© Springer Science+Business Media Dordrecht 2015
Youhe Gao (ed.)Urine Proteomics in Kidney Disease Biomarker DiscoveryAdvances in Experimental Medicine and Biology84510.1007/978-94-017-9523-4_44. Human Urine Proteome: A Powerful Source for Clinical Research
(1)
Core Facility of Instrument, Institute of Basic Medical Sciences, Chinese Academy of Medical Sciences and Peking Union Medical College, Dong Dan San Tiao, Beijing, 100005, China
Abstract
As noninvasive and easily available biological fluid, urine is becoming an ideal sample for proteomic study. In recent years, researchers endeavored in profiling urinary proteome and discovering potential disease biomarkers. However, there are still many challenges in the studies of urinary proteome for the complexity of urine. In this article, we review current status of urinary sample preparation, including collection, storage, and extraction of urinary proteins, and the overall urinary proteome analysis so far, which may be helpful for urinary proteome analysis.
Keywords
Urinary proteomeMass spectrometryPreparation and storageNormal human4.1 Introduction
Body fluids have been regarded as the significant source of biomarkers, which could be used for the early diagnosis and state forecast of clinical diseases [1–4]. As an easily obtainable biological fluid, urine plays a crucial role in the clinical proteomics.
Urine is excreted by the kidney to eliminate waste products from plasma. About 150–180 L of plasma is filtered by glomeruli to develop the “primitive urine.” More than 99 % of “primitive urine” is reabsorbed by the renal tubule, and the “final urine” is remained to be excreted [5]. Approximately 30 % of urinary proteins originate from the plasma proteins, whereas 70 % comes from the kidney and the urinary tract [6, 7]. Therefore, the urinary proteome might supply important biomarkers directly reflecting the functions of the kidney and related organs [8]. Urine has several advantages to discover biomarkers of diseases: (1) It is easy to be collected in large amount and noninvasive way and (2) urinary samples are less complex than plasma and carry many proteins, peptides, and amino acids that have not been discovered in plasma [9]. Therefore, many researchers did their best to have a deeper understanding of urinary proteins and discover potential biomarkers in recent years.
However, there still are many difficulties and problems needed to further explore and study. In this review, we summarize achievements of urinary proteomics, including sample collection, preparation, and urinary proteome analysis in recent years, which may be helpful for further studies.
4.2 Collection and Storage
4.2.1 The Types of Urine
In clinic, many kinds of urinary samples have been used according to the different examination. However, many factors may influence the components of urinary proteome [10], including daily activities, physiological variations, and environmental factors such as temperature and pH. Therefore, several types of urinary samples have been analyzed by proteomic approach, including first-morning urine, 24-h urine, second-morning urine, random urine, and the urine collected after drinking a large amount of water or after drinking coffee.
Twenty-four-hour urine can show the excretion of urinary proteins within a day [11]. But the collection of 24-h urine depends on patient compliance, which is unpractical to be completed entirely and easy to have some errors during the collection process [12]. Concerning first-morning urine, it cannot exhibit urine “diurnal variation” (different time-points’ variation in all day) [11]. And Hoorn et al. [13] reported that first-morning urine may have bacterial contamination due to the long residence time of bladder.
Sun et al. [10] made a qualitative and quantitative analysis of five samples (first-morning void, second-morning void, excessive water-drinking void, random void, and 24-h void) collected in 1 day from healthy volunteers by 1-D LC/MS. They found no significant differences in the protein numbers of these five samples, and 42 common proteins to five samples contributed an average of 88.7 % of abundance to each sample. Thongboonkerd et al. [14] compared four different time-points urine, including first-morning urine, afternoon urine, water-loading urine, and urine after drinking a cup of coffee. They found the first-morning urine contained greater amount of proteins, but less protein spots visualized in 2D gel than afternoon urine. The water-loading urine had the least amount of proteins by 2D-PAGE analysis, but exhibited a few newly presenting spots. There were more spots in the sample after caffeine ingestion than in water-loading urine.
To avoid bacterial contamination deriving from skin contamination, midstream urine is usually recommended as the standard for urinary proteome analysis, particularly for women [15]. Schaub et al. [16] employed surface-enhanced laser desorption/ionization time-of-flight mass spectrometry (SELDI-TOF-MS) in profiling the first-stream urine and midstream urine from three females and three males. For male samples, there were no observable differences between midstream and first-void urines. But for female samples, first-void urine emerged three specific SELDI peptide peaks after 3-day storage compared with midstream urine.
4.2.2 Protease Inhibitor
Protease inhibitors had been initially suggested to be used to prevent proteolysis of clinical biological fluids, which was caused by endogenous proteases [17]. However, whether protease inhibitors are necessary for the studies of urinary proteomics is debatable. Shinada et al. [18] incubated 30-kDa nonglycosylated [125I]IGFBP-3 with urinary samples and found IGFBP-3 proteolysis by SDS-PAGE analysis. But Havanapan et al. [17] studied the effect of protease inhibitors cocktail on midstream random urinary specimens and found that no observable qualitative and quantitative changes by two-dimensional gel electrophoresis (2-DE) analysis. Thongboonkerd [11] suggested protease inhibitors were unnecessary for the studies of nonproteinuric urine because there were lower amount of proteases in urine than in plasma, cells, or tissues.
4.2.3 Preservatives
During the storage, urinary samples might have bacterial overgrowth, which could change the urinary proteome [19]. Therefore, preservatives were recommended to prevent the bacterial overgrowth after collection [19]. Thongboonkerd and Saetun [19] studied the effects of the addition of either sodium azide (NaN3) or boric acid on bacterial overgrowth in pooled urine from 5 healthy individuals. They found the addition of NaN3 and boric acid could delay the bacterial overgrowth, and greater delay (for at least 48 h) was obtained by relatively higher preservatives. They recommended addition of 2–20 mM boric acid or 0.1–1 mM NaN3 to one-void random urinary specimens and addition of 200 mM boric acid or 10 mM NaN3 to 24-h urinary collections.
4.2.4 Storage Temperature
Appropriate storage temperature could decrease degradation of urinary proteins to some extent. In 1999, Klasen et al. [20] analyzed changes of albumin concentrations after urinary samples were stored at 4, −20, and −70 °C. They found that if samples could be analyzed in 4 weeks after collected, the best storage temperature was 4 °C. And they recommended −70 °C was used as storage temperature for longer storage. They did not suggest storage temperature −20 °C because they found the IgG concentrations decreased after 1-week storage at −20 °C. In 2007, Thongboonkerd and Saetun [19] reported that to prevent the bacterial overgrowth, uncentrifuged urinary samples without preservative should be kept no longer than 20 h at 4 °C. Without any preservative, urinary samples should not be stored at room temperature for longer than 8 h.
4.2.5 Freeze–Thaw Cycle
Previous studies showed freeze–thaw cycles could influence the components of body fluid proteome, including serum/plasma and cerebrospinal fluid [21, 22]. Studies also showed that when urinary samples were stored at low temperature, researchers should avoid freeze–thaw cycles [11]. Schaub et al. [16] analyzed first-void and midstream urinary specimens from three females and three males by SELDI-TOF MS. Results indicated that after 1–4 freeze–thaw cycles, the urinary proteome did not change remarkably except for the loss of intensity in some peaks, whereas some small peaks were undetectable after the fifth freeze–thaw cycle. Powell et al. [11] reported the degradations of some proteins resulted from 4 to 7 freeze–thaw cycles in urinary proteomics.
Furthermore, Klasen et al. [20] found some proteins forming precipitates after storage and thawing. According to the studies of Saetun et al. [23], after overnight storage at −20 °C, urinary proteins may precipitate, and they found that EDTA (5 mM) could reduce the amount of precipitates and pH could influence the type of precipitates. To redissolve the precipitates, effectively shaking of the specimens should be done at room temperature.
4.2.6 pH
Thongboonkerd et al. [24] analyzed adjusted pH urinary samples which were precipitated the proteins by 75 % ethanol. The 2-DE results showed different pH levels did not influence the consistency of individual urinary specimens and the total number of spots. Therefore, they thought it was unnecessary to adjust the pH of urinary samples before gel-based proteome analysis.
4.2.7 Standard Protocol for Urine Collection
Based on previous studies, a standard protocol for urinary collection was recommended by Human Kidney and Urine Proteome Project, HUKPP, and European Urine and Kidney Proteomics, EuroKUP Initiatives from 9 December 2009.
The details were described as follows:
Standard Protocol for Urinary Collection [25]
1.
Type of urinary sample
Midstream of second-morning urine (preferably) or morning random-catch urine, in sterile (preferably) or clean urinary collectors.
2.
Pre-treatment and storage
Centrifuge at 1,000g, for 10 min to remove cell debris and casts. Aliquot supernatant avoiding disturbing the pellets at 1.5, 10, or 50 mL (depending on downstream application); Do not overfill the tubes; Store at −80 (preferably) or −20 °C. Record time until freezing (it should be no longer than 3 h).
3.
Freezing and thawing
Avoid freeze–thaw cycles. If thawing and re-freezing occurs, always keep a record of this event.
Notes: http:/www.hukpp.org and www.eurokup.org.
4.3 Urinary Preparation
For urinary proteomics, crude urinary samples are complex including high concentrations of salts, small molecules, and some metabolic wastes [26], and concentration of urinary proteins is too low to be identified. So many sample preparation methods have been applied to concentrate urinary proteins and remove small molecules, such as organic solvent precipitation, ultracentrifugation, dialysis–lyophilization, and ultrafiltration (centrifugal filtration) [26, 27].
4.3.1 Organic Solvent Precipitation
Organic solvent precipitation is a popular method in urinary proteomics. Organic solvent can reduce of permittivity of urine and break hydration shell on the surface of protein molecules, and thus, urinary proteins are gathered and precipitated effectively. Organic solvent precipitation method had several characters. First, this approach can be used to handle larger volumes of urine and takes less time than other methods [27]. Second, it can enrich higher molecular weight proteins species than ultrafiltration [28]. Third, it can effectively precipitate more acidic and hydrophilic proteins than ultracentrifugation [6].
Studies showed types and concentration of organic solvents may play various roles on urinary protein precipitation [14, 27]. Khan et al. [27] employed 2-DE to analyze the urinary proteome precipitated by different organic solvents and found that higher resolution and more protein spots could be obtained using acetonitrile (urine-to-solvent ratio was 1:5). Thongboonkerd et al. [14] made a comprehensive comparison of different organic solvent precipitation methods by 2-DE. They revealed applying 90 or 75 % organic compounds could get greater recovery yield than lower percentage of organic compounds. Ethanol, methanol, or acetone precipitation methods were suggested to obtain more protein spots and higher protein recovery yield in routine or gel-based urinary proteome studies. Moreover, acetonitrile precipitation was suggested for proteinuric urine or a larger volume of urine for its highest number of protein spots but lower protein recovery yield.
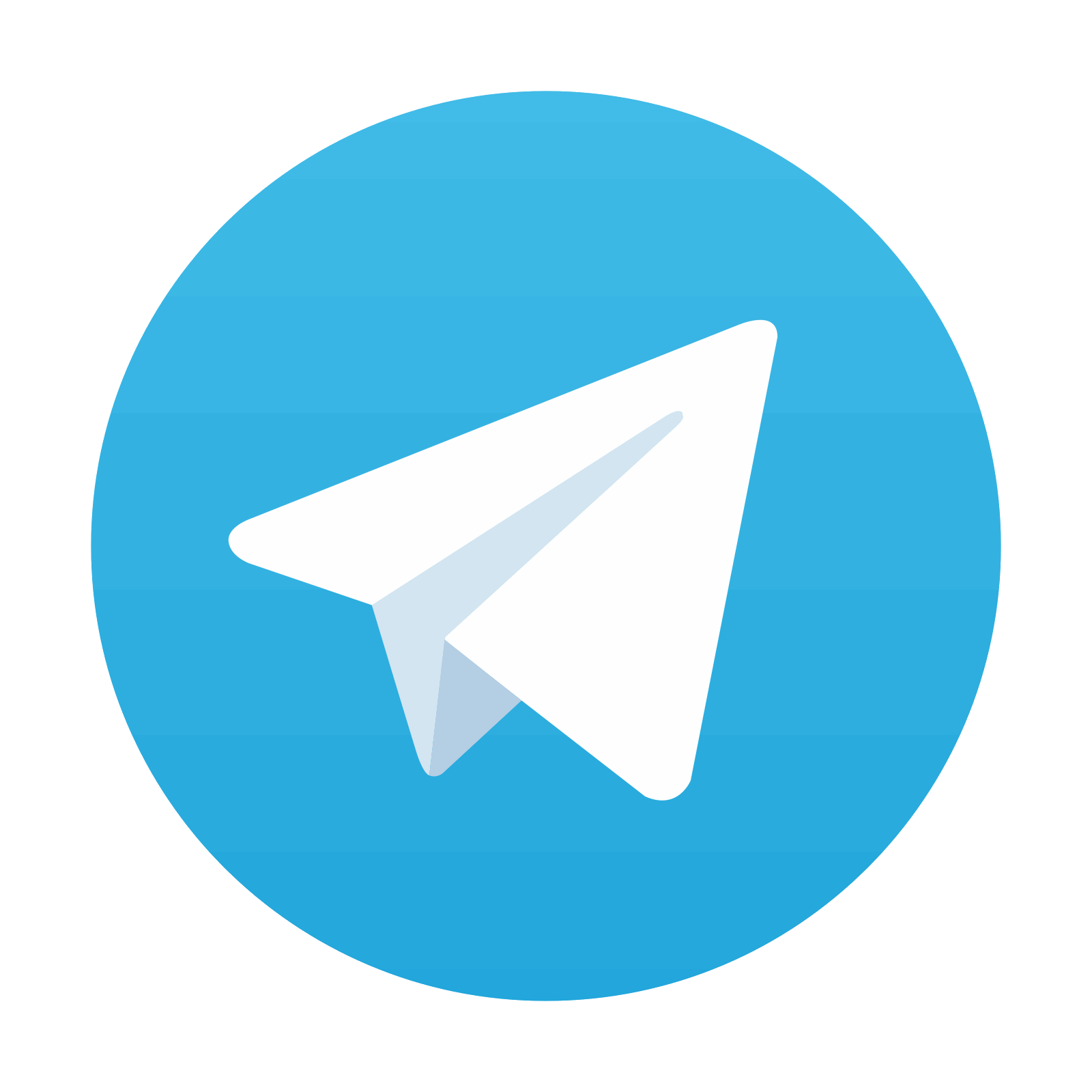
Stay updated, free articles. Join our Telegram channel

Full access? Get Clinical Tree
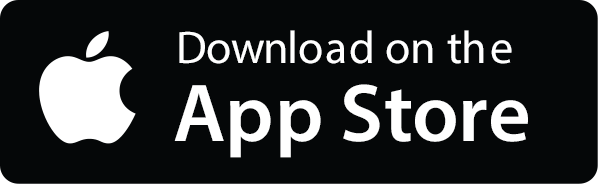
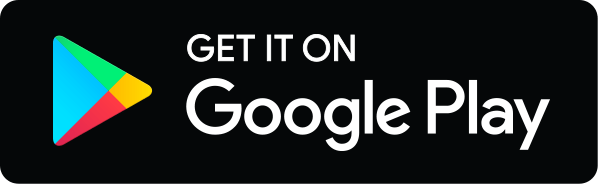