(1)
Department of Surgery, Division of Vascular Surgery, Duke University Medical Center, Durham, NC 27710, USA
(2)
Humacyte Incorporated, Research Triangle Park, NC 27709, USA
(3)
Department of Surgery, Duke University Medical Center, Durham, NC 27710, USA
Keywords
Arteriovenous graftDialysis accessHemodialysisPTFEVascular prosthesisIntroduction and Historical Perspective
Vascular surgery was forever changed – and the field of dialysis access established – with the advent of the Scribner shunt at the University of Washington in 1960 [1]. This external, Teflon tube is attached to the arterial and venous circulation (in the forearm or ankle) and then joined together in the “U” configuration by connectors and a piece of heparinized Teflon, while the patient is not actively being dialyzed. Later in the same decade, Drs. Brescia, Cimino, and Appell conceptualized the first autogenous, or non-synthetic, dialysis access in the form of the connection of the cephalic vein to the radial artery (radiocephalic arteriovenous fistula) [2].
In 1969, just 2 years after the clinical implementation of the arteriovenous fistula (AVF), the first autologous graft was used for the creation of an arteriovenous access almost simultaneously in both Mexico and Australia [3, 4]. Flores Izquierdo and May described the use of the saphenous vein for the creation of a forearm loop arteriovenous graft (AVG). May et al. observed that there was a group of patients in which both the suitable artery and the vein were lacking for the creation of an AVF, and an interposed conduit was required to provide an area of blood flow and access for hemodialysis.
These seminal events led to much excitement in the field of access creation and hence the further development and use of other materials, such as expanded polytetrafluoroethylene (ePTFE) and Dacron (polyethylene terephthalate) [5, 6] (Fig. 18.1). In the 1970s the use of ePTFE was pioneered as a suitable vascular graft and was rapidly adopted as an alternative material for connecting arteries and veins in an array of configurations around the body [7]. This fundamental advance of using an interposed synthetic tube to provide blood flow superficially beneath the skin for hemodialysis access has been a mainstay of access for millions of patients and can often be a life-sustaining solution for those patients whose, for an array of reasons, creation of a native AVF is not possible.


Fig. 18.1
Top: ePTFE histology from first conduits used for human arterial replacement circa 1970 (Johnson, Goldfarb, et al). Bottom: en bloc explants of Dacron grafts from dogs in an AV access model
The conception of prosthetic grafts revolutionized vascular access and gave rise to a number of new access sites that were previously unavailable when creation of an AVF was the sole option. However, this new type of vascular access presented a whole new set of challenges and complications, driving expenditures for dialysis access to another level. Today the health and social realities of ESRD are tremendous, with economic costs in the USA estimated at more than 2.9 billion dollars to maintain malfunction dialysis access [8]. While dialysis grafts have provided reliable access for millions of patients in need of hemodialysis, they are still far from ideal. Currently, regardless of the material used for an artificial AVG, their mean patency remains generally poor averaging between 9 and 15 months, and infection rates are greater than AVFs [9–13]. Further, enduring patency often requires multiple interventions including mechanical thrombectomy, thrombolysis, angioplasty, stent placement, and/or surgical revision. These interventions are fraught with recurrent failure, cost millions of healthcare dollars, and expose the patient to increased morbidity and mortality [8].
In patients lacking suitable vein for conduit, ePTFE is the most commonly used synthetic solution for creation of arteriovenous access. There are multiple modes of failure that plague prosthetic vascular access grafts including neointimal hyperplasia in the outflow vein, thrombosis, infection, graft ultrafiltration (weeping), steal syndrome, and traumatic degeneration of graft material [14]. Further, synthetic conduit has several biologic challenges. Specifically, because the conduit lacks the ability to form a stable endothelium, it appears to be more thrombogenic. Because it is impermeable to white blood cells, synthetic material is more prone to infection, which may lead to the need for surgical excision. Additionally, due to mechanisms that are incompletely understood, the body’s response to synthetic material can result in venous neointimal hyperplasia leading to venous outflow stenosis. Progressive outflow stenosis increases the pressure in the access and decreases the flow, which can increase the risk of graft cannulation bleeding and ultimately graft thrombosis. There are several proposed mechanisms by which venous outflow stenosis can occur in AV access models. Favored mechanisms cite inflammatory responses to synthetic material, as well as compliance mismatch between native vein and synthetic material resulting in hyperplasia at the transition zone between conduits [15]. Finally, synthetic conduit is prone to degradation over time due to “coring” caused by repeatedly accessing the graft with large bore needles for dialysis, resulting in the formation of pseudoaneurysms.
Although their initial use was met with much excitement, limitations of synthetic AVG such as infection and thrombosis were quickly recognized, leading to the development of biologic or bioengineered conduit, with several examples persisting to this date, including bovine carotid artery (Artegraft®, Artegraft, Inc., North Brunswick, NJ), bovine mesenteric vein (ProCol®, LeMaitre Vascular, Inc., Burlington, MA), and cryopreserved (human) femoral or saphenous vein (CryoVein®, CryoLife, Inc., Kennesaw, GA), among others [16–22]. However, biologic grafts are typically more expensive than standard synthetic ePTFE choices, and early iterations of such grafts were fraught with more troublesome complications such as rapid aneurysmal degradation and did not completely mitigate infectious complications as suspected [23]. Additionally, many patients utilize hemodialysis as a “bridge” to kidney transplantation, subsequently leading to concerns over induction of the immune response and overall antigenic properties of various graft materials.
However, as one can surmise, there have been modifications to previously developed graft materials and the introduction of new synthetic materials, such as polyurethane [24]. Modifications and introduction of new materials have, in theory, provided for earlier cannulation and less graft complications (i.e., ultrafiltration syndrome or “graft weep”) than older materials. New graft construction techniques, such as tissue-engineered vessels and three-dimensional (3D) printing, are unveiling an exciting new frontier for further development of easily handled, personalized, injury proof, and readily available HD access grafts, both biologic and synthetic.
Patient Selection
The preoperative evaluation is critical in the planning of vascular access surgery, and a long-term plan should be kept in mind while caring for these patients. Frequently, patients and surgeons opt to begin access on the nondominant extremity, and it seems that this strategy is fair and acceptable to most patients. The decision to implant an upper extremity AVG depends on the algorithm used when planning subsequent vascular access. The NKF/DOQI project guidelines promote fistula formation in the nondominant extremity [25]. The “fistula-first” initiative would suggest creating autogenous access on the dominant upper extremity once native fistula options have been exhausted on the nondominant side. However, a common practice is to remain on the ipsilateral nondominant limb and proceed with forearm looped (FL) or upper arm brachial artery to axillary vein (brach-Ax) AVG implantation.
One must take care not to overlook previous surgical access attempts or endovascular procedures that could have destroyed or obviated venous outflow (i.e., stent deployment which would not allow for sewing or clamping of the vein or previous graft failure with subsequent thrombosis in that segment of the vein). The general approach to AVG surgery seeks to provide the best immediate result while preserving additional options for future access surgery. Once native fistula possibilities in the nondominant extremity have been exhausted, primary FL AVG placement may be considered. Many surgeons will appropriately proceed to a dominant arm-forearm fistula, in the setting of appropriate venous anatomy, prior to committing to graft implantation. A brach-Ax AVG is a more proximal option usually reserved for failure of forearm access with no compelling options for endovascular or surgical revision. Beyond these locations, axillary artery to axillary vein grafts, chest wall grafts, and the central vein access graft (i.e., Hemodialysis Reliable Outflow [HeRO], Merit Medical Systems, Inc., South Jordan, UT) give the skilled surgeon a variety of options to maintain dialysis access without resorting to a tunneled dialysis catheter.
Determining the degree of target vein patency and the overall quality of venous outflow tends to be our greatest challenge when planning a new vascular access. Typically, one or more means of venous imaging is utilized as an adjunct in planning for new access. Venous duplex mapping of the extremity is simple, convenient, and the most inexpensive method of venous imaging but is limited to the periphery as it cannot evaluate the central veins. Conventional venography, MRV, or CTV are suitable alternatives for evaluation of central venous anatomy [26, 27].
Graft Technology and Graft Materials
In an attempt to provide solutions to the issues, which lead to graft failure, access care providers in collaboration with industry have developed a variety of conduit options and therapies to improve upon the care that we can offer patients in need of long-term vascular access. In that regard, the main focus of access graft advances over the past 10–15 years has been toward improving bleeding, thrombosis, weeping, and infection of access grafts as opposed to addressing the biologic aspects of outflow vein failure and venous proliferative disease. As a result, numerous AVGs with various base scaffolding, wrap or lamination methods, bonding or graft lining, or outflow designs are commercially available for use in our ESRD patients.
Modified ePTFE Luminal Surfaces
Propaten
W. L. Gore & Associates (Flagstaff, AZ) has attempted to make an impact on long-term graft patency by aiming to reduce luminal thrombus by covalently bonding bioactive heparin to the luminal surface of their ePTFE graft known as the Carmeda® BioActive Surface (CBAS®) (Fig. 18.2). Early studies showed encouraging data to support retention of the graft’s thromboresistant bioactive properties over time, but more recent studies do not support the notion of improved patency or performance in the hemodialysis access arena [28–30]. Further prospective, randomized trials may be in order to more fully elucidate the graft’s long-term performance when compared to standard ePTFE dialysis AVGs.


Fig. 18.2
Illustration of CBAS Heparin Surface showing the material surface, base coating, and end-point attached heparin. Also shown are the reactants antithrombin, conformationally altered antithrombin, thrombin, and the inactive thrombin antithrombin complex
Modification of Flow Dynamics
Tapered Grafts
Alteration in the flow pattern of blood through hemodialysis grafts has enabled vascular device companies to attempt to improve pathology related to vascular access, such as steal syndrome, patency, and venous outflow stenosis. Virtually all companies that offer ePTFE for hemodialysis have developed tapered configurations at the arterial end of the graft with the hope of reducing complications such as steal syndromes and high-output heart failure [31]. Most offer a 4 mm–7 mm short taper configuration. Of AV access case litigation, cases related to steal syndrome are the most common. Tapered AVGs can help to reduce the risk of creating a steal situation; however, in general, the potential for steal can be mitigated by alteration of the anastomotic technique on a case-by-case basis.
Venaflo II/Carboflo
In the realm of standard ePTFE material, the choices of graft material are fairly similar and generally come in a 6 mm standard wall configuration which is manufactured by one of five major vendors. As an example of graft modifications, Bard Peripheral Vascular, Inc. (Tempe, AZ) offers the Venaflo II AVG, which aims to optimize hemodynamic venous outflow patterns to reduce outflow vein intimal hyperplasia and thrombosis. Additionally, the Venaflo II as well as Bard’s Carboflo graft is lined with carbon which some studies have suggested result in a reduction in platelet aggregation and thrombus formation within the graft when utilizing this technology [32] (Fig. 18.3).


Fig. 18.3
Top: Venaflo II showing expanded hood and carbon lining. Bottom: Illustration of favorable flow dynamics with expanded Venaflo II hood
Gore Hybrid
The primary intent of the Gore Hybrid graft is to create a sutureless end-to-end vascular anastomosis and possibly reduce intimal cell proliferation and improve flow hemodynamics in the outflow track of arteriovenous access or arterial bypass circuits. The ePTFE transition to stent-graft (nitinol reinforced section) design creates an end-to-end anastomosis and maintains laminar flow from the graft conduit into the recipient vessel [33] (Fig. 18.4). Fluid and flow dynamics testing suggest that this design may reduce the vessel wall shear stresses conveyed on the outflow track when compared to a conventional end-to-side, sutured anastomosis [34, 35]. Presently, there is no peer-reviewed clinical data which proves that altering the outflow dynamics with this device truly has had an impact on the genesis of neointimal hyperplasia or overall graft patency. However, as in the case of most novel technology, ideas for new and innovative applications are often discovered, and as such, there has been success with expanded application of the Hybrid in various cases and complex situations. The Hybrid graft has been used for complex vascular access, peripheral bypass, carotid reconstruction, and renal and mesenteric artery reimplantation during aortic debranching surgery [36–38].


Fig. 18.4
Gore Hybrid Graft
“Low-Bleed” Technology
Flixene
Others have modified PTFE technologies to increase graft wall strength in an attempt to decrease cannulation misadventures, needle hole bleeding, and weeping. The FLIXENE graft manufactured by Atrium Maquet Getinge Group (Hudson, NH) utilizes a Tri-laminate Composite Construction and a hydrostatic protection membrane that dramatically increases burst and suture strength. Due to its construction, it claims to eliminate graft ultrafiltration syndromes that standard ePTFE grafts can develop. Although not FDA approved for early cannulation, there are several reports that support its use as an “early cannulation” graft [39]. In this setting, patency rates are low and infection rates high [40].
Vectra
The Vectra graft (Bard Peripheral Vascular, Tempe, AZ) is a bridge conduit constructed of a polyurethaneurea as an alternative to ePTFE. This rubbery, elastic-type material handles quite differently than the ePTFE grafts, and studies have suggested that this material seals after cannulation within 1–5 min, nullifying the requirement for tissue incorporation into the graft prior to cannulation for hemodialysis [41, 42]. The company suggests that this graft can be used for hemodialysis 24-h status post-implantation.
Acuseal
W. L. Gore & Associates have developed a multilayer ePTFE AVG with the intent to decrease bleeding and facilitate early cannulation. Acuseal is a tri-layer hemodialysis graft with a thicker outer ePTFE layer, surrounding a middle elastomeric layer, which surrounds a thinner ePTFE layer as the innermost graft layer (Fig. 18.5). This graft also features the CBAS heparin-bound technology. The idea is to reduce hematoma and bleeding after needle cannulation and to also minimize catheter contact time by reducing the time required for tissue incorporation around the graft, prior to needle access. Glickman et al. report on Acuseal’s utility as a dialysis graft as well as the graft’s utility on early cannulation; however, only half of the patients in the study were cannulated within 72 h [43]. Primary patency was marginal, but cumulative patency was consistent with the historic AVG literature. Tozzi and Aitken had previously reported similar results [44, 45].


Fig. 18.5
Left: Scanning EM of Gore Acuseal demonstrating the three layers of the graft: Outer graft layer of ePTFE, middle elastomeric membrane, and inner ePTFE graft layer. Right: needle cannulation through Acuseal
Bioprosthetic Technology
Xenogeneic or allogeneic blood vessels, for example, bovine blood vessels or cryopreserved human blood vessels, can be chemically treated leaving collagen, connective tissue proteins, and cells with decreased immunogenicity in order to prepare for their use as conduit for dialysis access. This approach offers the theoretical advantage of matching compliance since the conduit has some of the properties of a blood vessel, though there is evidence of structural alteration by the treatment process resulting in reduced tensile strength and compliance [46]. The first treated xenogeneic conduit was reported in the late 1960s, as a lower extremity arterial bypass conduit made from the collagen matrix of a bovine carotid artery treated by enzymatically removing the musculoelastic portion of the vessel [47]. These grafts are more expensive than standard and most premium grafts but can be a wise option for patients in immunocompromised states, who have small vessels, or those plagued by early thrombosis or chronic infection. There are several xenogeneic or allogeneic grafts currently available as listed below.
Artegraft
In 1970, bovine carotid arteries treated with glutaraldehyde (Artegraft, North Brunswick, NJ) were FDA approved for use among other indications as conduit for dialysis access with similar patency to ePTFE [48, 49]. This product offers a tightly woven and cross-linked, natural collagen matrix conduit derived from bovine carotid artery and has the advantage over ePTFE of behaving more like a human artery and has the theoretical decreased risk of infection because it is made of proteins and cells allowing the host defense to penetrate. However, once implanted in the human host, the media layer of the bovine arteries was susceptible to calcifications in vivo and in some cases resulted in structural degradation and aneurysmal degeneration over time as well as infection [50].
Procol®
Decades later, Hancock Jaffe Laboratories, Inc., Irvine, CA, developed a method to treat bovine mesenteric veins with glutaraldehyde (Procol®, LeMaitre Vascular, Inc., Burlington, MA) (Fig. 18.6). These grafts were developed with the potential advantage of improved vessel compliance and thus theoretic decreased rate of venous stenosis, due to the higher elastin content in the vein when compared to bovine carotid artery. Procol® was FDA approved in 2003 for implantation in patients who have failed at least one prosthetic access graft and was reported to have improved patency over ePTFE, with primary patency at 2 years of 70 % [20, 51]. Despite the positive performance of this product in terms of patency and decreased infection and that it is only modestly more expensive than that of ePTFE, Procol® has not gained widespread adoption.


Fig. 18.6
Placement of Needle through CryoVein
CryoVein
Cryopreserved treated human greater human saphenous veins, femoral veins, and femoral arteries (CryoVein, CryoLife, Kennesaw, GA) are commercially available for use in dialysis access in the USA. CryoLife is FDA registered as human cells, tissues, and cellular and tissue-based product establishment. As the CryoVein graft is made of allogeneic tissue, it is proposed to be more resistant to infection, and some report using cryopreserved vein for salvaging a localized prosthetic graft infection [52, 53]. However, others report no decrease in infection risk at least when these grafts are used as a thigh graft [54]. Secondary patency for CryoVein used as primary conduit for dialysis access was reported to have similar patency when compared to ePTFE [21]. Some have concern about blood type compatibility for these grafts, though they are treated to remove any blood type proteins. Additionally, CryoVein has been implicated in elevating panel-reactive antibodies (PRA), which may be a concern in the dialysis patient being considered for kidney transplantation. CryoLife developed a method of decellularization of their grafts called SynerGraft which resulted in lower levels of PRA, but their decellularized grafts are not currently commercially available [55].
Omniflow II
Omniflow II (LeMaitre Vascular, Inc., Burlington, MA), a glutaraldehyde-tanned ovine collagen tube grown around a Dacron mesh template in a sheep bioreactor, was originally developed by Bio Nova (North Melbourne, Australia) (Fig. 18.7). These grafts were first approved for use in dialysis access in Australia, Germany, and Canada, but more recently gained approval for use in Europe and the USA [56, 57]. The first version of this product, Omniflow I, had low patency of only 30 % at 4 years with occlusive complications, so changes were made to the culture technique and mesh composition. The newer version of this product, Omniflow II, has much improved primary and secondary patencies in preliminary studies which approach that of arteriovenous fistula, with secondary patency at 2 years of 75 % and a lower infection rate than ePTFE [58].


Fig. 18.7
Omniflow II – Dacron mesh template in a sheep bioreactor
Central Vein Pathology
HeRO Graft
As a means to provide care for end-stage access patients with central venous stenosis and/or occlusion, Hemosphere, Inc. (Eden Prairie, MN) developed a hybrid “graft-catheter” vascular access device. Officially classified as a graft, this device is FDA approved for use in the upper extremity in patients who would otherwise be catheter dependent, and it was made available for commercial use in 2008. When this graft is properly implanted, arterial blood is shunted from the donor artery into the central venous system without having to create a formal venous anastomosis. The Hemodialysis Reliable Outflow (HeRO) graft (now Merit Medical Systems, South Jordan, UT) is a completely subcutaneous implanted device, which can bypass central venous stenosis and/or occlusion by traversing the lesion endovascularly and terminating in the right atrium or any available large outflow target vein. This device consists of two components: a conventional ePTFE graft component and an endolumenal, large bore, single-lumen, nitinol-reinforced, silicone outflow component. Preliminary studies have shown that this device has primary and secondary patency rates equal or superior to conventional AVGs and superior infection rates when compared to TDCs [59]. The HeRO graft history, implant techniques, and pitfalls are expanded upon in greater detail later in this textbook.
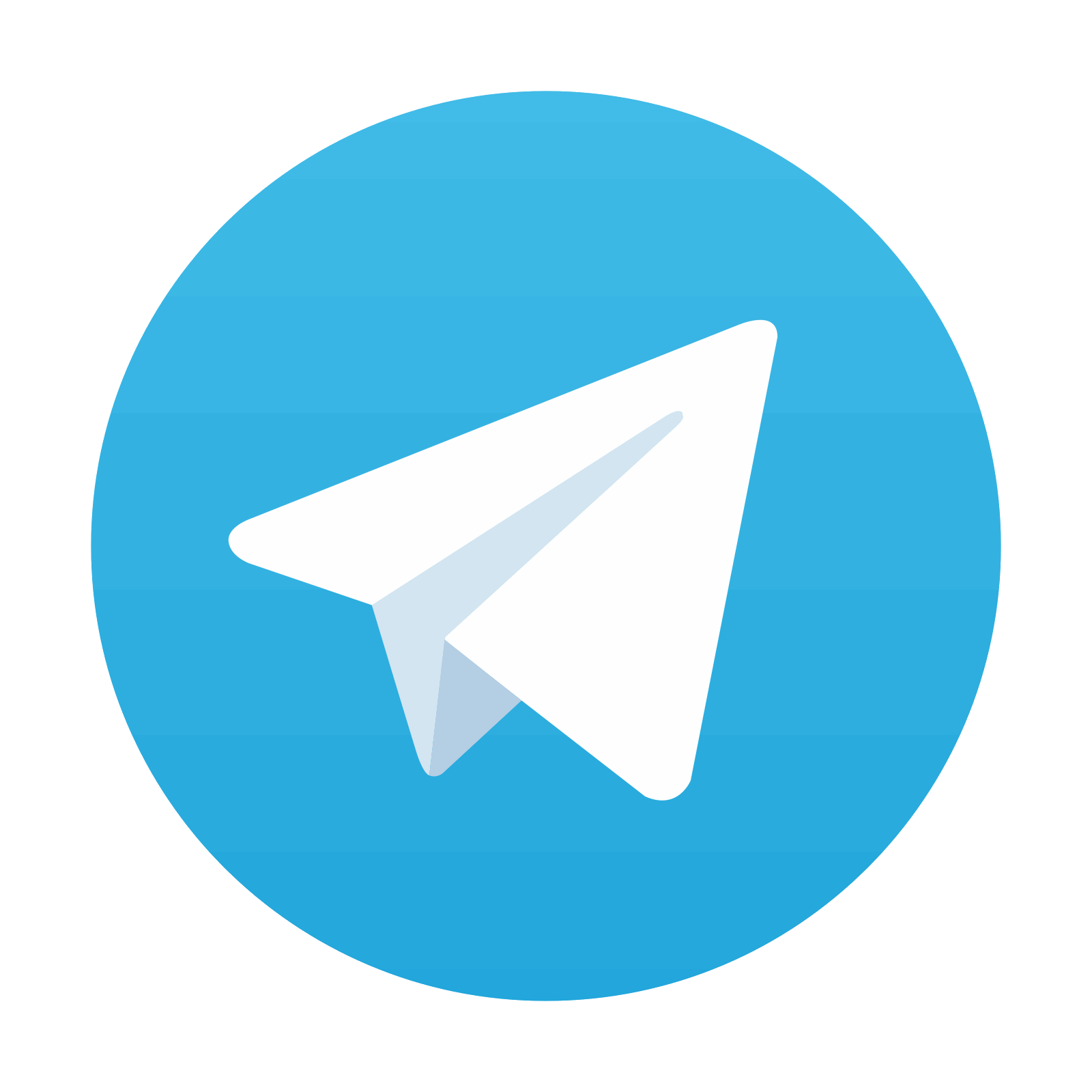
Stay updated, free articles. Join our Telegram channel

Full access? Get Clinical Tree
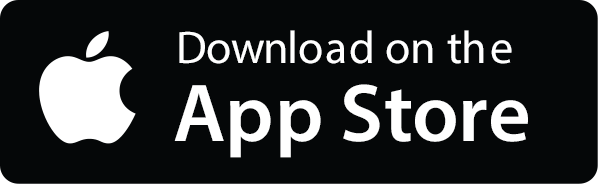
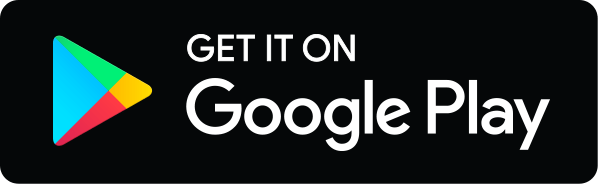
