Abstract
Infections of the gastrointestinal tract (GIT) by pathogenic microorganisms such as bacteria, viruses, or protozoan parasites impose a huge burden on society. Despite intensive research to combat infections, increasing antibiotic resistance and newly emerging infectious diseases continue to pose a challenge in developing effective therapeutic interventions. This necessitates increased understanding of how these organisms alter host cell physiology to cause disease. Dissecting the complex and dynamic interactions between the host immune systems, epithelial responses and pathogens will more clearly elucidate the mechanisms of pathogenesis. Significant advances in our understanding of important aspects of host-pathogen interactions have been made during the last decades utilizing both in vivo animal models as well as recently using human organoid cultures. This chapter aims to highlight common human pathogens that infect the GIT with an emphasis on the microbial-epithelial interactions that may lead to the identification of novel drug targets to prevent or treat such infections and the associated consequences.
Keywords
Enteric pathogens, Host-pathogen interactions, Intestinal infections
64.1
Introduction
Gastric acid is one of the most important host defenses against infectious agents that enter the gastrointestinal tract (GIT). At pH < 3, gastric juice can kill bacteria within 15 min and hypochlorhydria (caused by drugs that inhibit acid secretion, atrophic gastritis, or gastric surgery) increases the risk of enteric infections. GI motility also provides host defense by reducing the adherence of pathogenic microbes to the GI mucosa. In addition to gastric acid and GI motility, there is a complex, multifaceted barrier that protects the underlying mucosa from noxious agents that enter the GI tract as well as provides a healthy separation between the intestinal microbiota and intestinal epithelial cells. This barrier includes a robust mucus layer secreted by both goblet cells and intestinal epithelial cells, antimicrobial peptides produced primarily by Paneth cells but also intestinal epithelial cells, IgA, the intestinal epithelial cells themselves, and intercellular tight junctions that protect the paracellular space. Further, the gut microbiota consisting of ~ 10 14 resident bacteria promotes resistance to infection by competing with pathogens for resources and limiting pathogenic bacterial colonization. Besides the presence of these protective mechanisms in the host, microbial pathogens have developed elaborate machinery to subvert host functions and cause disease. For instance, many pathogens harness the cytoskeleton to mediate their own uptake into host cells or utilize vesicular trafficking pathways to avoid antigen presentation and their delivery into harmful intracellular compartments. In the course of ensuring their own survival and transmission to new hosts, these pathogens often produce a series of undesirable outcomes. Of primary concern in the GIT is diarrhea with an estimated mortality rate of one million deaths in children < 5 years, especially in developing nations. Traditional diarrheal pathogens have also been associated with chronic disease in both developing and modernized countries. For example, repeated infections before age 2 with enteric pathogens are associated with a permanent reduction in both height and IQ and impact lifelong earning potential. Also, up to one-third of patients develop prolonged GI complaints following acute bacterial gastroenteritis and a subset of those acquire postinfectious irritable bowel syndrome (IBS). Thus, even transient infection with enteric pathogens may have profound and life-altering consequences requiring increased understanding of host-pathogen interactions. Diarrhea, which is characterized by an increase in water content, volume, or frequency of stools, generally occurs when there is either a decrease in fluid/electrolyte absorption or increase in secretion of water and electrolytes in response to an infection. The major epithelial processes contributing to pathophysiology of diarrhea include (i) perturbation of barrier function; (ii) a decrease in the function of Na + absorbing Na + /H + exchanger family isoform 3 and the Cl − absorbing SLC26A3 ( <SPAN role=presentation tabIndex=0 id=MathJax-Element-1-Frame class=MathJax style="POSITION: relative" data-mathml='Cl−/HCO3−’>Cl−/HCO3−Cl−/HCO3−
Cl − / HCO 3 −
exchanger, DRA), and/or (iii) activation of the chloride-secreting channel, the CFTR. The following sections address the pathophysiology of the common diarrheogenic pathogens that provide insight into potential host-based therapeutic interventions by increasing our understanding of pathogen-specific virulence factors and signaling cascades affecting a wide-range of host-cellular functions to elicit luminal fluid accumulation causing diarrhea.
This chapter will highlight host epithelial-microbial interactions of various diarrheogenic pathogens that are classified as below:
- I.
Enterotoxin-producing organisms: Vibrio cholerae and enterotoxigenic Escherichia coli or viruses such as Rotavirus.
- II.
Invasive organisms: Salmonella spp., Shigella spp., Campylobacter spp., Cryptosporidium parvum .
- III.
Inflammatory diarrhea: Cytotoxin-producing noninvasive bacteria, for example, Clostridium difficile , enterohemorrhagic E. coli .
- IV.
Other pathogens: Nontoxigenic enteropathogenic E. coli and Helicobacter pylori .
64.2
Enterotoxin-Producing Organisms
Enterotoxins affect host target cells in a robust and rapid way. The following sections will discuss host-microbial interactions of microorganisms that are adherent as well as toxigenic such as V. cholerae , some E. coli strains and rotaviruses. The major receptors of the toxins and mechanisms of their actions are summarized in Table 64.1 .
Toxin | Organism | Host Receptor | Mode of Action |
---|---|---|---|
Cholera toxin (CT) | V. cholerae | G M1 ganglioside | ↑ cAMP; ↑ CFTR; ↓ NHE3 |
Heat stable toxin (ST a ) | Enterotoxigenic E. coli (ETEC) | Gunaylate cyclase (GCC) | ↑ cGMP; ↑ cAMP; ↑ CFTR; ↓ NHE3 |
Heat-labile toxin LT I | ETEC | G M1 ganglioside | ↑ cAMP |
LT IIa LTIIB | ETEC | G D1a ganglioside G D1b ganglioside | ↑ cAMP ↑ cAMP |
NSP4 | Rotavirus | Integrins TLR2 | ↑ Ca 2 + ↑ TMEM16A; ↓ SGLT1 ↑ Serotonin; ↑ VIP |
64.2.1
V. cholerae
V. cholerae is the causative agent of cholera. Cholera is perhaps the most dramatic of the diarrheal diseases causing copious amounts of fluid production, primarily due to the presence of cholera toxin (CT). Extensive research over the past decades has resulted in CT being one of the best understood bacterial toxins.
64.2.1.1
Structure of Cholera Toxin
While V. cholerae secretes a number of toxins, the most widely recognized and perhaps the most significant in terms of disease is CT. Characterization of CT began in the 1960s. Initial experiments involved bacterial lysates or filtered culture supernatants of V. cholerae that were found to inhibit sodium uptake. During attempts to purify the active toxin, the authors inadvertently discovered the two-component nature of CT. Initial preparation and dialysis allowed for retention of one component in the dialysis bag and another component in the surrounding media. Neither component was functional alone but when combined the putative CT was reformed. In later studies, the same group utilized antibody against CT to identify the components of the toxin at a more purified level. They identified what was referred to as choleragen, a single active unit, and choleragenoid, an inactive five-component protein that also reacted to the antibody. Through further studies, including the ultimate crystallization of the toxin, it became clear that CT contains a single A subunit consisting of two peptides CTA 1 and CTA 2 (22 and 5 kDa, respectively) joined by a disulfide bond located centrally and 5 B subunits (CTB-11 kDa) arranged in a ring-like structure peripherally. The choleragen, previously described by Finkelstein et al., represents the intact CT while the inactive choleragenoid consists of the pentameric B subunit ring. The CTA subunit is itself the active portion of the molecule, specifically the CTA 1 peptide causing the disease phenotype. The CTB subunit binds to the host-epithelial receptor G M1 and in subsequent retrograde transmission through the Golgi, delivers CTA to target cells (discussed below). Currently, recombinant CTB (rCTB) is used as a component of an internationally licensed oral cholera vaccine, as the protein induces potent humoral immunity that neutralizes CT in the gut. Interestingly, recent studies have revealed antiinflammatory effects of CTB in vivo; CTB was shown to mitigate intestinal inflammation associated with Crohn’s disease in mice and humans.
64.2.1.2
Mode of Action of CT
64.2.1.2.1
Interaction of CT With Receptor and Entry Into Host Cytosol
64.2.1.2.1.1
CT Receptor
CT is released from V. cholerae and efficiently transported extracellularly by a bacterial type 2 secretion system. The V. cholera secretion system acts like a piston to extrude CT as well as hemagglutinin-protease (HAP) and chitinase into the extracellular space via a pore in the outer membrane. Upon release into the intestinal lumen, CT binds with high affinity and specificity to specific cell membrane receptors. While the ganglioside G M1 [Gal(β1-3)GalNac(β1-4)(NeuAc(α2-3)Gal(β1-4)Glc] → ceramide was identified many years ago as the receptor for CT, the absolute requirement for binding to this protein was only recently recognized. G M1 is a glycolipid topped by a branched complex of five sugars including a single sialic acid residue. Each member of the pentameric CTB subunit ring binds to the specific carbohydrate moiety of G M1 , leading to the interaction of the five subunits with five separate ganglioside G M1 molecules. Ganglioside G M1 clusters within detergent insoluble membrane microdomains (lipid raft fractions) of T84 cells and this localization appears to be necessary for toxin internalization as β-methyl-cyclodextrin, a cholesterol chelating agent, prevents CT-mediated Cl − secretion by blocking CT endocytosis. After binding to GM1, CT is endocytosed by the cell. The specific interaction of CT with G M1 is critical to toxin internalization. Enterotoxigenic E. coli produce a heat-labile enterotoxin known as LTIIb in which the A subunit functions identically to that of CT while the B subunit preferentially binds to ganglioside G D1a . Chimeras containing the A subunit of CT and the binding subunits of LTIIb do not cause a secretory response in T84 intestinal epithelial cells despite the availability of G D1a on the surface. Reciprocally, LTIIb, which is normally nonfunctional in T84 cells, could be rendered active as a chimera containing the A subunit of LTIIb and the B subunits of CT. In human intestinal epithelial cells, lipid raft-mediated endocytosis appears to be the preferred method of entry; however, in other cell types where caveolins are more abundant, caveolae can be utilized for toxin entry. However, it should be noted that these studies were carried out in adult intestinal lines and there is some evidence that there is a substantial difference between the uptake of CT by adult cells versus those of infants. The uptake of CT by fetal small intestinal cells (H4) is significantly greater than by T84 cells and endocytosis occurs through a clathrin-dependent process. Differentiation of these fetal cells using hydrocortisone reverses this phenotype by increasing the association of G M1 with lipid rafts and inducing internalization via caveolae.
64.2.1.2.1.2
CT Secretion in the Host Cytosol
After endocytosis, CT travels to the endoplasmic reticulum (ER) via a retrograde transport pathway ( Fig. 64.1 ). During the normal process of protein translation and movement outward to the Golgi, some proteins are specifically targeted for retention within the ER by a specific sequence (KDEL). If ER-targeted proteins escape the ER and end up in the Golgi, they are returned to their proper ER location through interaction of the KDEL sequence with an integral membrane protein called ERD2 which shuttles between the ER and Golgi. Because CTA possesses a KDEL sequence in the C-terminus, it resembles a lost ER protein and is promptly escorted to the ER by ERD2. While the KDEL motif enhances the process of retrograde transport, it is not entirely necessary for the process because KDEL mutants of CT are still able to elicit some Cl − secretion. After arriving in the ER, CT is subject to the actions of protein disulfide isomerase (PDI), which reduces the disulfide bond found between the CT A1 and A2 subunits and assists in the unfolding of the toxin ( Fig. 64.1 ). Much in the way that a space shuttle discards spent booster rockets, the CT A1 subunit can now leave behind the B and A2 subunits as they have served their purpose of binding and transport into the ER. The lone A1 peptide then coopts yet another host system, this time one designed to assist improperly folded proteins traffic from the ER to the cytosol so that they can reach the proteasome and be degraded. This system is referred to as ERAD- or ER-associated protein degradation. The ERAD system uses Sec61, which is normally thought of as an entry channel for newly synthesized proteins. Rather than allowing entry into the ER, the Sec61 complex in conjunction with ERAD allows for the secretion of the CT A1 peptide into the host cytosol. Recent studies utilizing mutant toxin and cell surface biotinylation approaches have shown that CT can in fact breach the intestinal barrier and cross the epithelial cells from the apical to the basolateral surface. These studies indicate an additional mechanism that bypasses retrograde transport to the TGN and enables transport of whole CT probably via a GM1-mediated transcytotic pathway.

64.2.1.2.2
Mechanisms of CT-Associated Diarrhea
64.2.1.2.2.1
cAMP and Secretory Responses
Soon after CT was purified, insights into its mechanism of action were revealed ( Fig. 64.1 ). CT entry into host cytosol and translocation to basolateral membrane allows for the characteristic interaction with Gαs, the regulatory GTPase of adenylate cyclase leading to inhibition of GTP hydrolysis and excessive production of cyclic AMP (cAMP). This step requires CTA1-mediated transfer of the ADP-ribose moiety of NAD + to an arginine residue (Arg 201 ) of an α subunit of Gαs. It is the overproduction of cAMP mediated by CT that triggers Cl − secretion and fluid imbalance associated with rice-water like diarrhea of V. cholerae . At the time CT was purified and identified, the nature of individual ion transporters and channels was not clearly understood. However, it was noted that addition of either cAMP or CT to rabbit ileal mucosa produced the same effect, an increase in Cl − secretion and a decrease in Na + absorption. Therefore, it was clear that alterations in cAMP levels were in fact responsible for the altered secretion resulting from V. cholerae infection. As additional information regarding individual ion channels and transporters was elucidated, a more detailed understanding of the mechanism of cAMP-induced Cl − secretion emerged. It is now known that the Cl − channel targeted by CT is CFTR (cystic fibrosis transmembrane conductance regulator). Upon release of cAMP, protein kinase A (PKA) is activated and phosphorylates several key serines in the regulatory domain of CFTR. Phosphorylation of the regulatory domain, along with ATP binding by the two nucleotide-binding domains, results in activation of the channel. The availability of Cl − for secretion by CFTR is driven by Na + /K + ATPase. The opposing action of the Na + /K + /2Cl − cotransporter (NKCC1), which transports K + , Na +, and 2 Cl − into the cell, and the Na + /K + ATPase, which actively secretes Na + and K + ions, results in an overabundance of Cl − within the cell. This excess Cl − is rectified through the secretion of Cl − ions. Besides the direct effect of CT on adenylate cyclase activity and cAMP production in enterocytes, CT also stimulates enterochromaffin cells to release the secretagogues serotonin and VIP.
64.2.1.2.2.2
Absorption
While the nature of the comparably smaller decrease in Na + absorption upon stimulation with CT has been largely ignored in favor of studying Cl − secretion, it is likely that downregulation of absorptive pathways such as epithelial sodium channel (ENaC) by CFTR is responsible for the decrease in Na + uptake. In this regard, activation of CFTR via cAMP results in a decrease in ENaC activity. Additionally, CT has been shown to inhibit both NHE2 and NHE3 activity in a cAMP- dependent manner with a more dramatic impact on NHE3. CT has also some effect on a number of aquaporins although they have not been shown to contribute to fluid accumulation in closed loops.
64.2.1.2.3
Human Enteroids as a Model System to Study CT-Associated Diarrhea
The development of a protocol for propagating primary cultures of mouse or human intestine to produce self- organizing mini-intestines, termed enteroids, has provided unique functional models for studying host-pathogen interactions and translating the findings to clinical scenarios. It is interesting to note that O blood group has been associated with more severe cholera infections. A recent study utilizing enteroids demonstrated a direct molecular link between blood group O expression and differential cellular responses to CT. These studies showed that in human enteroids expressing O blood group, CT stimulated robust cAMP relative to enteroids derived from blood group A individuals. In another study, exposure of proximal small intestinal human enteroids to CT-inhibited NHE3 and induced luminal dilatation that is blocked by CFTR inhibitors. Organoid-based functional swelling assays can provide preclinical models to aid in customized drug development and the evaluation of inhibitor potencies of drugs targeting pathogen-derived toxins.
64.2.1.3
Other Toxins of V. cholerae
While CT is well studied and key for altering host physiology during infection, a number of other less understood toxins appear capable of mediating a secretory response or perturbing barrier function that contribute to diarrhea. Because toxins are procured by acquisition of foreign DNA through phage, pathogenicity islands, plasmids, and other mobile genetic elements, not all V. cholerae strains express the same set of toxins. The toxins that affect ion secretion directly include accessory cholera toxin (ACE), which stimulates Ca 2 + -dependent Cl − secretion; V. cholerae cytolysin (VCC), which creates anion permeable pores and finally, NAG-stable toxin, which activates guanylyl cyclase, thus stimulating cGMP production and leading to PKG-mediated activation of CFTR. The V. cholerae toxins that alter intestinal barrier function include RTX and hemagglutinin/protease or HA/P.
64.2.1.3.1
Ace
Accessory cholera toxin (Ace) was initially discovered as a result of attempts to make a live, attenuated vaccine to prevent cholera. The vaccine strain JBK70 was created by deleting the genes encoding both the A and B subunits of CT. While this strain did not cause the severe diarrhea typically associated with cholera, many of the participants in the study developed mild diarrhea. This suggested that while CT may be the most potent factor contributing to diarrhea, other toxins exist within the parent El Tor strain. By exploring the cholera genome proximal to the CT gene, ctx , referred to as the core region, an additional toxin, Ace was identified. In a strain where the core region containing Ace and CT was deleted, Ace activity was studied by complementing with an Ace-expressing plasmid. Strains expressing Ace increased short circuit current ( I sc ), a measure of net ion movement, and fluid accumulation in a rabbit ileal loop model. In subsequent in vitro studies using cultured human colonic epithelial T 84 cells, purified Ace elicited a similar increase in I sc in a Ca 2 + —but not cAMP- or cGMP-dependent manner. The Ace-mediated increase in I sc was prevented by bumetanide or ouabain, inhibitors of the basally located Na + /K + /2Cl − transporter (NKCC1) and Na + /K + ATPase, respectively. Chelation of Ca 2 + with BAPTA-AM or addition of dantrolene, a Ca 2 + antagonist also inhibited the I sc response as did removal of Cl − or <SPAN role=presentation tabIndex=0 id=MathJax-Element-2-Frame class=MathJax style="POSITION: relative" data-mathml='HCO3−’>HCO3−HCO3−
HCO 3 −
from the Ringer’s solution in response to Ace. Involvement of a disodium 4,4′-diisothiocyanatostilbene-2,2′-disulfonate (DIDS)-sensitive apical Cl − channel was suspected because the alterations in I sc were dependent on Cl − and <SPAN role=presentation tabIndex=0 id=MathJax-Element-3-Frame class=MathJax style="POSITION: relative" data-mathml='HCO3−’>HCO3−HCO3−
HCO 3 −
. DIDS caused a 50% reduction in I sc suggesting that the secretion is mediated by the subsequently identified DIDS-sensitive Ca 2 + -activated Cl − channel (CaCC).
64.2.1.3.2
VCC
V. cholerae cytolysin (VCC) is a pore-forming toxin that is structurally related to α-toxin and leukocidin F from Staphylococcus aureus . While the S. aureus toxins are known to be heptamers, there is contradictory evidence regarding VCC. Initial biochemical experiments using silver stain suggested that the VCC complex was a pentamer, but subsequent electron microscopic studies suggest that the toxin forms a heptamer as seen for α-toxin and leukocidin-F. The VCC heptamer is believed to be an anion selective channel, in part due to its net positive charge. Bacteria initially secrete VCC in a procytolysin form that must be activated by a cellular protease. Recent studies suggest that the pro-VCC initially binds to the host cell membrane where it encounters ADAM-17, a cellular metalloproteinase that cleaves and thereby activates VCC, which then forms an oligomeric pore. In addition to alterations in ion permeability, VCC causes a dramatic vacuolation phenotype. The anion channel activity of VCC is required for vacuolation as DIDS and SITS (4-acetamido-4′-isothiocyanatostilbene-2,2′-disulfonic acid (disodium salt)) inhibition of the channel prevents this phenotype and cell death. The enlarged vacuoles have more recently been shown to occur as a result of autophagy. During the process of vacuole formation, VCC is internalized and associated with vacuolar membranes. While CT is clearly the most important factor in producing cholera-related diarrhea, VCC increases fluid accumulation in the rabbit ileal loop model and in suckling mice. In addition, VCC elicits a 20% increase in I sc from human colonic tissue.
64.2.1.4
Heat-Stable Enterotoxin
Heat-stable enterotoxin (NAG-ST or ST) is a 17 amino acid peptide originally characterized in a nonagglutinable (NAG) strain of V. cholerae , so-named because they are not reactive to anti-01 serovar antibodies. NAG-ST acts in a manner similar to E. coli toxin ST a . NAG-ST induces Ca 2 + release from intracellular stores in response to IP 3 , which activates PKC-α, leading to the activation of guanylyl cyclase and the production of cGMP. Most of the work performed with heat-stable toxin has been done with E. coli ST a or EAST-1 and, because of its homology, NAG-ST is presumed to act in a similar manner. The E. coli toxins ST a and EAST-1 are known to interact directly with membrane-associated guanylyl cyclase C (GC-C) and phosphorylation of guanylyl cyclase by PKC-α enhances its activation and production of cGMP. GC-C receptors mutated to alanine at Ser 1029 fail to respond to ST a upon the addition of PMA. Both the activation of PKC-α by NAG-ST and its homology to ST a suggest that the two toxins act by the same mechanism although a direct interaction of NAG-ST with guanylyl cyclase has not been demonstrated. Production of cGMP activates CFTR through either PKG-II or PKA-II, depending on cell type. Fluid accumulation and I sc in response to the guanylyl cyclase agonist ST a were reduced in the small intestine of PKG-II-knockout mice in comparison to control animals. Colon-derived cells such as T84 and Caco-2 also respond to ST a through activation of PKA-II.
64.2.1.4.1
RTX
RTX toxin, also referred to as VcRtxA, RtxA, or MARTX vc , elicits cell rounding and loss of epithelial barrier function. This is mediated in part through cross-linking of actin monomers, with a characteristic laddering of actin seen by Western blot. RTX is unusually large at 484 kDa, although only the 48 kDa actin cross-linking domain (ACD; aa 1913–2441) is necessary to mediate actin polymerization. Crystal structure and mass spectrometry of the cross-linked actin revealed residues K50 and E270 to be covalently bound through an isopeptide bond. Toxins lacking the ACD promote an intermediate phenotype of cell rounding without actin polymerization. This effect has been recently attributed to a Rho-inactivating domain (RID) between amino acids 2552–3099. The effect of RID on Rho, Rac, and Cdc42 is not permanent ruling out ADP-ribosylation or degradation and does not mimic GAP or phosphatase activity suggesting a novel mechanism of inactivation. The transepithelial electrical resistance (TER) of T84 monolayers drops significantly without a corresponding increase in I sc in response to RTX expressing V. cholerae while a RTX mutant strain does not alter TER. These studies were performed in a strain in which HA/P, Ace, and CT had been deleted. In addition, there was a 6.6-fold increase in the permeability of 3000 Da fluorescein-conjugated dextran following infection with the RTX-expressing strain. In addition to the ACD and RID regions of the protein, there is a cysteine protease domain (CPD) that is necessary for autoproteolytic activation of RTX. Interestingly, screens of a cysteine protease inhibitor library identified several candidate inhibitors in the aza-epoxide family that prevent actin cross-linking in human foreskin fibroblasts.
64.2.1.4.2
HA/P
Hemagglutinin/protease or HA/P cleaves a number of substrates including fibronectin, mucin, and lactoferrin in addition to the nick site necessary for activation of CT. There was a 90% drop in TER in T84 monolayers infected with a V. cholerae strain deleted for the core region of the CT-containing phage, which harbors CT, Ace, and RtxA (Bah-3). In contrast, an isogenic deletion mutant in hapA had no effect on TER. Complementation of this mutant fully restored the ability to disrupt barrier function. This work represents molecular confirmation of the earlier work by Wu et al., which showed that HA/P decreased TER in MDCK monolayers. A later study by the same group suggested that the loss of barrier function was due to both degradation of occludin and relocation of ZO-1. HA/P is an extracellular toxin and degrades occludin only at the two extracellular domains creating two antibody-reactive products of 35 and 50 kDa, which differ from those seen with trypsin degradation. The degradation of occludin was prevented by the zinc metalloprotease inhibitor Zincov as well. Changes in occludin were preceded by the disappearance of ZO-1 from tight junctions; however, ZO-1 was not degraded.
64.2.2
Vibrio parahemolyticus
Another member of the Vibrio family, V. parahemolyticus , is predominantly found in brackish coastal waters and causes a limited number of human infections per year. These infections are typically acquired through the consumption of raw oysters and during outbreaks harvest is postponed in heavily contaminated oyster beds until cooler waters, less favorable to V. parahemolyticus growth, arrive. Pathogenic V. parahemolyticus is characterized by production of thermostable direct hemolysin (TDH); however, it should be noted that < 1% of environmental isolates actually contain the TDH toxin. In addition to TDH, V. parahemolyticus produces another toxin known as TDH-related hemolysin (TRH). TRH is similar to TDH, but is incapable of creating the characteristic β-hemolysis on Wagatsuma agar, typically used for identifying pathogenic isolates of this species. TDH and TRH appear to be structurally and functionally similar and therefore the better-studied TDH will be used as an example.
There are several variants of the tdh gene that are associated with insertion elements within the V. parahemolyticus chromosome although tdh2 appears to be primarily responsible for the classic hemolytic phenotype. The structure of TDH is not well defined although the mature toxin functions as a dimer and contains an intramolecular disulfide bond between Cys 151 and Cys 161 . TDH activates PKC and raises intracellular calcium levels leading to a Cl − -mediated increase in I sc . The DIDS and Ca 2 + sensitivity of the subsequent Cl − secretion suggests involvement of the calcium-activated chloride channel (CaCC). Correspondingly, inhibition of CFTR with glibenclamide failed to alter the TDH-induced I sc response.
In addition to TDH and TRH, two type III secretion systems (T3SS) have been recognized in V. parahemolyticus . They reside on two different chromosomes and are labeled I and II accordingly. T3SS I affects cell rounding and tight junctions and will be discussed in the second half of this chapter while T3SS II has both cytotoxic and enterotoxic effects. Studies of this T3SS were only recently initiated; however; mutations that prevent delivery of translocated effector proteins such as VopT, also prevent cAMP stimulation and fluid accumulation in rabbit ileal loops. T3SS II had more of an impact than TDH in infected rabbit ileal loops. Loop inflation decreased 75% in a T3SS II mutant but was not changed by mutation of T3SS I. Thus, type 3 secreted effectors are important elements and perhaps even primary factors in V. parahemolyticus infections.
V. parahemolyticus also has an effect on tight junctions. TER decreased 80% by 3 h postinfection and caused extensive mislocalization of ZO-1 in a manner independent of TDH. While a specific mechanism for these tight junction changes has not been identified, one effector, VopS, has been shown to AMPylate the Rho family of GTPases, preventing their activity. The rapid loss in TER and ZO-1 phenotype are consistent with the inactivation of small G-proteins by TcdA and B. In addition, VopS expression in HeLa cells promoted cell rounding. VopS (Vp1686) is secreted by T3SS I and causes Rho family inhibition.
64.2.3
Enterotoxigenic E. coli
ETEC causes traveler’s secretory diarrhea characterized by massive intestinal fluid secretion similar to cholera that may range from mild to life threatening. The key virulence attributes of ETEC include adherence to epithelial cell surfaces by colonization factors and elaboration of heat-labile (LT) and heat-stable (ST a ) enterotoxins. Some strains of ETEC may also express enteroaggregative heat-stable toxin 1 (EAST1).
64.2.3.1
ST a and EAST1
ST a (heat-stable enterotoxin) and EAST1 (enteroaggregative E. coli heat-stable enterotoxin 1) are structurally and functionally similar toxins to the previously discussed NAG-ST of V. cholerae . EAST1 is found in a broader range of E. coli than the name suggests, but due to its functional similarity and high homology to the better-studied ST a , only ST a will be summarized here.
64.2.3.1.1
ST a Structure and Host Receptor
E. coli ST a consists of either 18 or 19 amino acids but only the residues between cysteines 5 and 17 are critical for toxicity. Crystal structures of this portion suggest that the molecule adopts a hairpin-like structure, stabilized by three intramolecular disulfide bonds. ST a enterotoxins bind to the extracellular domain of the receptor guanylyl cyclase C (GC-C) present in the brush-border membranes of the intestinal epithelium and renal proximal and distal tubules, as well as other cells and tissues. The natural ligands for GC-C are the natriuretic peptides guanylin and uroguanylin, although the binding affinity of these native ligands is substantially lower than that of ST a . GC-C −/− mice are protected from ST a -mediated secretion in the suckling mouse model.
64.2.3.1.2
Mode of Action of ST a
Binding of ST a to the GC-C receptor leads to the activation of the intracellular catalytic domain of GC-C that results in the formation of cGMP from GTP. Several studies have suggested that PKC-mediated phosphorylation of Ser 1029 of GC-C enhances the effect of ST a on cGMP production. While these studies utilized PMA to activate PKCs, it was later found that ST a itself activates PKC-α. Production of cGMP leads to activation of cGMP protein kinase II (PKG-II or cGKII). PKG-II-knockout mice had no increased fluid accumulation or I sc in response to ST a . PKG-II is myristolated, and therefore membrane associated, and directly phosphorylates CFTR. Studies utilizing various model systems, including the recently developed enteroids, showed that ST a enhances fluid secretion through CFTR. In addition, cGMP is known to inhibit phosphodiesterase 3 (PDE3), which hydrolyzes cAMP. Inhibition of PDE3 results in accumulation of cAMP which, in turn, activates protein kinase A, providing an additional PKGII-independent mechanism to stimulate Cl − secretion. cAMP and cGMP can trigger the movement of CFTR from intracellular vesicles to the cell membrane.
With respect to effects on absorption, the Na + /H + -exchanger (NHE) is another target of cGMP and PKA activation in intestinal epithelia. ST a was shown to inhibit Na + /H + exchange in jejunal enterocytes. A detailed analysis of the contribution of ion transport processes would benefit the modeling of disease pathogenesis and help elucidate the mechanisms of ST a -associated diarrhea. A number of other pathogens possess heat-stable enterotoxins including Yersinia enterocolitica and Klebsiella pneumoniae , although their mechanisms of action have been less well studied. From the therapeutic aspect, Linaclotide, a structural analog of ST a and GCC agonist, is an FDA approved drug used for treatment of IBS with constipation and chronic constipation. Although the mechanisms of Linaclotide have not been fully examined, CFTR activation has been suggested as one of the potential mechanisms modulating fluid homeostasis.
64.2.3.2
LTI and LTII
In addition to the heat-stable enterotoxins mentioned above, enterotoxigenic E. coli have two heat-labile enterotoxins called LTI and LTII. LTI and LTII resemble CT in both structure and function. LTI and LTII are each composed of an enzymatic A subunit and a pentameric B ring, which is responsible for binding to the host cell. The B ring of LTI like CT recognizes five separate molecules of ganglioside G M1 . LTII is divided into two subtypes with slightly different binding specificities. LTIIa recognizes ganglioside G D1b , while LTIIb recognizes ganglioside G D1a . These gangliosides differ only slightly in the branched sugar chain attached to the lipid backbone; however, this is sufficient to convey specificity. For example, LTI and LTII ADP-ribosylate the G αs subunit of adenylate cyclase elicit cAMP production in much the same way as CT. However, while CT interaction with G M1 mediates cAMP production in T84 cells, there is no cAMP production in response to LTIIb in these cells. In contrast, only LTIIb stimulates cAMP production in mouse Y1 adrenal cells, thus demonstrating the specificity of ganglioside binding. As is the case for CT, cAMP activates PKA which, in turn, phosphorylates CFTR leading to secretion of Cl − ions.
As is the case for CT, LTI and LTII undergo reverse trafficking through the trans Golgi network following receptor-mediated endocytosis. The ER-targeting sequence RDEL is present in LTI and LTIIa while LTIIB shares the KDEL sequence with CT. Reversing the RDEL sequence to LEDR reduced cAMP production by 60% in Caco-2 cells. While ER targeting enhances toxin activity, it is not entirely necessary as the toxin remains functional without the appropriate targeting sequence. The subsequent reduction of the disulfide bond linking the A1 and A2 peptides by the cellular enzyme PDI is better described for CT; however, it is reasonable to speculate that an equivalent process is responsible for the separation of the toxic A1 peptide from the A2 and B subunit binding and delivery portions. After being freed from the delivery portion of the molecule, the A1 subunit is secreted by Sec61 into the cytosol where it ADP-ribosylates G αs leading to activation of adenylate cyclase, cAMP production, activation of PKA, phosphorylation of CFTR, and Cl − secretion.
64.2.4
Rotavirus
Rotavirus is the leading cause of life-threatening diarrheal diseases among young children. Research over the past several years has provided important insights into mechanism of viral pathogenesis. Although, the mechanisms of rotavirus- induced diarrhea are slowly emerging, in terms of progress toward the containment of the infection, the rotavirus field has outpaced all others. Two recently developed oral vaccines are highly effective demonstrating a > 74% prevention of disease and an even greater prevention of severe disease. Both are live oral vaccines, but RV1 (Rotarix) is monovalent and based on strain isolated from Cincinnati, OH, which has been passed through cell culture lines numerous times resulting in an attenuated phenotype. RV5 (RotaTeq) contains five live reassortant viruses, which remix bovine and human rotaviruses such that the resulting strains have a mix of a capsid protein from one species and an attachment protein from the other preventing any of the viruses from being fully effective at infecting humans.
64.2.4.1
NSP4
Rotavirus primarily infects small intestinal villus cells and can cause watery diarrhea without significant intestinal inflammation. The double-stranded RNA genome of rotavirus encodes for six structural proteins that form virus particles (Vps) and six nonstructural proteins (NSPs). Nonstructural protein 4 (NSP4) of rotavirus is the only widely recognized viral enterotoxin. NSP4 is a transmembrane glycoprotein with a key role in the assembly of intermediate double-layered particles and their maturation to infectious virions within the infected cell. As a nonstructural protein, NSP4 is not included within the virion particle, but instead requires active infection and protein synthesis and is therefore consistent with the delayed onset of diarrhea in rotavirus infections. NSP4 is also actively secreted from virus- infected polarized epithelial cells after posttranslational modifications. The plasma membrane receptors that mediate the signaling pathways in response to exogenous NSP4 are still not fully identified. Recombinant NSP4 has been shown to bind to the metal ion-dependent adhesion site (MIDAS) motif present on integrins α1β1 and α2β1, which are mostly localized basolaterally. In this regard, mutations in NSP4 that reduce integrin binding correlated with an inability to cause diarrhea in mice. In addition to integrin receptors in intestinal epithelial cells, recent studies demonstrated that NSP4 elicits intracellular signaling in macrophages resulting in the secretion of inflammatory cytokines. These studies identified NSP4 as a novel agonist of a microbial pattern recognition receptor toll-like receptor 2 (TLR2) and a potential trigger of innate immunity to rotavirus infection in vivo.
64.2.4.2
Mechanisms of NSP4-Induced Diarrhea
NSP4 was originally discovered to produce diarrhea in neonatal mice during an attempt to produce antibodies against the NSP4 protein. Further characterization of this effect suggested that even a small 21 amino acid peptide of NSP4 is capable of inducing diarrhea in 6- to 7-day-old mice but not 18-day-old mice. While the nature of rotavirus-mediated diarrhea is known to be multifactorial, alterations in secretion and absorption are believed to account for the early onset of diarrhea that occurs prior to disruption of the villus structure. Whereas, the peptide nature of this toxin might suggest comparison with the heat-stable enterotoxins, it is believed to function in an entirely different manner. In the case of the heat-stable toxins, cGMP is produced resulting in the activation of CFTR. As a result, CFTR-knockout mice fail to respond to heat-stable enterotoxins; however, NSP4 causes diarrhea even in the absence of CFTR. NSP4 has-been shown to induce a transient increase in I sc that is believed to be mediated through a calcium-dependent pathway. The synthetic NSP4 peptide induces multiple prosecretory pathways that induce diarrhea including activating the recently identified Ca 2 + -activated Cl − channel TMEM16A and by inhibiting Na + absorption by the epithelial Na + channel ENaC and the Na + /glucose cotransporter SGLT1. Despite many years of research, there is no definitive identification of the transporters that are activated or inactivated with regard to chloride transport. However, focus on the theoretical transporters involved in this process has diminished in lieu of a search for alternative mechanisms. For example, a Cl − /H + symport has been proposed despite the generally accepted belief that Cl − and H + are moved through the coupled activity of DRA and NHE3. Recent studies utilizing human intestinal organoid cultures demonstrated that rotavirus antigen was found in enteroendocrine cells, suggesting the importance of serotonin production in rotavirus-induced diarrhea and pathogenesis. Drugs that block the action of the enteric nervous system (ENS) attenuate rotavirus-induced secretion in the intestine, suggesting a role for the ENS in the associated diarrhea.
64.2.4.3
Rotavirus and Barrier Function
NSP4 is the only known viral enterotoxin and, in addition to inducing ion secretion, it affects tight junction proteins as well as paracellular permeability. TER of MDCK monolayers dropped 75% after apical but not basolateral application of NSP4. A concentration of 1 nmol/50 μL was most effective as a 10-fold reduction in toxin concentration resulted in only a 25% drop in TER and an additional 10-fold reduction to 0.01 nmol/50 μL had no effect on TER. NSP4 also increased paracellular permeability to FITC-dextran by fivefold at 46 h. In addition, TER development was blocked with 20 μM NSP4 as was the early recruitment of ZO-1 into the lateral membrane of recently seeded cells. However, 20 μM NSP4 did not alter ZO-1 localization in established tight junctions. Similarly, disruption of ZO-1 occurred when cells were seeded in the presence of virus. Apical actin levels were also subtly decreased after 24 h of NSP4 treatment. While these effects were specifically attributed to the enterotoxin NSP4, additional effects on junctions were seen but not characterized in terms of the viral protein. By 10 h postinfection with an MOI of 10, claudin-1 was severely disrupted and redistributed to vesicle-like structures located within the cytoplasm. Breaks and loss of occludin occurred as early as 10 h postinfection with more severe disruption by 18 h.
64.3
Invasive Pathogens
The following section reviews invasive pathogens that most commonly cause acute bacterial enteritis in the United States including Shigella , Salmonella , and Campylobacter . The protozoan parasite Cryptosporidium species, the causative agent of cryptosporidiosis that is increasingly recognized as an alarming global health problem, is also highlighted. Table 64.2 summarizes important features of these pathogens critical for disease pathogenesis.
Organism | Disease | Symptoms | Virulence factors |
---|---|---|---|
Shigella flexeneri | Shigellosis | Bloody diarrhea Fever Abdominal pain | T3SS (IpaB) Shigella enterotoxin 1 (ShET1) Shigella enterotoxin 2 (ShET-2) SPATES (sipA, segA, pic) |
Salmonella enterica | Salmonellosis | Diarrhea Fever Abdominal cramps Nausea, vomiting | T3SS-1 (SPI1) T3SS-2 (SPI2) |
Cryptosporidium parvum | Cryptosporidiosis | Watery diarrhea Lack of appetite Nausea, vomiting Weight loss, fever Abdominal cramps | Excystation (proteases, peptidases) Adhesion (CSL, Gp-60/40/15, TRAP-C1) Invasion (phospholipases) Multiplication/survival (cysteine proteases, HSP70/90) |
Adherent invasive E. coli (AIEC) | Crohn’s disease | Inflammation | Type I pili, Flagella Outer membrane proteins (OMPs) |
64.3.1
Shigella
Shigella spp. are gram-negative pathogenic bacteria that cause Shigellosis , an acute intestinal infection, the symptoms of which range from mild watery diarrhea to severe inflammatory bacillary dysentery. Infection is caused by bacterial species from the Shigella genus ( S. dysenteriae , S. boydii , S. sonnei , and S. flexneri ). A subset of IBS patients develops postinfectious IBS after episodes of infection with S. dysenteriae or other pathogens such as Salmonella typhimurium or Trypanosoma cruzi . The bacteria are highly infectious with as few as 10–100 CFU capable of causing disease. As virulence factors, Shigella encodes a type 3 secretion system (T3SS) and other toxins encoded on the chromosome or virulence plasmid such as Shigella enterotoxin 1 (ShET-1), Shigella enterotoxin 2 (ShET-2), and Shiga toxin (Stx). ShET-1 and ShET-2 induce fluid secretion resulting in severe watery diarrhea. Shiga toxin (Stx) is an exotoxin produced by S. dysenteriae serotype 1 and certain serotypes of E. coli . Stx is cytotoxic for a variety of cell types and is responsible for the development of vascular lesions in the colon, kidney, and central nervous system. Extensive molecular studies on mechanisms underlying Shigella pathogenesis have been derived from studies of S. flexneri . These studies have led to identification of loci on the virulence plasmid encoding a T3SS and bacterial effectors required for invasion and modulation of the host immune response. This 31-kb region of a large virulence plasmid encodes many T3SS effector proteins, including IpaA, IpaB, IpaC, and IpgD, with IpaB being essential for the pathogenesis.
64.3.1.1
Host-Pathogen Interactions During Infection
During initiation of infection, S. flexneri rather than interacting with the apical surface, uses microfold cells (M cells) to induce its own uptake and then is transcytosed across the epithelial layer to reach the basolateral compartment. M-cells are specialized epithelial cells of the mucosa-associated lymphoid tissues that transport antigens from the lumen to immune cells. Their basolateral surface is invaginated to form a pocket-like structure to which macrophages and lymphocytes migrate. After transcytosis, S. flexneri encounter resident macrophages, where they rapidly induce apoptosis to ensure their survival. Macrophage cell death is associated with release of the proinflammatory cytokines interleukin-1β (IL-1β) and IL-18. Once released from the dying macrophage, S. flexneri invades intestinal epithelial cells from the basolateral side, escapes from the phagosome, and replicates in the cytoplasm ( Fig. 64.2 ). Cytoplasmic S. flexneri moves within the cell by directed polymerization of actin allowing the bacteria to spread to adjacent epithelial cells and avoid exposure to extracellular components of host immune defense. Cell-to-cell spread of Shigella requires E-cadherin (L-CAM) or N-cadherin. This was originally demonstrated utilizing two L-cell lines, one that expressed E-cadherin and another that did not. While cadherin-transfected cells showed the greatest ability to promote cell-to-cell spread, cadherin-positive cells that were infected and allowed to interact with cadherin-negative cells exhibited a reduced rate of cell-to-cell spread but higher than in cadherin-negative cells alone. This suggested that cadherin/cadherin binding was not necessary for cell-to-cell spread but enhanced this process. In addition to utilizing cadherins, a number of cytoskeletal components that typically support adherens junctions were found to be associated with traversing bacteria. These included α-actinin, vinculin, and α-, and β-catenins. Later studies showed that IpaC, a type III secreted effector molecule directly binds to β-catenin. Shigella infection leads to tyrosine phosphorylation of β-catenin causing alterations in E-cadherin association. By 6 h of infection, dissociation of the E-cadherin complex from α- and β-catenins was evident.

64.3.1.2
Pathophysiology: The Serine Protease Autotransporters of Enterobacteriaceae (SPATEs)
Similar to E. coli strains, S. flexeneri secretes enterotoxins belonging to a family of serine protease autotransporters of Enterobacteriaceae (SPATEs), including SigA, SepA, and Pic. SigA is homologous to the EAEC protein Pet and causes severe cytotoxic effects including cell detachment associated with rounding and loss of focal adhesions and actin stress fibers. SigA mutants cause 30% less fluid accumulation than wild-type strains in a rabbit ileal loop model. It should be noted that this decrease in fluid accumulation also occurs in a strain that harbors another known enterotoxin ShET1, suggesting that SigA plays a substantial role in this phenotype. SepA is the most abundant protein secreted into culture supernatant by S. flexneri . The proteolytic activity of SepA has been well characterized utilizing artificial peptide substrates; however, the natural substrate for SepA during infection has not been identified. The preferred substrates include Phe-Leu-Phe and Val-Pro-Phe peptides among others that are cleaved with varying degrees of success. The Pic protease (protease involved in colonization) is expressed by S. flexneri , enteroaggregative E. coli (EAEC), and uropathogenic E. coli (UPEC). Pic protease induces mucus release, cleaves mucin, and confers a subtle competitive advantage in mucosal colonization. Pic has a limited ability to cleave mucin as only purified Pic, but not Pic expressing strains, creates zones of clearing on agarose plates containing bovine or murine mucin. Recent studies showed that Pic efficiently cleaves mucin-like surface glycoproteins such as CD43, which is expressed on nearly all lineages of hematopoietic cells, including cells of the bone marrow, the thymus, and peripheral lymphoid tissues. Given the role of SPATES in immune modulation, a detailed understanding of their impact on host epithelial and immune cell function may aid in vaccine development against enteric pathogens.
64.3.1.3
Pathophysiology: Tight Junction Disruption by Shigella
Shigella alters tight junction structure and function. ML-7, a myosin light chain kinase (MLCK) inhibitor, prevented cell-to-cell spread of Shigella but not another intracellular pathogen, Listeria monocytogenes . In a later study, TER was shown to drop 70% by 90 min following infection with S. flexneri regardless of whether an invasive or noninvasive strain was used. Invasive Shigella reduced the amount of Triton X-100 insoluble claudin-1 found in apically infected T84 monolayers as early as 10 min postinfection, returning to control levels by 60 min. In contrast, noninvasive strains did not induce such a dramatic loss; however, levels of TritonX-100 insoluble claudin-1 were diminished by 10–30 min postinfection. Neither strain elicited this response when applied to the basal compartment. By 60 min postinfection, both invasive and noninvasive strains disrupted claudin-1 localization. As is the case for EPEC and EHEC infection, Shigella infection results in dephosphorylation of occludin and a corresponding loss from tight junctions and appearance in cytoplasmic vesicles. While ZO-1 was lost from TritonX-100 insoluble fraction, immunofluorescent staining of ZO-1 in infected cells was not altered compared to controls. However, the distribution of ZO-2 and E-cadherin in TritonX-100 soluble and insoluble fractions was altered. Interestingly, Shigella was visualized by immunofluorescence in the paracellular spaces of T84 cells, proximal to disruptions in ZO-1. These effects were reproducible among two different S. flexneri strains although a direct comparison of strain 5 to the previously described serotype 2a showed only a slightly reduced ability to decrease TER coupled with an inability to alteration claudin-1 distribution. However, changes in occludin, ZO-1, ZO-2, and E-cadherin were similar.
64.3.2
Salmonella enterica
The genus Salmonella is divided into two species, S. bongori and S. enterica , which include > 2400 serovars. Out of these, the nontyphoidal Salmonella (NTS) enterica serovar, such as Typhimurium (causing Salmonellosis) and the serovar Typhi (causative agent of typhoid fever) have served as important tools to study host-pathogen interactions. Compared to Shigella with low infective dose, Salmonella infection requires a larger innoculum to cause disease. In contrast to typhoid fever, which is common in the developing world, NTS Salmonelloses characterized by diarrhea, fever, and abdominal pain occurs worldwide. Three types of vaccines against S. typhi are currently commercially available. While there are vaccines against NTS serovars Enteritidis and Typhimurium that are effective in poultry, there are no vaccines available for NTS in humans or other animal reservoirs such as cattle or pigs. The following sections will discuss the current knowledge regarding the host-pathogen interactions of Salmonella enterica , increased understanding of which is important for future therapeutic and vaccine development.
64.3.2.1
Host-Pathogen Interactions During Infection
Salmonella , an invasive pathogen, replicates within host cells and creates a niche in membrane-bounded compartments called Salmonella -containing vacuoles (SCVs). Salmonella replicates in SCVs in both nonphagocytic epithelial cells and macrophages. Intravacuolar bacterial replication depends on tightly controlled interactions with host cell vesicular compartments. Salmonella encodes two distinct T3SSs on chromosomal Salmonella pathogenicity islands 1 and 2 (SPI1 and SPI2). The T3SS-1 translocates Salmonella effectors into the host cell to promote actin cytoskeletal modification and rearrangements to force bacterial internalization. The second T3SS, SPI-2, is necessary for maintenance of the integrity of the Salmonella -containing vacuole that is essential for intracellular growth in macrophages and systemic spread of the bacteria. T3SS-1 and -2 translocated effectors also trigger various host pathways to cause a massive efflux of electrolytes and water into intestinal lumen and manipulate the host innate and adaptive immune response.
64.3.2.2
Diarrhea Induced by Infection
Limited information is available on the mechanisms of fluid secretion in response to Salmonella infection. It has been proposed that increased inflammatory cytokine expression or increased epithelial proliferation may be partly responsible for influencing alterations in ion transporters in response to infection. For example, Salmonella has been shown to induce mediators of intestinal epithelial cell proliferation through specific inflammatory pathways that include production of interleukin-17/interleukin- 22, interleukin-18, and granulocyte-macrophage colony-stimulating factor and activation of the β-catenin/Wnt pathway. Also, various T3SS1 effectors, including SopA, SopB, SopD, SopE2, and SipA, are proposed to mediate the level of fluid secretion into the gut by their ability to induce inflammation. The following sections will highlight some of the studies demonstrating alterations in ion transport and tight junctions in response to infection.
64.3.2.2.1
Ion Transport Modulation
S. typhimurium -mediated fluid accumulation in a porcine ileal loop model was reported to be inhibited by indomethacin, a COX/PGE 2 inhibitor. Similarly, S. enterica has a similar increase in Cl − secretion due to twofold increase in Cox-2 levels and a threefold increase in PGE 2 levels as little as 2 h postinfection in human xenografts. Studies in Ussing chambers demonstrated reduced basal and/or adenosine 3′,5′-cyclic monophosphate-mediated electrogenic ion transport in Salmonella- infected mouse colonic tissues attributable to changes in Cl − or Na + transport. These effects were mediated, at least in part, by effector proteins secreted by the bacterial Salmonella pathogenicity island 1—and Salmonella pathogenicity island—2-encoded virulence systems. Infected tissue showed reduced expression of the chloride-bicarbonate exchanger (downregulated in adenoma) in surface colonic epithelial cells. CFTR was internalized in colonic crypt epithelial cells without a change in overall expression level. Expression of epithelial sodium channel β was reduced in distal colons of Salmonella -infected mice.
64.3.2.2.2
Alterations in Tight Junctions
S. enterica serovar Typhimurium infection results in a rapid loss of TER beginning as early as 60 min postinfection. At the same time, there is a marked accumulation of actin, ZO-1, and E-cadherin in a ring-like pattern below adherent bacteria, which tend to localize at cellular junctions rather than across the cell surface ( Fig. 64.2 ). In addition to alterations in TER and junctional protein localization, fence function is lost as shown by labeling apical membrane with BODIPY-sphingomyelin and subsequently infecting with Salmonella . Uninfected cells retain the BODIPY-sphingomyelin apically while infected cells allow passage into lateral membranes. Infected cells become notably smaller and contorted compared to adjacent uninfected cells suggesting contraction of the actomyosin ring. This contraction is prevented by the kinase inhibitor staurosporine; however, this inhibitor does not prevent alterations in fence function or TER. Instead, staurosporine enhances the effects of Salmonella on BODIPY migration to the lateral membrane, the drop in TER and increase in inulin flux. Salmonella produces at least two type III secreted effector molecules, SopE and SptP, that alter the regulation of members of the small G protein family. It has been hypothesized that small G-proteins such as Rac and Rho may contribute to the alterations in epithelial tight junction properties induced by Salmonella infection. The decrease in TER is prevented by GGTI, which inhibits geranylgeranylation of the small G-proteins, necessary for the appropriate membrane localization of active proteins. While this suggests that the small G-proteins are involved in barrier alterations, GGTI also blocks invasion of this strain of Salmonella (SL1344). The authors suggest that reduced invasion is not responsible for the protection of TER because lesser MOIs associated with a 50% invasion rate remained fully effective in dropping TER. Similar to previous studies from the in vivo model of Salmonella infection, recent studies in mouse organoids demonstrated a decreased expression of ZO-1 and occludin as well as an increase in claudin-2 in Salmonella- infected organoids. These studies also showed increased inflammatory responses such as activation of NF-κB and IL-8 in response to infection of organoids.
64.3.3
C. parvum
Cryptosporidium is a leading cause of waterborne disease among humans in the United States (CDC) and is also known to cause chronic diarrhea in patients with AIDS. In addition, Cryptosporidium infection can cause atypical manifestations in immunocompromized patients including biliary tract disease, respiratory tract disease, and pancreatitis. The Global Enteric Multicenter Study (GEMS), and most recently a global network study of malnutrition and enteric diseases (MAL-ED), reported this neglected parasite to be one of the four major enteric pathogens causing life-threatening diarrhea in infants and associated with growth stunting. The disease is transmitted via fecal-oral route following ingestion of the thick-walled oocysts present in contaminated water or food. The life cycle begins after the infectious sporozytes invade small intestinal epithelial cells and undergo asexual and sexual stages of propagation via a myriad of poorly understood interactions with the host cell. The putative virulence factors important for interactions of Cryptosporidium oocysts and sporozoites with host epithelial cells, including excystation, attachment, invasion, parasitophorous vacuole formation, and intracellular maintenance, have been characterized and extensively reviewed elsewhere. Initial attachment of the parasite to host cells occurs via mucin-like glycoproteins and thrombospondin-related adhesive proteins. Notably, the infective forms attach to the apical membrane of the epithelial cell, inducing a host-cell membrane protrusion that encapsulates the parasite in a parasitophorous vacuole, inside which the parasite undergoes asexual multiplication. The sporozoites then enter a sexual reproductive stage (female macrogamonts and male microgamonts) and after fertilization, the zygote develops into oocysts. The thick-walled oocysts are excreted into the environment but the thin-walled oocyst can auto-infect the host.
64.3.3.1
Pathophysiology of Cryptosporidia Diarrhea
In humans, cryptosporidiosis mainly involves infection of the jejunum and ileum resulting in the major phenotype of watery diarrhea lasting up to 2 weeks. Much of the understanding of cryptosporidia-associated diarrhea has been obtained from animal models (calf, neonatal pig, human xenograft animal models, primate and various neonatal or immunodeficient rodents) as well as in vitro intestinal epithelial cell culture models. Data from these models suggest villus atrophy, crypt hyperplasia, infiltration of the lamina propria, Cl − secretion, glucose malabsorption, and reduced barrier function as major contributing factors to cell damage and disease pathogenesis. Dysregulation of absorptive and secretory functions of the gut have been identified such as impaired glucose-stimulated Na + and H 2 O absorption and/or increased Cl − secretion in experimental models of cryptosporidiosis. The mechanisms that cause these alterations are not fully defined; however, it has been shown that intestinal epithelial cells respond to C. parvum infection with increased PGE 2 and PGF 2α production in piglet and human enterocyte models, which may contribute to increased fluid secretion.
64.3.3.2
Perturbation of Barrier Function During Cryptosporidium Infection
Besides the transport defects, perturbation in the barrier function of the intestinal epithelium also contributes to diarrhea caused by Cryptosporidium . Earlier studies in Caco-2 monolayers infected with C. parvum had increased permeability to molecules of 1000 Da but were impermeable to exogenously added or endogenously produced proteins of 1881 Da. C. parvum infection of the monolayers resulted in the release of cytoplasmic lactate dehydrogenase from the apical media, indicative of cellular injury. Recent electron microscopic studies using an organoid model of cryptosporiodosis revealed irregular stunting of microvilli, foci of indistinct tight junctions, and areas of dilated paracellular spaces. Malabsorption and abnormal intestinal permeability (decreased vitamin B 12 absorption, decreased d -xylose absorption, abnormal lactulose/mannitol permeability test) have also been demonstrated in persons with AIDS and cryptosporidiosis. Using a mouse model, Cryptosporidium has been shown to disrupt the tight junction proteins occludin, primarily by inducing redistribution to the intracellular vesicular pool. Increased claudin-2 expression, yet no change in ZO-1, was also noted in response to infection.
Intestinal cryptosporidiosis is also frequently associated with the loss of absorptive surface area. Using an HCT-8 cell culture model, early C. parvum infection was shown to inhibit host cell apoptosis as parasite development was initiated, while at later stages of infection apoptosis increased. Infected enterocytes have also been shown to exhibit necrotic changes. In a study using human volunteers infected with C. parvum , the expression of interleukin (IL)-1β and TNF in jejunal biopsies correlated with infection but not symptoms, while substance P levels correlated with the severity of intestinal disease. In a recent study, expression levels of IL-17 mRNA and Th17 associated cytokines were found to be significantly increased in the gut associated lymphoid tissue (GALT) and spleen during C. parvum infection of dexamethasone treated immunocompromized mice, suggesting that Th17 cells may be involved in immunity against Cryptosporidium infections. However, further studies in Th17-deficient animals are needed to confirm the relevance of the Th17 responses in cryptosporidium infections.
64.3.4
Campylobacter jejuni
C. jejuni is not as well characterized as other enteric pathogens despite the fact that it is nearly ubiquitous in chickens and represents a major food borne pathogen. C. jejuni -induced changes in tight junctions require days rather than hours. While this is longer than most pathogens, it is important to note that these bacteria were grown in a reduced oxygen environment (5% or less), conditions that are quite different than those encountered in cell culture. Similarly, early EHEC experiments required at least 15 h of infection before changes in bacterial culture conditions from LB broth to tissue culture media substantially decreased this number. Both invasive and noninvasive strains drop TER by 24–48 h postinfection although the invasive strain 81-176 was slightly more effective (–50%, 81%–176%, and − 36% NCTC 11168). The TER of T84 monolayers dropped in response to both apical and basolateral infections and correlated with the dephosphorylation and internalization of occludin. However, there were no apparent changes in ZO-1 levels or localization. Association of claudin-1 with lipid rafts increased while JAM-1 raft association was decreased without alteration in total protein levels. The mechanism underlying these changes has not been determined nor has the overall contribution of these changes to disease.
64.3.5
Adherent-Invasive E. coli
There is much interest in AIEC now that this E. coli pathotype is increasingly implicated in the pathogenesis of Crohn’s disease (CD). Although AIEC bacteria may colonize the intestinal mucosa of non-IBD patients, these bacteria typically do not penetrate a healthy mucosal barrier. Adhesion to intestinal epithelial cells is partly mediated by type 1 pili, which interact with the glycoprotein CEACAM6 in a mannose-associated manner, and by adhesin FimH. Interestingly, CEACAM6 is overexpressed in CD patients with ileal disease, making them more susceptible to colonization by AIEC. Gp96, an ER-localized stress response protein, is also overexpressed on the apical surface of ileal epithelial cells in patients with CD and can act as a host cell receptor for bacterial outer membrane vesicles (OMVs) promoting AIEC invasion. The intermediate filament vimentin, expressed on host mesenchymal cell surface, has also been suggested as a AIEC receptor. Given that vimentin interacts with the nucleotide binding oligomerization domain 2 (NOD2), host deficiencies frequently found in CD patients such as defects in autophagy related to NOD2, ATG16L1 and IRGM, have been shown to underlie increased AIEC invasion. Similarly, altered bile salt metabolism in CD patients has been suggested to promote long polar fimbriae expression permitting translocation through M cells. Besides M cells, bacterial translocation across epithelia can occur via alteration of intestinal tight junctions. AIEC decreases transepithelial resistance and causes loss of barrier function by inducing expression of the pore-forming protein claudin-2 and displacing ZO-1 and E-cadherin from apical junctions. AIEC are most effective at disrupting tight junctions when infecting from the basolateral domain. Basolateral infection of T84 monolayers decreased TER by 80% after 16 h while apical infection induced only a 46% drop. Permeability to Alexa-647 dextran 10 kDa increased 20-fold following basolateral infection of MDCK cells and correlated with regional loss of ZO-1 association with tight junctions. AIEC infection also induces the secretion of proinflammatory cytokines, such as TNF, IL-8, which can influence barrier function. Production of HIF-1α and activation of the classical NF-κB pathway has also been reported via binding of AIEC strain LF82 type 1 pili to CEACAM6 and flagella to TLR5 in intestinal epithelial cells. How AIEC modulate other host-epithelial responses to modulate ion absorption or secretion has not been studied.
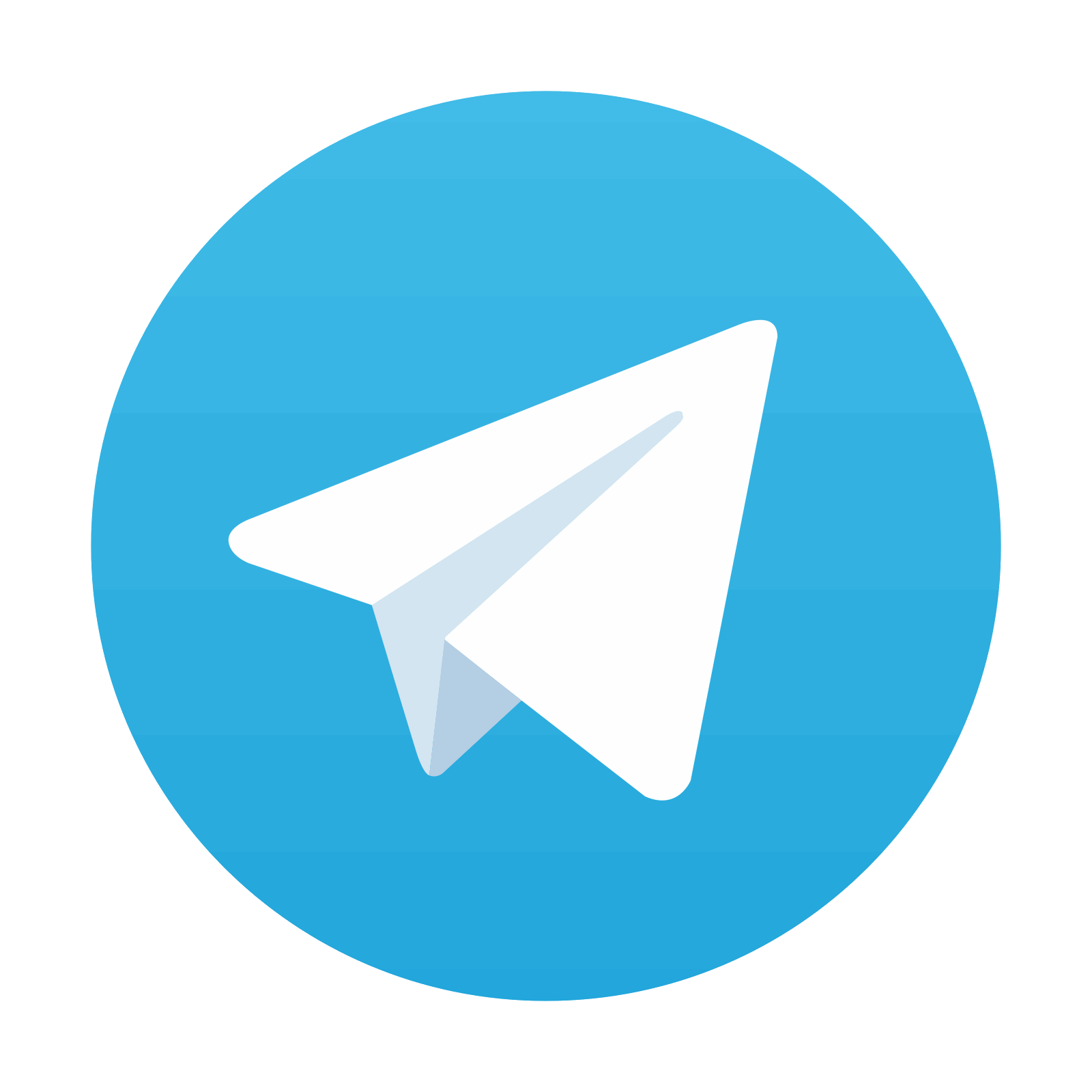
Stay updated, free articles. Join our Telegram channel

Full access? Get Clinical Tree
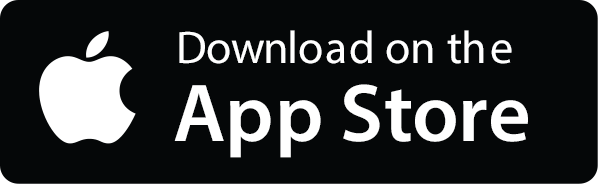
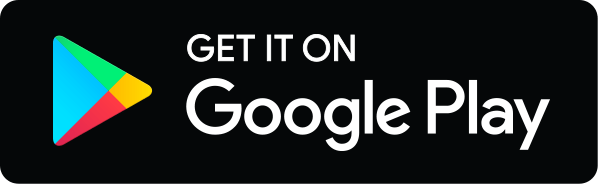