Fig. 3.1
Bozzini’s Lichleiter with one aperture for the user and one for light conductors (By Philipp Bozzini (1773–1809) [Public domain], via Wikimedia Commons; By Countincr at en.wikipedia (Transferred from en.wikipedia) [Public domain], from Wikimedia Commons)
Decades would pass before the literature revealed new forays into the endoscopy problem. Two physicians, Pierre Salomon Segalas and John Fischer, are generally given credit for simultaneously and independently improving Bozzini’s cystoscope. Segalas introduced his invention, the “urethro-cystique” in 1826, which incorporated a double lens system and mirror to improve the lighting and black coating on the viewing tube to reduce light scatter [4]. Fischer used the same principle as Segalas but used hollow tubing with two right-angled turns to form a Z-shape to visualize the urethra and bladder [5]. Like Bozzini’s lichtleiter, Segalas and Fischer’s inventions improved visualization but similarly failed to enable effective inspection of the bladder due to limited delivery of light.
The term ‘endoscope’ was coined in 1853 by French urologist Antonin Desormeaux. He reported the use of gazogène (a mixture of alcohol and turpentine) instead of a candle to illuminate his “l’endoscope” device based on Bozzini’s lichtleiter. This new light source provided a much brighter yet clearer flame than candlelight. He also adjusted the angle of his lenses to better focus the light, providing a clearer image [4]. Désormeaux used his device to perform the first endoscopic surgery, an endoscopic excision of a urethral papilloma, and is heralded as the “father of cystoscopy.” Unsurprisingly, the major complication of his procedure was burns [4]. Despite this improvement, Desormeaux’s endoscope was only able to inspect a very narrow field of view and still lacked sufficient illumination [6].
Second Generation Endoscopy: The Advent of Electricity, Intracorporeal Light Sources, and Increased Field of Vision
Advances in the techniques of illumination were intimately coupled with the discovery of electricity . Bozzini’s successors concentrated primarily on visualizing the urethra and bladder via speculum examination and an external light source. The next major breakthroughs came with German urologist Maximilian Nitze (Fig. 3.2). Nitze was uniquely credited with achieving a sweeping revolution in the diagnosis, treatment and photographic documentation via cystoscopy and is appropriately titled the father of modern urology [5, 7].


Fig. 3.2
(a) Maximilian Carl-Friedrich Nitze (1848–1906). (b) Prototype of Nitze’s cystoscope including the hot platinum filament at the tip (By Internet Archive Book Images [No restrictions], via Wikimedia Commons)
Nitze is credited with the first use of intracorporeal electric light sources. Aware of the limitations plaguing endoscopes of the time, Nitze is famously quoted stating that, “in order to light up a room one must carry the lamp inside” [7]. Nitze was inspired by the early works of Julius Bruck, a young dentist in Breslau, to overcome “insufficient illumination of objects” [8]. Bruck used incandescent platinum wires, commonly used for cautery in dental procedures, as a powerful light source contained in the endoscope to illuminate the distal most portion of the device [7, 9]. Nitze employed two optical technicians, Wilhelm Deicke and Louis Beneche of Berlin, to aid in the construction of the “Zystoskop,” a 21 French angled metal catheter with Bruck’s water-cooled platinum wire functioning as an intracorporeal light source at the tip. The first demonstrated use on a patent occurred on December 21, 1877 [7].
Nitze made a second revolutionary contribution to the advancement of endoscopy, recognizing that the existing cystoscopes were also plagued by “minuteness of the field of vision” [8]. In 1879, Nitze paired with Viennese surgical instrument maker Joseph Leiter. The Nitze-Leiter cystoscope was augmented with prisms and lenses allowing an unprecedented transurethral visualization of the bladder with a field of vision greater than the size of the aperture and scope [4]. Although functional by allowing much greater visualization than previous endoscopes, the complicated bulky water-cooled wire apparatus was difficulty to insert and cumbersome to use; the entire device caused heat burns to patients and had a prohibitively expensive price [7, 10].
Platinum wires had limited success as light sources but importantly served as the basis for electrocautery, transforming endoscopy from a mere diagnostic tool to a therapeutic one as well [4]. Nitze and Leiter reported a cystoscope devoid of the bulky cooling system in 1880. The primacy of this internal light source invention was strongly contested and eventually rewarded to Parisian engineer Gustave Trouve for his 1873 invention. Trouve’s distal light source was made out of thin platinum filaments (one-fourteenth to one-sixteenth inch thick) that produced little heat [4].
Third-Generation Endoscopy: Cold Light Sources, Non-Inverted Images, and Upper Urinary Tract Visualization
The 1878 development of the incandescent light bulb simultaneously by Joseph Swan in England and Thomas Edison in the United States heralded the advent and ultimately widespread use of cystoscopes free of cooling systems, called cold light sources. In 1883, David Newman of Glasgow was the first to place an incandescent light bulb at the end of his cystoscope. This provided a safer light source with fewer patient burns [11]. Nitze incorporated the incandescent light bulb into the tip of his cystoscope in 1887 (Fig. 3.2). This addition instantly transformed the Nitze cystoscope into a simple, inexpensive instrument. Newer models designed by Nitze, Hartwig in Berlin and Leiter in Vienna had larger visual fields with a thinner design, becoming the forerunners of modern cystoscopes. Illumination and device size further improved with the mignon lamp, a “cold” or low-amperage lamp, invented by electrician Charles Preston in 1898 in New York. The mignon lamp was a smaller, more reliable, less expensive and non-heat generating vacuum lamp [5].
At the heels of Preston’s invention, American physicians also had significant contributions to the lens systems and field of view. An established need for more efficient repair options drove the production of American endoscopic instruments. In 1893, Howard Kelly at Johns Hopkins manufactured the first American-made, direct view, air-distension cystoscope, which became the American standard for many years [12]. Reinhold Wappler, William Otis and opticians Bausch & Lomb created a spherical prism optical system in 1902, allowing wider angle viewing [5]. Improvements in the “Wappler Brilliant Lens System ,” a hemispheric lens implanted into the cystoscope tip in 1905, created an image four times larger and permitted visualization of the entire bladder, a dramatic improvement in the field of vision.
Cystoscopes historically had a direct-vision axis that produced inverted or mirrored images of the anatomy requiring significant surgeon skill to operate. In 1906, the Amici prism was developed by the Swiss Zeiss Company; it used an additional prism to produce a double reflection and a true “right-side-up” image. This advancement was widely accepted in Europe but the concept of an additional prism and subsequent upright image was slow to gain widespread acceptance in America. The first documented use was in 1908 by Leo Berger of New York.
With improvement in cystoscopes, the upper urinary tract was the logical next frontier. Initially pediatric cystoscopes were used as the first rigid rod-lens ureteroscopes. Hugh Hampton Young first reported transurethral visualization of the ureter in 1912 when passing a pediatric cystoscope into a massively dilated upper urinary collecting system in a 2-week old infant with posterior urethral valves [13]. Ureteroscopy was not reported again until 1977 by Tobias Goodman with the use of an pediatric cystoscope to visualize the distal ureter in three adult patients [14]. Access to the ureter was primarily limited by the length of the instruments and secondarily limited by the size of the instruments. Ureteroscopy in men was further hampered by the male urethra and prostate limiting manipulation of the ureteroscope. Edward Lyon reported transurethral ureteroscopy in men in 1979 [15]. In 1980 the entire ureter, renal pelvis and upper calyces were visualized using a long rigid ureteroscope by Drs. Pérez-Castro and Martínez-Piñeiro [13, 16].
Fourth-Generation Endoscopy: The Evolution of Flexible Optics
Designed to better navigate anatomical curves, the evolution of flexible endoscopy paralleled the development of rigid instruments. The use of bent glass rods to illuminate body cavities dates back to Roth and Reuss of Vienna in 1888, and a bent glass rod surgical lamp was patented by David D Smith a decade later [17]. Regardless of shape and size, glass transmits light due to internal reflection. Although possessing the same chemical properties, stretching glass rods into fibers decreases the diameter which bestows new physical properties upon the glass fibers. The bundle of glass filaments also transmits light but exhibits added flexibility and strength when compared to the corresponding diameter glass rod.
As a medical student in 1930, Heinrich Lamm showed that the fibers could be bent without effects on light transmission. This illumination technique is still used in flexible ureteronephroscopes today. Lamm used a bundle of glass optical fibers to carry an image of a light bulb, but his patent application was rejected due to poor image quality and an existing British patent for image transmission by Clarence Hansell; even his professor thought his work was a failure so Lamm independently published his findings. Lamm and Hansell also independently documented that clear image transmission requires accurate spatial mapping with fibers aligned at the same point at each end [17].
Early efforts with bare glass fibers resulted in poor image quality and were subject to significant light loss during transmission. As described by Henry C Saint-René in 1895, each glass fiber in a bundle transmits an image point by point such that “the whole array gives a complete illusion of the object,” thus many small fibers are needed to show details. Physicist Harold Hopkins,—inventor of the zoom lens in 1948—and graduate student Narinder Kapany improved image resolution by increasing the number of fibers in a bundle [18]. Imperfect fiber surfaces and light leaking between fibers interfered with internal light reflection down the long axis of the bare fibers. Optical physicists Brian O’Brien and Abraham van Heel suggested coating a fiber with low refractive index material to maintain total internal reflection and also protect the optical surface at the cost of added complexity and reduction in light collection (Fig. 3.3). Van Heel concentrated on developing transparent coatings to improve light transmission. Both Hopkins and van Heel independently published their work in the same Nature edition in 1954; together the two papers launched fiber optics. In 1956, based on van Heel’s work, undergraduate Larry Curtiss created glass fibers coated with an extra layer of glass with a lower refractive index in order to achieve better total internal reflection and reduce the amount of light lost in transmission [17]. The glass-clad fiber was the last piece needed to develop the fiberoptic endoscope, which was first used by Dr. Basil Hirschowitz, a gastroenterologist who tested the prototype on himself [19]. Glass-clad fibers are still used today.


Fig. 3.3
Enlarged view of optical glass fiber coated with lower reflective index glass cladding illustrating total internal reflection of light (User A1 at the English language Wikipedia [GFDL (http://www.gnu.org/copyleft/fdl.html) or CC-BY-SA-3.0 (http://creativecommons.org/licenses/by-sa/3.0/)], via Wikimedia Commons)
In 1959, Hopkins revolutionized the lens systems by reversing the standard lens configuration. Previously the lens system consisted of a tube of air with a succession of thin glass lenses; the new Hopkins lens system consisted of a tube of glass with a succession of thin lenses of air [20] (Fig. 3.4). A significant increase in light transmission and image quality was achieved by the increased refractive properties of glass over air and maximized lens size for the outer diameter due to mechanical lens mounting. Additional use of different multilayer anti-reflection coatings on the lens surface improved the light transmission to 80 times greater than traditional systems of the same diameter [5, 9, 18].


Fig. 3.4
(a) Previous lens system with air tube and succession of thin glass lenses. (b) Hopkins lens system with glass tube and succession of small air lenses
Unfortunately, English and American companies displayed little interest in Hopkins’ invention but his steely determination continued to drive his work. In 1965, Karl Storz, a German manufacturer of precision instruments, recognized the potential of the Hopkins rod-lens system and production of instruments using this technological advance began in 1967. Storz abandoned the rigid terminal cystoscopic barrel and replaced this with his fiber optic illumination [18, 21]. The Hopkins-Storz endoscope reinvented useful glass-fiber optics by creating a smaller overall diameter endoscope with brighter images due to better light transmission, increased image sharpness, wider viewing angle, improved contrast and color rendering, and outstanding resolution [21]. The Hopkins-Storz endoscope effectively eliminated the nearly 200-year-old problem of insufficient illumination. After production began in 1960, the new illumination system spread rapidly worldwide and the same principle exists in nearly all modern cystoscopes [9].
Fiberoptic endoscopy was first documented in the urinary tract in 1962 by John McGovern and Myron Walzak [22]. Dr. Tadanobu Takagi used a fiberscope to visualize the upper urinary collecting system in 1968 [23]. The far-sighted investigations of fiber optic ureteroscopy by Marshall, Bush and Whitmore as well as Tsuchida and Sagawara were not followed up [22, 24, 25]. There seemed no obvious place in urology for fiberscopes with their necessarily poorer image quality and limited operative potential.
In the early 1980s technical improvements in the fiberscope construction decreased the caliber of the instruments, increased the overall length, and rekindled clinical interest. Wilbur, Burchardt and Wagenknecht demonstrated some of the many uses in urology for a choledochoscope with a 5 mm diameter [26–28]. Despite limitations on optical resolution from the coating and packing of glass fibers, Fowler and colleagues in London and subsequently Clayman and colleagues in the United States showed that the image quality of the flexible cystoscope was sufficient to demonstrate lesions in the bladder with similar diagnostic accuracy as rigid cystoscopy [29, 30]. Small caliber fiberscopes adapted to the serpentine anatomy of the male urethra such that only topical anesthesia was required to minimize discomfort. Advantages to all patients in terms of comfort and convenience were immediately evident. The major stimulus for clinical use stemmed from the idea that flexible cystoscopes would allow pain-free diagnostic cystoscopy under topical anesthesia in the office or outpatient clinic [31].
Fifth-Generation Endoscopy: Digital Endoscopes
Fiberoptic endoscopes were limited by the insurmountable finite diameter of image-carrying glass fibers and subsequent pixelated images, as well as the excessively bulky cameras required to record images. These challenges were overcome with distal video chip sensor technology, which functions as a miniature camera using a charge-coupling device (CCD) image sensor or complementary metal oxide sensor (CMOS) . CCD and CMOS chips are sensor arrays that allow pixel conversion of incoming light photons, including color accuracy, into electrical charge and ultimately to a digital form. CCD chips were originally designed at AT&T Bell Labs by Willard Boyle and George E Smith [32, 33]; they were awarded the Nobel Prize for Physics in 2009 for their invention. CCD chips produce a very high quality image instantly at a high cost. CMOS chips offer a reduction in cost and size thanks to fewer electronic components requirements and lower energy consumption at the expense of image quality. The first electronic video endoscope was manufactured in 1983 [34]. Today, both CCDs and CMOSs are used in digital video flexible cystoscopes, ureteroscopes and nephroscopes [35–37]. Superior optical fidelity is hypothesized to result in superior surgical performance [6].
The latest developments in scope technology have enabled newer optical technologies to be adapted into endoscopy. Though traditional cystoscopy has relied on the use of visible “white” light since the days of Bozzini and his lichtleiter, modern medical optics seek to harness and refine light to accomplish tasks not achievable with white light illumination. The development of the CCD has allowed the high-definition processing of a variety of light sources, from infra-red to ultraviolet and across the spectrum of visible light. The result is that the frontiers of endoscopy lie in using light to study tissues and processes in ways heretofore impossible. The next few sections will review some of these newer optical techniques being used in endourology.
Narrow Band Imaging
Narrow Band Imaging (NBI) is an imaging technology that has recently been employed in endoscopic imaging to improve detection of malignant tissues. First used medically in the early 2000s for the endoscopic identification of bronchial and gastrointestinal tumors, this optical technology uses a CCD chip and a special filter to enhance the visualization of light of 415 and 540 nm bandwidths, which correspond to the green and blue light most readily absorbed by hemoglobin [38, 39]. This technology has been particularly useful in the cystoscopic detection of bladder cancers, which tend to be hypervascular. While the identification of small tumors can be challenging with traditional white light cystoscopy, the option to “flip a switch” on the scope to turn on NBI to make tumors more visible has been appealing. Another advantage of this technology is that, instead of relying on an injectable or topical contrast agent, NBI harnesses the innate properties of hemoglobin itself to increase contrast in the image and increase the visibility of cancerous tissues.
Cystoscopy with NBI to evaluate for bladder tumors was first reported in 2008, and the favorable results of these and other preliminary investigations fostered interest in further research of this technology in bladder cancer. A recent multi-center prospective randomized controlled trial conducted by the Clinical Research Office of the Endourological Society (CROES) enrolled 965 patients and found that bladder lesions were significantly more visible with NBI than standard white light (p = 0.033) [40, 41]. While the study found no difference in overall recurrence at 12-month follow-up between NBI-assisted bladder tumor resection and standard white light, there was a lower risk of recurrence in low-risk patients with NBI (0% vs 15.1%; p = 0.006). This same optical technology has been used to diagnose and treat upper tract urothelial cancers, though data is limited. Traxer and colleages reported a series of 27 digital flexible ureteroscopies performed for upper tract urothelial cancers using both white light and NBI imaging [42]. Similar to the bladder, NBI was found to make tumors more obvious but also allowed detection of additional tumors or extended limits of tumors unseen by white light endoscopy in 22.7% of patients.
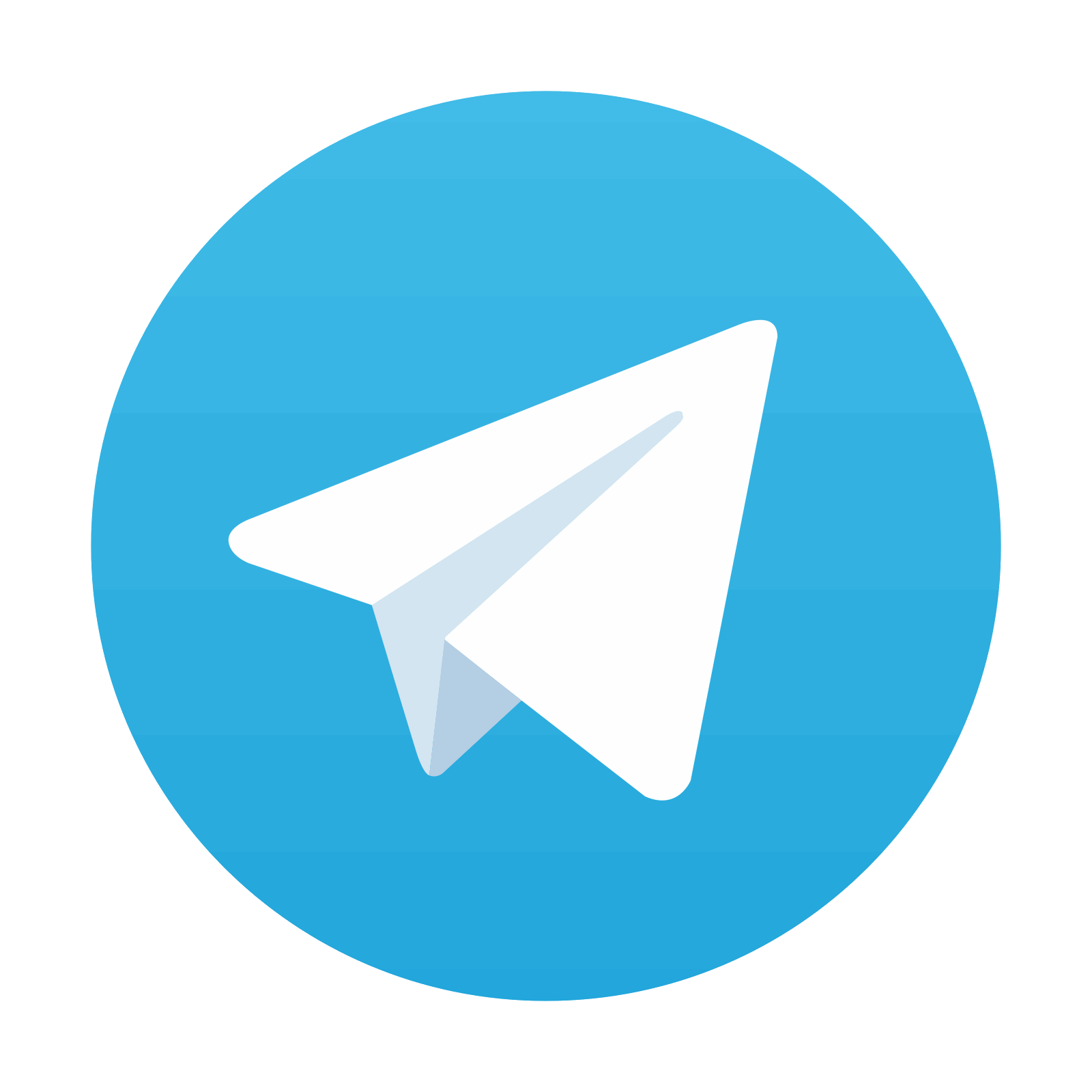
Stay updated, free articles. Join our Telegram channel

Full access? Get Clinical Tree
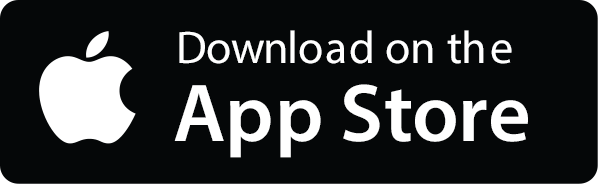
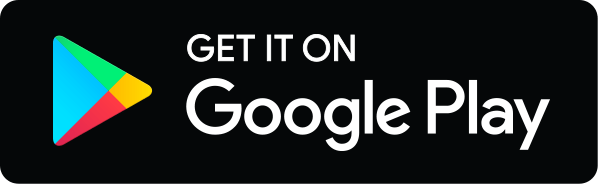