Helicobacter pylori infection induces chronic inflammation and is the strongest known risk factor for gastric cancer. The genomes of H pylori are highly diverse and therefore bacterial virulence factors play an important role in determining the outcome of H pylori infection, in combination with host responses that are augmented by environmental and dietary risk factors. It is important to gain further understanding of the pathogenesis of H pylori infection to develop more effective treatments for this common but deadly malignancy. This review focuses on the specific mechanisms used by H pylori to drive gastric carcinogenesis.
Key points
- •
Infection with Helicobacter pylori plays a central role in the development of gastric cancer.
- •
The pathogenicity of H pylori infection is attributable to specific interactions between virulence components, variable host inflammatory responses, and environmental factors.
- •
Mechanisms for the carcinogenesis induced by H pylori include changes in host gene expression, alterations in proliferation and apoptosis, and disruption of apical-junctional complexes.
Introduction
Helicobacter pylori was definitively identified by culture in 1984 by Robin Warren and Barry Marshall, and 10 years later this organism was recognized by the International Agency for Research on Cancer (WHO) as a type I carcinogen. H pylori infection is the strongest known risk factor for gastric cancer, and epidemiologic studies have estimated that, in the absence of H pylori infection, 75% of gastric cancers would not exist. H pylori is considered to be the most common causative agent of infection-related cancers, and is estimated to be responsible for 5.5% of all cancers worldwide. Although it is clear that H pylori is the strongest causative agent for gastric cancer, the precise mechanisms for gastric cancer development in response to H pylori infection are less well defined, and a complex interplay of strain-specific bacterial constituents, inflammatory responses governed by host genetic diversity, and/or environmental influences are involved in determining the fate of the host that is persistently colonized by H pylori . This review focuses on the specific mechanisms used by H pylori to drive gastric carcinogenesis.
Introduction
Helicobacter pylori was definitively identified by culture in 1984 by Robin Warren and Barry Marshall, and 10 years later this organism was recognized by the International Agency for Research on Cancer (WHO) as a type I carcinogen. H pylori infection is the strongest known risk factor for gastric cancer, and epidemiologic studies have estimated that, in the absence of H pylori infection, 75% of gastric cancers would not exist. H pylori is considered to be the most common causative agent of infection-related cancers, and is estimated to be responsible for 5.5% of all cancers worldwide. Although it is clear that H pylori is the strongest causative agent for gastric cancer, the precise mechanisms for gastric cancer development in response to H pylori infection are less well defined, and a complex interplay of strain-specific bacterial constituents, inflammatory responses governed by host genetic diversity, and/or environmental influences are involved in determining the fate of the host that is persistently colonized by H pylori . This review focuses on the specific mechanisms used by H pylori to drive gastric carcinogenesis.
H pylori virulence factors that mediate carcinogenesis
The H pylori Type IV cag Secretion System
The cag pathogenicity island ( cag PAI) is a well-characterized and intensively studied H pylori virulence determinant, and strains that harbor the cag PAI increase the risk for distal gastric cancer compared with strains that lack the cag island. Genes within the cag island encode proteins that form a bacterial type IV secretion system (T4SS) that translocates proteins across the bacterial membrane into host gastric epithelial cells. The terminal gene product of the cag island is CagA, and this is one of the substrates that is translocated into host cells by the T4SS. CagA translocation occurs through the interaction of the H pylori protein CagL, which is located on the distal tip of the T4SS pilus, with integrin α 5 β 1 on host epithelial cells. CagI and CagY have also been shown to interact with β 1 integrin and mediate CagA translocation, and CagL physically associates with CagI and CagH. In addition, CagA facilitates its own translocation through specific binding to β 1 integrin. CagA is also reported to be delivered into host epithelial cells by T4SS-induced externalization of phosphatidylserine from the inner leaflet of the cell membrane. The N-terminus of CagA then interacts with phosphatidylserine to gain entry into host epithelial cells. Once inside host cells, CagA is tyrosine phosphorylated by Src and Abl kinases at glutamate-proline-isoleucine-tyrosine-alanine (EPIYA) motifs located within the carboxyl-terminus of CagA.
There are 4 distinct CagA EPIYA motifs (A, B, C, or D) and these are distinguished by different amino acid sequences surrounding the EPIYA motif. In contrast to EPIYA-A and EPIYA-B motifs, which are present in strains throughout the world, EPIYA-C is typically found only in strains from Western countries (Europe, North America, and Australia), and in these strains, an increased number of CagA EPIYA-C sites confers a heightened risk for developing gastric cancer. The EPIYA-D motif is almost exclusively found in East Asian strains. Tyrosine phosphorylation of CagA is tightly regulated, and on injection into the host cell, CagA is immediately phosphorylated on EPIYA-C or EPIYA-D by Src kinase, followed later by phosphorylation on A, B, C, or D motifs by Abl.
Once phosphorylated by members of the Abl and Src family kinases, phospho-CagA targets and interacts with numerous intracellular effectors to lower the threshold for carcinogenesis. Phospho-CagA activates a eukaryotic tyrosine phosphatase (SHP-2), leading to sustained activation of extracellular signal-regulated kinase 1 and 2 (ERK1/2), Crk adaptor, and C-terminal Src kinase, and induces morphologic transformations similar to the changes induced by growth factor stimulation. Interaction of phospho-CagA with C-terminal Src kinase rapidly activates a negative feedback loop to downregulate Src signaling and subsequently the generation of phospho-CagA.
The quantity of phospho-CagA is tightly self-regulated; however, nonphosphorylated CagA also exerts effects within the cell that contribute to pathogenesis. Nonphosphorylated CagA interacts with the cell adhesion protein E-cadherin, the hepatocyte growth factor receptor c-Met, the phospholipase PLC-γ, the adaptor protein Grb2, and the kinase PAR1b/MARK2, and activates β-catenin, culminating in proinflammatory and mitogenic responses, disruption of cell-cell junctions, and loss of cell polarity, all of which promote neoplastic progression. Nonphosphorylated CagA also associates with the epithelial tight junction scaffolding protein ZO-1, and the transmembrane protein, junctional adhesion molecule (JAM)-A, leading to nascent but incomplete assembly of tight junctions at sites of bacterial attachment distant from sites of cell-cell contacts. CagA also directly binds PAR1b/MARK2, a central regulator of cell polarity, inhibits its kinase activity, and promotes loss of cell polarity. These events are discussed in more detail later ( Fig. 1 ).
Another pathway through which H pylori CagA can increase the risk for gastric cancer is through manipulation of apoptosis, by increasing spermine oxidase production in gastric epithelial cells. This generates oxidative damage and selects for a subpopulation of DNA damaged cells that are resistant to apoptosis. H pylori also targets the tumor suppressor p53 to regulate apoptosis in a CagA-dependent manner. CagA interacts with the apoptosis-stimulating protein of p53 (ASPP2) and prevents ASPP2 from inducing apoptosis through activation of p53. This results in proteasomal degradation of p53 and resistance to apoptosis. Recent findings suggest that H pylori induces specific p53 isoforms that inhibit p53 and p73 activities, induce nuclear factor kappa B (NF-κB) activity, and increase cell survival.
CagA is not the only bacterial product delivered through the T4SS; components of H pylori peptidoglycan are also delivered into host cells and trigger signaling pathways that lower the threshold for carcinogenesis. Peptidoglycan interacts with the host intracellular pattern recognition molecule Nod1, which leads to activation of NF-κB-dependent proinflammatory responses such as secretion of IL-8 or β-defensin-2, as well as production of type I interferon (IFN). Translocated peptidoglycan can also activate phosphatidylinositol 3-kinase (PI3K)/Akt signaling, leading to decreased apoptosis, increased proliferation, and increased cell migration.
Vacuolating Cytotoxin A (VacA)
Vacuolating cytotoxin A (VacA), a toxin produced by H pylori , is associated with increased disease risk. VacA exerts multiple effects on epithelial cells including vacuolation, induction of apoptosis, and suppression of T-cell responses, which may contribute to the longevity of infection.
Most H pylori strains possess the vacA gene; however, there is considerable variation in v acA gene structures within the signal (s) region, the middle (m) region, and the intermediate (i) region. The s-region and m-region are stratified into s1 or s2 and m1 or m2 alleles, respectively. vacA s1/m1 strains induce greater vacuolation than s1/m2 strains, and there is typically no vacuolating activity in s2/m2 strains. The vacA s1/m1 allele is strongly associated with duodenal and gastric ulcer disease, and gastric cancer. There are 2 i region subtypes, i1 and i2; and the i region plays a functional role in vacuolating activity. Colonization with vacA i1 strains is strongly associated with the presence of CagA, vacA s1, and gastric cancer.
Of great interest are recent reports suggesting that VacA and CagA are able to counterregulate the effects of each other on the host, representing an effective mechanism to promote persistent colonization of H pylori . Phospho-CagA is able to inhibit trafficking of VacA and thus prevents VacA from reaching its intracellular targets and inducing vacuoles. Nonphosphorylated CagA is also able to oppose vacuolation by blocking VacA activity at the mitochondria. Another example of the antagonistic effects of CagA and VacA is CagA activation of the NFAT (nuclear factor of activated T cells) family of transcription factors. CagA induces translocation of NFAT from the cytoplasm to the nucleus, whereas VacA prevents the translocation of NFAT. VacA is also able to counteract the effects of CagA by inactivating epithelial growth factor receptor, suppressing activation of ERK1/2 mitogen-activated protein kinase, and preventing CagA-mediated cellular elongation. Recent work has identified another mechanism for regulation of CagA by VacA, whereby VacA induces autophagy and degradation of CagA through specific binding of VacA m1 to low-density lipoprotein receptor-related preotein-1 (LRP1) on epithelial cells. In cells expressing a marker of stem cells, CD44 variant 9 (CD44v9) VacA-induced degradation of CagA is circumvented as a result of resistance to reactive oxygen species. These findings further highlight mechanisms through which H pylori can avoid the induction of excess cellular damage and maintain long-term persistence in the gastric niche.
Although CagA and VacA clearly counteract the effects of each other, an exception is facilitation of iron uptake from polarized host epithelial monolayers. In this situation, CagA and VacA act synergistically to create a replicative niche on the apical surface of host epithelial cells by inducing apical mislocalization of transferrin receptors to sites of bacterial attachment.
Adhesins and Outer Membrane Proteins
For H pylori to colonize, deploy virulence factors, and persist within the gastric niche, adherence of H pylori to gastric epithelium is required. Sequence analyses have revealed that an unusually high proportion of the H pylori genome is predicted to encode outer membrane proteins (OMPs), and OMP expression is associated with gastroduodenal ulceration and may heighten the risk for developing gastric cancer (see Fig. 1 ).
Blood group antigen-binding adhesin (BabA) is an OMP encoded by the babA2 gene and binds to fucosylated Lewis b antigen (Le b ) on the surface of gastric epithelial cells. Le b -mediated colonization may increase the pathogenic potential of H pylori and H pylori babA2 + strains are associated with an increased risk of developing gastric cancer, especially when found in conjunction with cagA and vacA s1 alleles.
Another H pylori adhesin is sialic acid-binding adhesin (SabA). SabA binds to the carbohydrate structure sialyl-Lewis x antigen expressed on gastric epithelium, and is associated with increased gastric cancer risk. Sialyl-Lewis x expression is induced during chronic gastric inflammation, suggesting that H pylori modulates host cell glycosylation patterns to enhance attachment and colonization.
Outer inflammatory protein (OipA) is an inflammation-related outer membrane protein, and the presence of a functional oipA gene is associated with more severe disease outcome and gastric cancer. OipA expression is linked to increased production of proinflammatory cytokines including IL-8, IL-1, IL-17, and tumor necrosis factor α (TNF-α), as well as other host effector proteins such as upregulation of matrix metalloproteinase (MMP)-1, an MMP associated with gastric cancer, and induction of OipA can result in activation of β-catenin.
Effects of H pylori on the host immune response that mediate carcinogenesis
Infection with H pylori invariably results in chronic gastric inflammation, and this occurs through a variety of pathways. As discussed earlier, bacterial factors play an important role in determining the severity of disease outcome; however, these alone are not sufficient to dictate the outcome of H pylori infection. The immune response of the host is a key determinant of the development of gastric cancer. H pylori upregulates several inflammatory molecules including interleukin (IL)-1β, IL-32, IL-10, and TNF-α and this plays a key role in H pylori –induced disease progression.
IL-1β is a Th1 cytokine that inhibits acid secretion and is increased within the gastric mucosa of individuals infected with H pylori . Polymorphisms in the IL-1β gene cluster, specifically IL-1β -31 and IL-1β -511, are associated with increased IL-1β production, and are associated with a significantly increased risk for hypochlorhydria, gastric atrophy, and distal gastric adenocarcinoma compared with persons with genotypes that limit IL-1β expression, but only among persons infected with H pylori . Given that IL-1β is a potent inhibitor of acid secretion, is profoundly proinflammatory, and is upregulated by H pylori , colonized individuals harboring high-expression IL-1β polymorphisms are at increased risk for the development of gastric cancer.
Another cytokine that may increase the risk for gastric cancer is TNF-α. TNF-α is a proinflammatory, acid-suppressive cytokine that is increased within human gastric mucosa colonized by H pylori . TNF-α polymorphisms that increase TNF-α production are associated with an increased risk of gastric cancer and its precursors. TNF-α expression has been linked to increased β-catenin signaling through inhibition of glycogen synthase kinase (GSK)-3β through the use of transgenic mice that overexpress the β-catenin agonist Wnt1, and these mice develop gastric dysplasia. In vitro studies have revealed that supernatants from activated macrophages promote β-catenin signaling in gastric epithelial cells, which is attenuated by inhibition of binding of TNF-α to its receptor on gastric epithelial cells, providing a potential mechanism through which enhanced levels of TNF-α may increase the risk for gastric cancer.
In contrast to IL-1β and TNF-α polymorphisms, which lead to increased cytokine production and are associated with increased risk for gastric cancer, polymorphisms that decrease the production of the antiinflammatory cytokine IL-10 reciprocally increase the risk for distal gastric cancer. Investigations into the combinatorial effects of IL-1β, TNF-α, and IL-10 polymorphisms on the development of cancer have revealed that the risk of cancer increases progressively with an increasing number of proinflammatory polymorphisms and 3 high-risk polymorphisms increased the risk of cancer 27-fold over baseline.
The role of IL-32, a recently described proinflammatory cytokine that is overexpressed in various inflammatory diseases and cancer, has also been investigated in H pylori infection. Expression of IL-32 parallels the severity of gastric pathology, with increased expression in gastritis and gastric cancer compared with uninfected gastric mucosa. IL-32 expression induced by H pylori is cag PAI-dependent and requires activation of NF-κB. Within the context of H pylori infection, IL-32 expression is linked to expression of the cytokines CXCL1, CXCL2, and IL-8, suggesting that IL-32 may function as a master regulatory protein that controls cytokine expression in H pylori infection.
Manipulation of the apical-junctional complex by H pylori
Gastric mucosal barrier function is controlled by the apical-junctional complex and is essential for preventing potentially immunogenic elements present in the gastric lumen from gaining access to the gastric mucosa. The apical-junctional complex is composed of tight junctions and adherens junctions, and studies have revealed that H pylori targets many of the host molecules that form apical-junctional complexes, which lowers the threshold for carcinogenesis (see Fig. 1 ).
Studies focused on tight junction proteins have revealed that H pylori recruits the tight junction proteins ZO-1 and JAM-A to the site of bacterial attachment, and disrupts occludin localization at the tight junction. H pylori also induces redistribution of claudin-4 and claudin-5 and disrupts barrier function.
One mechanism through which H pylori can disrupt the tight junction is via the interaction of CagA with partitioning-defective 1b (PAR1b)/microtubule affinity-regulating kinase 2 (MARK2). PAR1b is a member of the PAR1 family of kinases, and has an essential role in maintaining epithelial cell polarity. The PAR1b-binding region of CagA is a sequence of 16 amino acids known as the CagA-multimerization (CM) sequence, which is involved in CagA dimerization. The CM motif binds to the MARK2 kinase substrate binding site and mimics a host cell substrate that inactivates the kinase activity of PAR1, leading to defects in epithelial cell polarity and disruption of tight junctions. Dysregulation of tight junctions also permits H pylori to gain access into sites previously deemed sanctuary sites, such as intercellular spaces and the lamina propria.
H pylori also targets specific components that comprise the adherens junction to promote progression toward gastric carcinogenesis (see Fig. 1 ). Adherens junctions are required for maintenance of adhesive cell-cell contacts, cell polarity, and for signal transduction to the nucleus to regulate transcription. E-cadherin is 1 adherens junction protein that H pylori dysregulates via methylating the E-cadherin gene promoter, thereby reducing E-cadherin expression. Loss of E-cadherin function is associated with gastric cancer, and hypermethylation of the E-cadherin promoter can be reversed by eradication of H pylori.
H pylori infection also disrupts the adherens junction by inducing translocation of membranous E-cadherin, β-catenin, and p120 to the cytoplasm of epithelial cells. Specifically, nonphosphorylated CagA interacts with E-cadherin, leading to destabilization of the E-cadherin/β-catenin complex, and accumulation of cytoplasmic and nuclear β-catenin, which subsequently transactivates β-catenin–dependent genes that may promote carcinogenesis. Through activation of PI3K/Akt signaling by nonphosphorylated CagA, H pylori inactivates GSK-3β, which results in increased cytoplasmic expression of β-catenin. H pylori closely regulates β-catenin activation within host cells through an inhibitory domain within the N-terminus of CagA. The N-terminus of CagA counteracts the effects exerted by the C-terminus of CagA to reduce host cell responses by strengthening cell-cell contacts and decreasing CagA-induced β-catenin activity.
H pylori can also cleave E-cadherin through the actions of the secreted virulence factor high-temperature requirement A (HtrA). Loss of E-cadherin from the adherens junction is associated with dissociation and movement of β-catenin and p120 from the adherens junction into the cytosol. Under normal physiologic conditions, nuclear expression of p120 is low; however, in transformed cells, expression of p120 is increased. H pylori is associated with mislocalization of p120 to the nucleus in human gastric epithelia and in infected murine primary gastric epithelial cells. Further analysis of downstream signaling pathways has determined that p120 mislocalized to the nucleus in response to H pylori acts to relieve transcriptional repression of mmp-7 , an MMP implicated in gastric tumorigenesis, by an interaction with Kaiso. Nagy and colleagues have also reported that a p120 and β-catenin target gene, PPARδ, regulates gastric epithelial proliferation via activation of cyclin E, representing another important mechanism through which H pylori may lower the threshold for the development of gastric cancer.
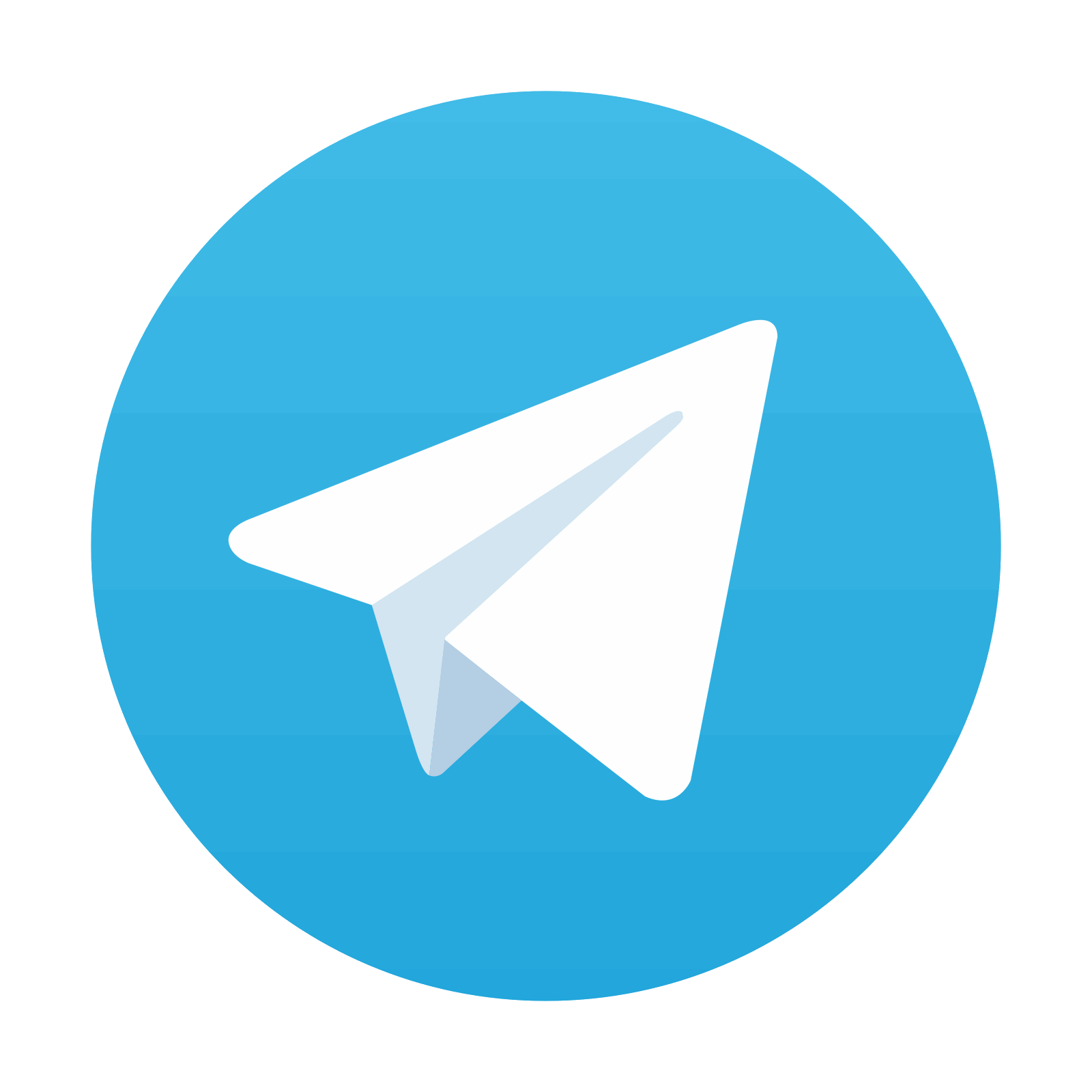
Stay updated, free articles. Join our Telegram channel

Full access? Get Clinical Tree
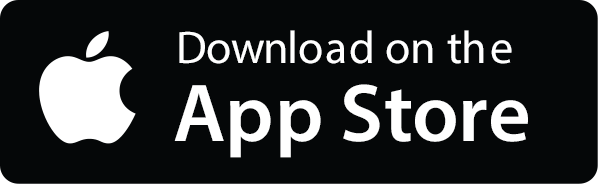
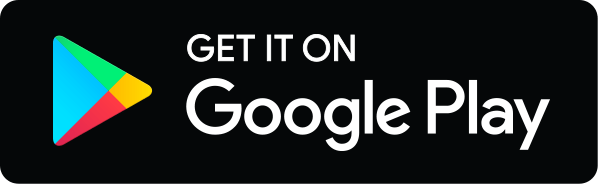