool in severe diarrhea. Conditions that increase insensible losses include fever, burns, open wounds, and hyperventilation.
Although hypernatremia is due to an imbalance of water homeostasis, there may be a concomitant salt disturbance. After a thorough clinical history is obtained and a complete physical examination performed, the first decision point in the evaluation of any patient with hypernatremia is to determine the patient’s sodium balance or volume status (see Fig. 8.1 ). Hypernatremia can be seen in patients whose sodium balance is negative (hypovolemic), normal (euvolemic), or positive (hypervolemic), but in all cases a negative water balance is present. Determining the patient’s sodium balance is essential to planning the appropriate therapy.
Negative Sodium Balance
Hypernatremia due to the loss of hypotonic fluids is associated with both a negative sodium balance and a negative water balance ( Table 8.1 ). In such cases, hypernatremia develops because the sustained loss of water exceeds that of sodium. These patients usually manifest typical signs of sodium depletion, or hypovolemia, such as tachycardia and orthostatic hypotension. Determination of the urine sodium concentration ([U Na ]) can help distinguish between renal losses, such as from diuretics or osmotic diuresis ([U Na ] >20 mEq/dL), and extrarenal losses, such as from diarrhea or vomiting ([U Na ] <20 mEq/dL).
RENAL CAUSES OF WATER DEFICIT | ||
---|---|---|
Salt Deficit (Urine [Na + ] >20 mEq/dL) | Salt Balanced (U osm /P osm <1) | Salt Excess |
Diuresis
| Diabetes Insipidus (See Box 8.1 )
| Mineralocorticoid excess |
EXTRARENAL CAUSES OF WATER DEFICIT | ||
Salt Deficit (Urine [Na + ] <20 mEq/dL) | Salt Balanced (U osm /P osm <1) | Salt Excess |
Gastrointestinal
Cutaneous
| Increased Insensible Losses
Decreased Intake
Intracellular Shift
| Excessive Na + Intake
|
Normal Sodium Balance
Hypernatremia in patients with a normal sodium balance results from the loss of electrolyte-free fluid, or pure water (see Table 8.1 ). These patients have a normal total body sodium (and are therefore euvolemic) but are TBW depleted. The causes of hypernatremia in patients with a normal sodium balance may be renal or extrarenal. In these cases, urine osmolality (U osm ), which reflects ADH levels and function, is often more helpful than U Na . A low U osm is consistent with renal losses and therefore with low ADH levels or function (DI), whereas a high U osm suggests extrarenal losses of free water and intact secretion of and response to ADH.
Etiologies of DI may be central, nephrogenic, or gestational ( Box 8.1 ). The key diagnostic step in determining a central versus a nephrogenic cause is based on the response to exogenous hormone replacement (i.e., vasopressin). A finding of little or no increase in U osm after administration of exogenous vasopressin is diagnostic of nephrogenic DI. However, it is important to remember that central or nephrogenic DI may be partial: either ADH is present but in insufficient quantity (partial central DI) or there is an incomplete response to ADH in the collecting duct (partial nephrogenic DI).
ADH Dependent
Central DI (Lack of ADH Release)
Congenital
Trauma
Neurosurgery
Primary or secondary CNS tumor
Infiltrative disorder
Sarcoidosis, tuberculosis
Hypoxic encephalopathy
Post cardiac arrest, Sheehan syndrome
Hemorrhage
Infection
Meningitis, encephalitis
Aneurysm
Idiopathic
Nephrogenic DI (Unresponsive to ADH)
Hereditary
X-linked recessive
Defect in V 2 receptor or aquaporin channel
May be complete or partial
Gestational DI (Degradation of ADH)
Vasopressinase mediated
Occurs in peripheral circulation
ADH Independent
Acquired Nephrogenic DI
Electrolyte disturbances
Hypercalcemia
Hypokalemia
Drug induced
Lithium, demeclocycline, amphotericin B,
foscarnet, methoxyflurane, vaptans
Chronic interstitial kidney disease
Medullary cystic disease, sickle cell disease,
amyloidosis, Sjögren syndrome
Malnutrition
Decreased medullary gradient
ADH , Antidiuretic hormone; CNS, central nervous system; DI, diabetes insipidus.
One rare form of DI is gestational, or pregnancy-related, DI, which is caused by production of placental vasopressinase. Gestational DI should be evident from the clinical history. The manifestations are similar to those of nephrogenic DI in that there is little or no increase in U osm with exogenous vasopressin; however, gestational DI responds to desmopressin acetate (dDAVP), a synthetic analogue of ADH that is unaffected by vasopressinase.
Nephrogenic DI can be either hereditary (genetic defect of the V 2 receptor gene or aquaporin water channel) or acquired. Acquired nephrogenic DI may be reversible and includes any clinical condition in which the kidney is unable to maximally concentrate the urine. The mechanisms of acquired nephrogenic DI are ADH independent. The most common cause of acquired nephrogenic DI is chronic lithium use. The mechanism of lithium-induced nephrogenic DI includes both a decrease in density of V 2 receptors and decreased expression of aquaporin-2 channels. Hypercalcemia, hypokalemia, and severe malnutrition are other common examples of reversible nephrogenic DI. Hypercalcemia can induce a reversible nephrogenic DI through inhibition of sodium reabsorption in the loop of Henle, which impairs the generation of an adequate medullary gradient and reduces concentrating ability. In addition, dysregulation of the aquaporin-2 channel can be seen with hypercalcemia. Hypokalemia causes nephrogenic DI by decreasing collecting tubule responsiveness to ADH. Decreased protein intake leads to decreased urea production and therefore a decreased medullary gradient with inability to maximally concentrate the urine.
A high U osm suggests extrarenal losses as the cause of euvolemic hypernatremia. To generate a high U osm , the kidney must be able to concentrate the urine, an ability that requires intact ADH-dependent mechanisms. Insensible losses are the primary source of electrolyte-free water loss in this subgroup of patients. Increased insensible losses occur via the skin (burns, sweat), respiratory tract (tachypnea), or both.
Finally, patients with hypodipsia or adipsia may develop euvolemic hyponatremia. Most often, they have normally functioning kidneys but lack adequate water intake. These patients typically have a high U osm and low urine output. Idiopathic hypodipsia occurs, but identification of an impaired thirst mechanism as the primary disorder causing hypernatremia should lead to a more thorough neurologic investigation to rule out the presence of hypothalamic tumors or disorders. An impaired thirst mechanism or limited access to water in the setting of DI can result in severe hypernatremia and can be life threatening.
Positive Sodium Balance
Hypernatremia resulting from total body sodium gain is the least common type of hypernatremia (see Table 8.1 ). In these cases, total body sodium is uniformly increased, but TBW may be increased or unchanged, depending on the cause. An increase in extracellular volume should be readily identifiable by the presence of hypervolemia on clinical examination. This clinical presentation is usually iatrogenic, resulting from hypertonic fluid administration (saline or bicarbonate), and it reflects a gain of sodium without an appropriate gain of water. Excess mineralocorticoid activity can also result in hypernatremia with a positive sodium balance and, in the absence of typical iatrogenic risk factors, should alert the clinician to evaluate for potential causes of mineralocorticoid excess.
An example of hypernatremia in the setting of positive sodium balance is the hemodynamically stable hypernatremic patient with acute respiratory distress syndrome (ARDS) and an elevated central venous pressure. This relative hypervolemic hypernatremic state reflects an imbalance of both water and salt. Commonly, the physician might be concerned that administration of the free water necessary to correct the serum [Na + ] (e.g., 3 L) would cause the patient to become fluid overloaded. This would be in direct contrast to the goal of a net negative fluid balance for optimal management of ARDS. However, because of the normal distribution of water, less than 10% (i.e., <300 mL for administration of 3 L) of the administered water, either intravenously or enterally, would remain in the vascular space; therefore the fluid administration would not materially impact the patient’s volume status. In addition, it is imperative to understand that further diuresis to obtain a net negative sodium balance, in the absence of water administration, will exacerbate the hypernatremia by increasing free water urinary losses.
Treatment
Treatment goals of hypernatremia include both replacement of the free water deficit and prevention or reduction of ongoing water loss. The amount, route, and rate of replacement depend on the severity of symptoms, rate of onset, concurrent clinical conditions, and the patient’s sodium balance. Correction of the latter in a hemodynamically unstable patient is always a priority, no matter how severe the hypernatremia. In this setting, ECF depletion should always be corrected with 0.9% saline before the water deficit is addressed. After the patient is hemodynamically stable, it is important to focus on the treatment of the water deficit because the complications of hypernatremia frequently result from its inappropriate correction or treatment, not the electrolyte disturbance itself. Management of hypernatremia should include identification of the underlying cause in addition to correction of the hypertonic state. Treatment of hypernatremia can, most often, be broken down into the following seven steps ( Box 8.2 ).
Step 1. Determine sodium balance.
Step 2. Calculate free water deficit.
Step 3. Choose a replacement fluid.
Step 4. Determine rate of repletion.
Step 5. Estimate ongoing “sensible” losses.
Step 6. Estimate ongoing “insensible” losses.
Step 7. Determine underlying cause, if possible.
Step 1
Determine Sodium Balance.
Evaluation of the patient’s sodium balance, or volume status, is a critical first step for both appropriate diagnosis and treatment of hypernatremia. This information should be obtained through a thorough history and physical examination. If the patient is hemodynamically unstable from sodium depletion, this should be addressed first with the administration of normal saline.
Step 2
Calculate Free Water Deficit.
Before initiating therapy, it is both prudent and appropriate to quantify the deficit and develop a treatment plan for the individual patient. Calculation of the water deficit represents only a snapshot in time. If it were possible to prevent any further water losses, insensible or otherwise, the calculated water deficit would be the amount that must be administered to normalize the serum [Na + ], as shown in Box 8.3 , Formula 1:
Water deficit = TBW × ( plasma [ Na + ] / 140 − 1 ) = ( 0.5 or 0.6 ) × lean body weight × ( plasma [ Na + ] / 140 − 1 )
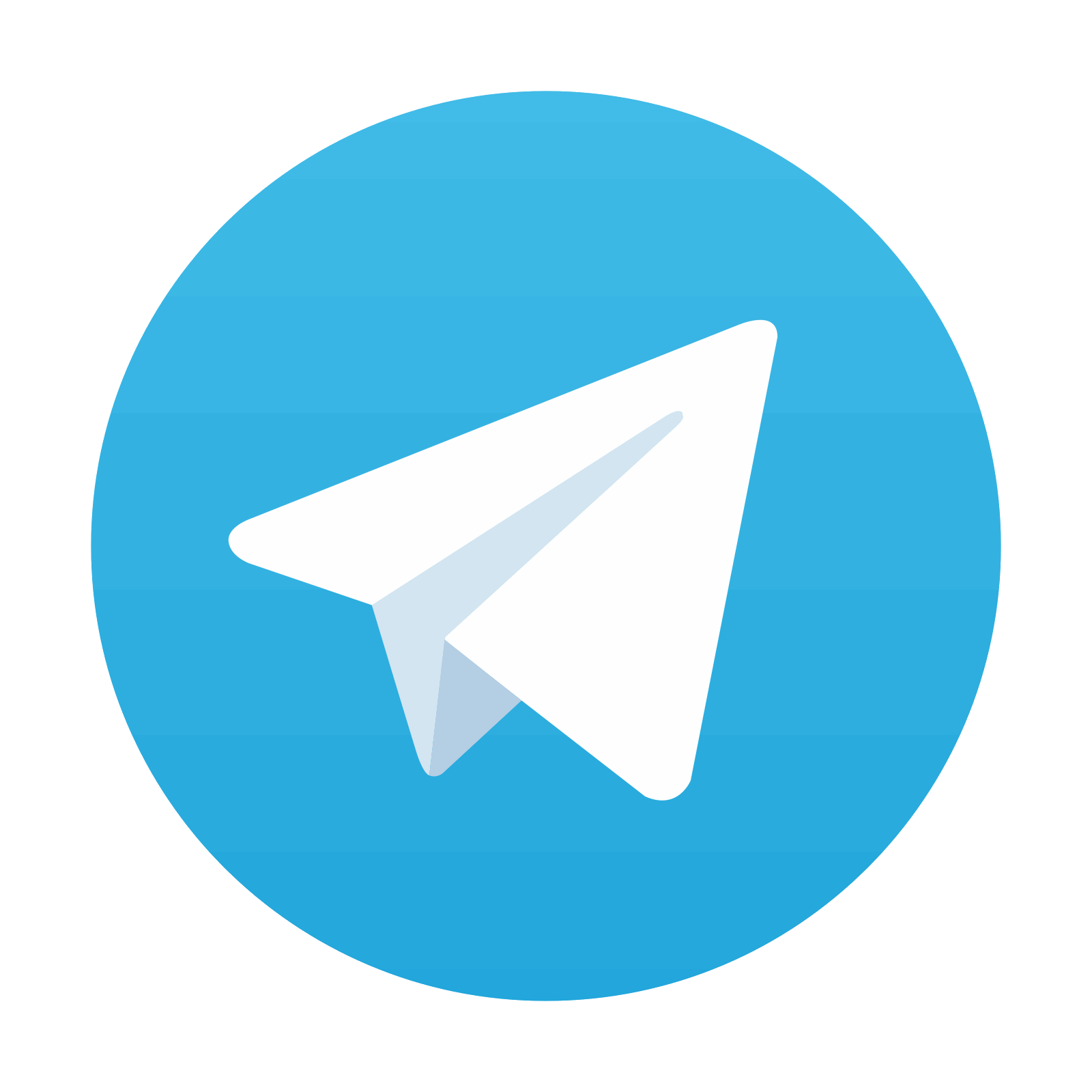
Stay updated, free articles. Join our Telegram channel

Full access? Get Clinical Tree
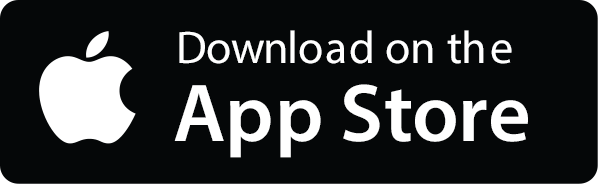
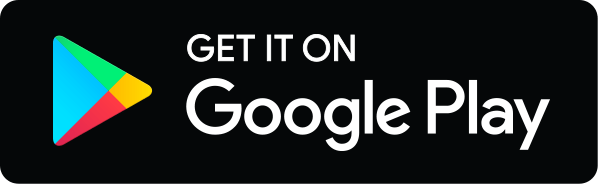