Fig. 28.1
Human ovarian steroidogenesis. The starting point for steroid biosynthesis is the conversion of cholesterol in pregnenolone by P450scc. One route of pregnenolone metabolism is the delta-5 pathway ( red arrows) by action of CYP17 (P450c17). Hydroxylation of pregnenolone at the C17a position forms 17-hydroxypregnenolone, and subsequent removal of the acetyl group forms the androgen precursor dehydroepiandrosterone ( DHEA) . Another route of pregnenolone metabolism is the delta-4 pathway ( purple arrows) in which pregnenolone is converted to progesterone by the action of 3b-HSD (an irreversible conversion). Progesterone is then converted to 17-hydroxyprogesterone by CYP17. In humans, 17-hydroxyprogesterone cannot be further metabolized. Aromatization of androgens to estrogens is a distinct activity within the granulosa layer induced by FSH via activation of the P450 aromatase (P450arom) gene
Importantly, CYP17 is located exclusively in thecal and interstitial cells, the extrafollicular compartment of the ovary, whereas CYP19 (aromatase), that converts androgens to estrogens, is expressed exclusively in GC, the intrafollicular compartment [31, 32]. Thus, aromatization of androgens to estrogens is a distinct activity within the granulosa layer induced by FSH via activation of the P450 aromatase (P450arom) gene. Androgens produced in the theca layer must therefore diffuse into the granulosa layer to be converted to estrogens. Hence, increasing levels of estradiol in the peripheral circulation during follicular phase reflect the release of estrogen from granulosa cells into blood vessels (Fig. 28.2; [18, 22]) .
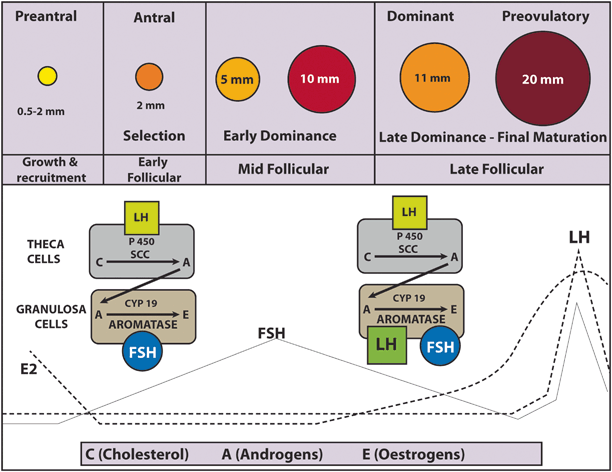
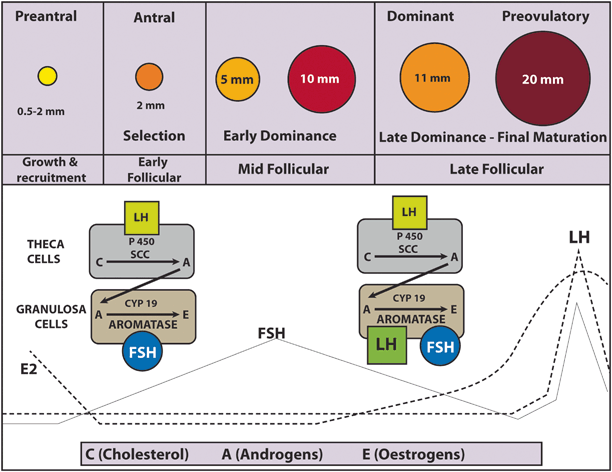
Fig. 28.2
The “two-cell” system. FSH receptors are present only in the granulosa cells. LH receptors are present in the theca cells and initially absent in the granulosa cells. In response to LH, theca cells convert cholesterol to androgens (testosterone and androstenedione). CYP17 is located exclusively in thecal cells whereas CYP19 (aromatase) is expressed only in the granulosa. Thus, androgens must diffuse into the granulosa layer to be converted to estrogen via aromatization induced by FSH. Both FSH and LH act via AMPc production. In the late follicular phase, FSH induces LH receptor formation in the granulosa cells, which acquire LH responsiveness. In the granulosa, LH enhances FSH action (increasing estrogen production)
Theca and granulosa cells also secrete peptides that act as both autocrine and paracrine factors [33]. Insulin-like growth factor (IGF) is secreted by theca cells and enhances LH-mediated androgen production within the thecal compartment as well as FSH-mediated aromatization in granulosa cells. Inhibin and activin are produced in the granulosa cells in response to FSH, and modulate the expression of steroidogenic enzymes, especially P450c17 in theca cells. While inhibin enhances androgen synthesis, activin has an opposite effect. Activin also has the important autocrine role of enhancing FSH action mainly by increasing the expression of FSH receptors [18].
In conclusion, estrogen secretion by the follicle prior to ovulation is the result of combined LH and FSH stimulation of the two cell types, theca and granulosa, influenced by autocrine and paracrine factors (Fig. 28.3; [18, 22]) .
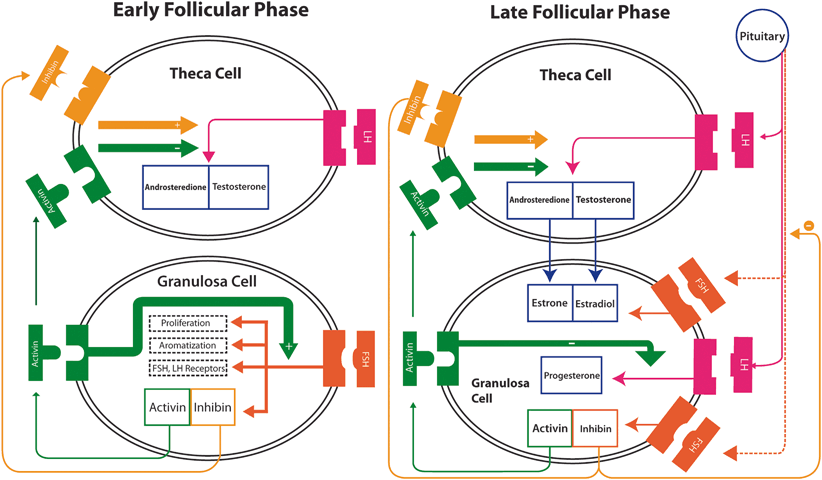
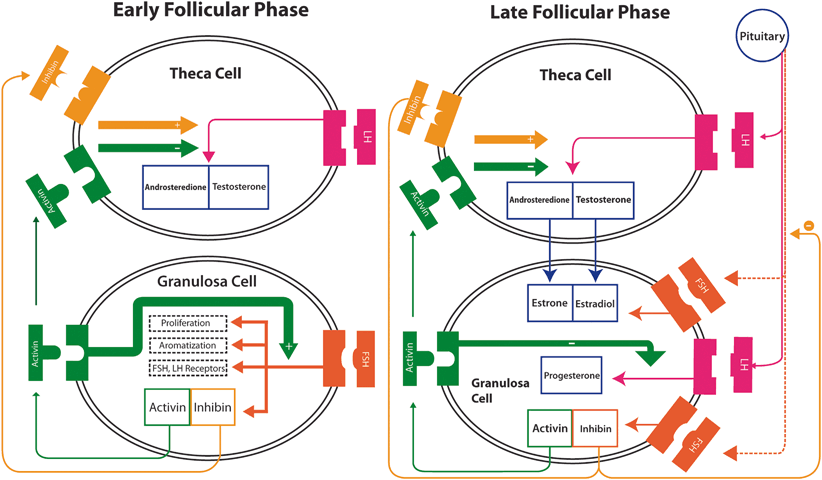
Fig. 28.3
Modulation of steroidogenic enzymes. In the early follicular phase inhibin and activin are produced by the granulosa cells in response to FSH. They have important paracrine functions to modulate the expression of steroidogenic enzymes, especially P450c17 in theca cells. Inhibin enhances LH function thus stimulating androgen synthesis to latter aromatization to estrogen in the granulosa, whereas activin suppresses androgen synthesis. Activin has also an important autocrine role of enhancing FSH action especially by increasing the production of FSH receptors. Production of inhibin by the granulosa cells is increased in the late follicular phase while activin is decreased, with a positive effect on androgen production by theca cells. FSH induces LH receptor formation in the granulosa cells, which acquire LH responsiveness and thus, less FSH dependence. In granulosa, LH enhances FSH action which in turn increase estrogen production, initiates progesterone production (negatively modulated by activin), and control granulosa production of inhibin. The increase in inhibin, in turn, suppresses FSH secretion by the pituitary, important to ensure the dominance of a single follicle
Follicular Selection
The enzymatic activity within both granulosa and theca cells leads to estradiol rise (and inhibin), and as a consequence FSH serum concentration progressively falls due to inhibition of GnRH synthesis in the hypothalamus [16, 18]. The mid-follicular FSH fall causes atresia of less mature follicles that are unable to grow without adequate FSH stimulation. On the other hand, a maturing selected follicle continues to develop because of its increased sensitivity to FSH that in turn makes it less dependent to FSH itself [16]. In addition, FSH induces LH receptor expression in preovulatory follicle granulosa cells [34]. The action of LH on its receptors also activates adenyl cyclase leading to the production of cAMP, which represents an additional stimulus to follicular growth [35]. Thereby, the maturing follicle also reduces its dependency on FSH by acquiring LH receptors and LH responsiveness [16, 34, 36, 37].
LH acts in GC to stimulate steroidogenesis, ultimately leading to an increase in estrogen production (Figs. 28.2 and 28.3) [22]. At first, estrogen levels rise slowly during the late follicular phase. It is then followed by a rapid rise that reaches its peak approximately 24–36 h prior to ovulation [38]. The LH surge occurs when the peak of estradiol is achieved [39]. Under physiologic conditions, an estradiol threshold of 200 pg/mL for at least 2 days is needed for LH surge [40].
Most circulating progesterone (~ 95 %) is produced in the intrafollicular compartment by the granulosa cells via the action of 3β-HSD that catalyzes the conversion of pregnonolone (delta-4 pathway) under LH influence (Fig. 28.1; [22, 29]). Despite a marked increase in progesterone levels measured at the veins of the active ovary in the mid-follicular phase, peripheral concentrations increase only slightly probably due to active liver metabolism [42]. Progesterone can be further converted to 17-hydroxyprogesterone by CYP17 (via delta-4 pathway). However, very little 17-hydroxyprogesterone is converted to androstenedione since human CYP17 catalyses this reaction at only 3 % of the rate for the conversion of 17-hydroxypregnenolone to DHEA [29, 21, 41]. Hence, 17-hydroxyprogesterone is basically the final product of the delta-4 pathway in humans. Moreover, progesterone cannot be metabolized in the GCs because CYP17 is not expressed within this cell compartment; as such, progesterone is the final product of the delta-4 pathway in the intrafollicular compartment and cannot be further converted to estradiol within the GC under the effect of LH (or hCG) [31].
The preovulatory rise in progesterone facilitates the positive feedback action of estrogen on the pituitary; the latter is the key factor to induce the midcycle LH peak. Progesterone also stimulates a midcycle FSH surge, important to support the full expression of LH receptors in the granulosa layer [22, 44]. In experimental studies, it has been demonstrated that elevated levels of estradiol per se can elicit simultaneous surges of LH and FSH, thus indicating that progesterone is not mandatory although it certainly enhances estradiol action [22, 45].
Ovulation
The LH surge triggers resumption of oocyte meiosis that had been halted at prophase I (germinal vesicle stage) . Also, it promotes luteinization of granulosa cells and the synthesis of prostaglandins and other eicosanoids that are essential for follicle rupture. The LH surge is characterized by three phases: (i) a rapidly ascending phase lasting for 14 h, (ii) a plateau of approximately 14 h, and (iii) a descending phase of about 20 h [46]. During the LH surge, intrafollicular levels of progesterone continue to rise up to the time of ovulation. FSH, LH, and progesterone stimulate the activity of proteolytic enzymes, such as matrix metalloproteinases, disintegrin, and metalloproteinase with thrombospondin-like repeats (adamts), which digest collagen in the follicular wall and increase its distensibility [22, 47, 48]. The LH surge also triggers the release of histamine, which has been shown to fully support follicle rupture in some experimental models [22].
Granulosa and theca cells also produce plasminogen activator in response to the LH surge, which activates plasminogen in the follicular fluid to produce plasmin. Plasmin, in turn, generates active collagenase that disrupts the follicular wall [22]. In humans, only one follicle usually achieves dominance and grows up to ovulation. Occasionally, two follicles reach the 10 mm stage at the same time; since both are equally sensitive to FSH, they survive and grow in this relatively low physiological FSH environment. As a result, two ovulations can occur in the same cycle, possibly leading to a dizygotic twin pregnancy [16] .
After ovulation, the corpus luteum is formed. Hormone production by the luteinized granulosa layer is dependent on the number of LH receptors expressed during the preovulatory phase. Luteal cells derived from the theca compartment continue to produce androgens for aromatization into estrogens by luteal cells derived from the granulosa compartment. In addition, progesterone is produced in both luteinized theca and granulosa cells [18]. As such, the corpus luteum produces estradiol and progesterone under the influence of endogeneous LH activity. As the luteal phase progresses, progesterone inhibits LH release via negative feedback. During the luteal-follicular transition, the LH decline causes the corpus luteum to involute and demise [49]. If implantation occurs, the syncytiotrophoblast cells start to produce hCG, which binds to LH/hCG receptors. In early pregnancy, hCG rescues the corpus luteum and maintains luteal function until placental steroidogenesis is well established [22] .
Structure and Function of Endogenous Gonadotropins
Gonadotropins (FSH, LH, and hCG) are proteins covalently linked to a carbohydrate (glycoproteins). They are composed of two noncovalently linked protein subunits, the alpha and beta. The three-dimensional structure and the active conformation of the subunits are maintained by internal disulfide bonds [50]. The alpha subunit contains 92 amino acids and is identical in FSH, LH, and hCG. In contrast, beta subunits are distinct and confer unique receptor specificity as well as differential biological and immunological properties [51]. Protein subunits alone have no biologic activity; the latter is provided by the attachment of carbohydrate moieties forming heterodimers [3]. In general, protein glycosylation plays a very important regulatory role in determining protein activity and function. The extent and pattern of glycosylation convey the differential spectrum of charges, bioactivities and half-lives of each glycoprotein [52]. Glycoproteins have two basic types of glycosylation patterns, the O-linked and N-linked. O-linked glycosylation is characterized by attachment of the carbohydrate N-acetylgalactosamine (GalNAc) to the hydroxyl group of an amino acid, serine, or threonine. N-linked glycosylation involves attachment of N-acetyl glucosamine (GlcNAc) to the amide group of asparagine (Asn; Fig. 28.4; [53]).
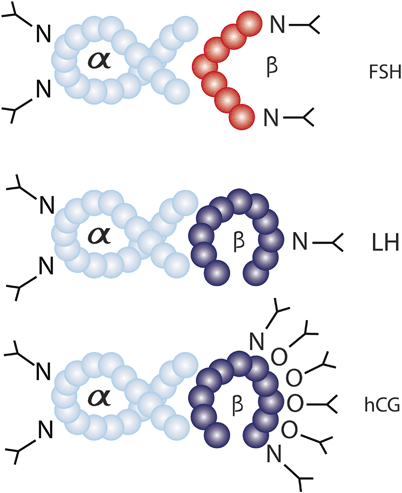
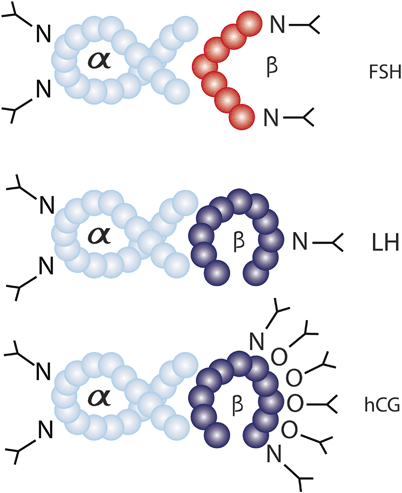
Fig. 28.4
Glycosylation patterns of FSH, LH, and hCG. The alpha subunits of each hormone are identical in amino acid sequence and contain two sites of N-linked glycosylation. The beta subunit confers hormone specificity and contains variable amounts of N-linked glycosylation. LH beta subunit contains a single site of N-linked glycosylation, while FSH and hCG beta subunits contain two sites of N-linked glycosylation. In addition, hCG has an extended C-terminal that contains four sites of O-linked glycosylation
Gonadotropins are further modified in vivo by the addition of a sialic acid (sialylation) or sulfonic group (sulfonation) to the carbohydrate moieties. Both sialylation and sulfonation are physiological processes with major roles in gonadotropin biological activity modulation [54, 55]. The oligosaccharides often terminate with sialic acid and/or sulfonated β1-4-linked GalNAc (SO3-4GalNAc) [54, 56]. The sulfonation pathway leading to terminal SO3-4GalNAc is first regulated by a peptide-specific β1-4GalNAc-transferase adding GalNAc to the subterminal GlcNAc residue on the glycan chains. This enzymatic effect occurs in competition with a β1-4-galactosyltransferase adding galactose to the subterminal GlcNAc in a sialylation pathway leading to terminal sialic acid [54]. There are some elements that must be recognized by GalNAc-transferase [57]. For example, a Pro-Leu-Arg tripeptide motif in the beta subunit of LH and a cluster of cationic amino acids (Pro-Leu-Arg-Ser-Lys-Lys) in the corresponding alpha subunit are recognized by the β1-4GalNAc-transferase leading to a considerably increased rate of GalNAc transfer to the LH molecule. Unlike LH, the tripeptide motif is not present in the beta subunit of FSH thus resulting in a low-enzymatic activity of the peptide-specific β1-4GalNAc-transferase. Therefore, sulfonation plays a considerably lower role in the clearance of FSH compared with LH. On the other hand, sialylation pathway dominates the FSH molecule [54]. The extent and pattern of glycosylation also seem to be under hormonal influence, most likely from a combination of steroidal feedback and GnRH [55, 58].
Molecules with increased number of sulfonated Gal-NAc disappear faster from the circulation than less sulfonated isoforms, due to their affinity to specific SO3-4GalNAc receptors in the liver [54, 59]. On the other hand, increased number of sialic acids enhances half-life [54, 60]. While removal of the carbohydrate moieties of either subunit diminishes gonadotropic activity, experimental data indicate that carbohydrate chains have no role in gonadotropins binding to their receptors [61]. However, carbohydrate components affect the biologic activity of the hormone-receptor complex after binding, thus playing a critical role in activation (coupling) of the adenylate cyclase system [62].
Follicle-Stimulating Hormone (FSH)
Likewise LH and hCG , the alpha subunit of FSH has 92 amino acids (AA). The beta subunit is composed of 111 amino acids with four N-linked glycosylation sites, two on the alpha subunit, added to Asn52 and Asn78, and two on the beta subunit (Asn7 and Asn24) [53, 63]. Thereby, each subunit is attached to two carbohydrate moieties with variable compositions that, in turn, create different isoforms (Fig. 28.5; [3, 53]). These multiple isoforms of FSH differ in their plasma half-lives (ranging from 3 to 4 h) and bioactivity [3] .
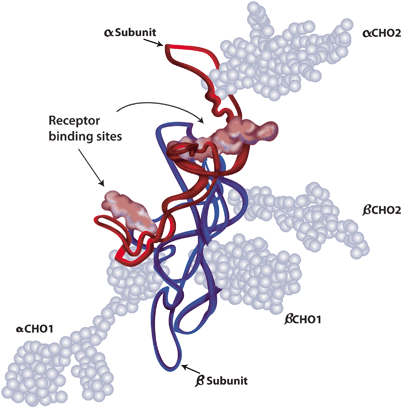
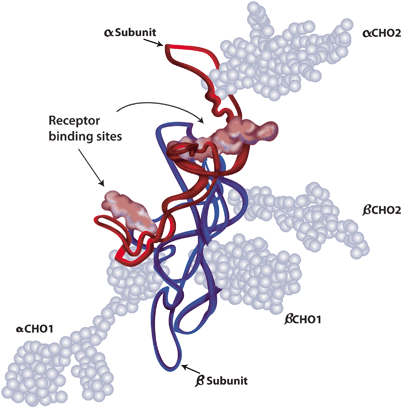
Fig. 28.5
Follicle-stimulating hormone molecule. FSH is a glycoprotein consisted of two subunits, the alpha subunit ( red) and the beta subunit ( blue). There are four carbohydrate attachment sites, two in each subunit. The carbohydrate chains are represented by the light blue balls
Although both sialic acid and sulfonated GalNAc residues modulate the half-lives of human gonadotropins, sialic acid residues are much more common in FSH than sulfonated residues [60]. Increased sialylation enhances FSH metabolic stability by decreasing both glomerular filtration and clearance by sialoglycoprotein receptors in the liver, which is the major site for gonadotropin clearance [64, 65]. It means that the greater the sialic acid content, the longer the hormone remains in circulation [54–56, 60, 64, 65].
Production of different isoforms is controlled by a combination of steroidal feedback and GnRH [55, 66]. The higher the estradiol levels, the lower the FSH sialylation (Table 28.1; [3, 67]). Therefore, the pattern of circulating FSH during the menstrual cycle is dynamic with respect not only to its quantity (concentration) but also to isoform distribution (quality) [58]. The isoform profile is more acidic during early follicular to midfollicular phase, and become more basic shortly before ovulation [3, 58, 68]. These dynamic changes in sialylation are not mimicked by exogenous gonadotropin formulations, and it is unknown whether the absence of such fluctuations during controlled ovarian stimulation (COS) would affect oocyte quality [3] .
Table 28.1
Characteristics of native FSH isoforms
Isoform | Sialic acid content | Biologic activity | In vivo half-life | Predominance |
---|---|---|---|---|
Acid | High | Low | Long | Early/mid-follicular and luteal phase |
Basic | Low | High | Short | Late follicular/preovulatory phase |
Luteinizing Hormone
Although the LH alpha subunit is identical to that of FSH, the beta subunit contains more amino acids (121 AA) than FSH, a difference that confers its specific biologic activity and is responsible for its interaction with the LH receptor [3] .
Elimination of LH from circulation is modulated by the number of both SO3-GalNAc and sialic acid residues attached to the carbohydrate moieties [64]. LH β-subunits contain a single site of N-linked glycosylation (Asn 30) and less sialic acid residues (only 1 or 2); as such, LH has a short initial half-life of only 20–30 min (Fig. 28.6; [64]). Furthermore, LH molecules with increased number of SO3-4GalNAc disappear faster from the circulation due to binding of sulfonic groups to specific SO3-4GalNAc receptors at the hepatic endothelial cells [54, 59]. In rats, it has been demonstrated that the aforesaid hepatic receptors bind bovine LH with highest affinity only when two or more sulfonated GalNAc residues are present on multiple oligosaccharides. Because this particular carbohydrate structure is also found on human LH, it seems possible that a similar system operates in humans [60].
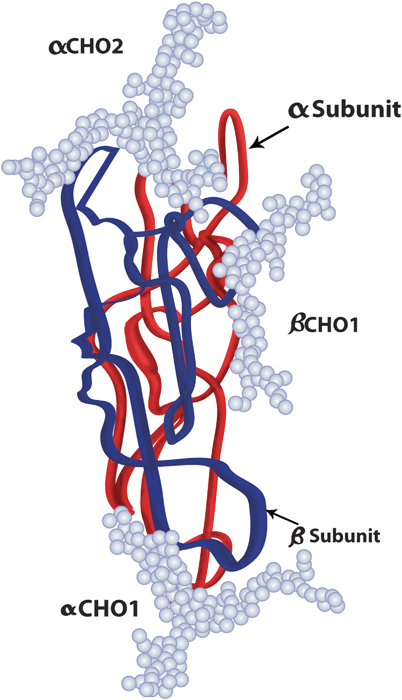
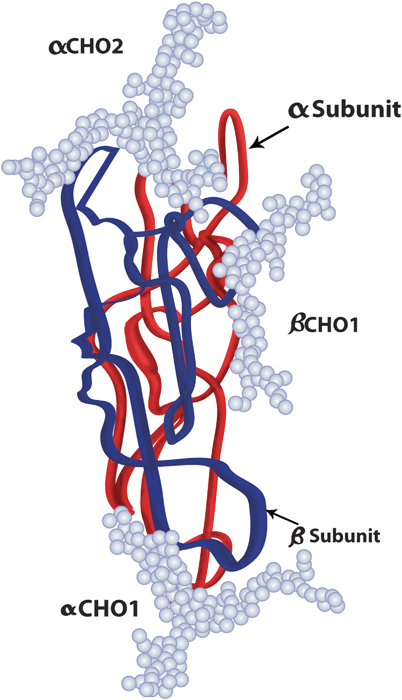
Fig. 28.6
Luteinizing hormone molecule. LH is a glycoprotein with two subunits, the alpha subunit ( red), similar to that of FSH and hCG with two carbohydrate attachment sites, and the beta subunit ( blue), with only one carbohydrate attachment site. The carbohydrate chains are represented by the light blue balls
Likewise FSH, LH shows fluctuations in isoform profile during the menstrual cycle. More basic LH isoforms are seen at midcycle due to considerably decreased sulfonation concomitant with slightly increased sialylation. Both changes increase LH half-life in the circulation, thus explaining the increased levels of serum LH at this period. This change in isoform profile seems to be physiologically important for ovulation triggering [60] .
Human Chorionic Gonadotropin
As already mentioned, the alpha subunit of hCG is identical to those of LH and FSH. Although hCG amino acid sequence is similar to that of LH, a notable difference is the presence of a long carboxyterminal segment with 24 AA containing four sites of O-linked oligosaccharides (Fig. 28.7; [3, 53]). In addition, hCG beta subunits contain two sites of N-linked glycosylation compared with a single site in LH. Due to the higher number of both glycosylation sites and sialic acid residues (approximately 20) than LH, native hCG exhibit a markedly longer terminal half-life in comparison with LH (Table 28.2; [64]) .
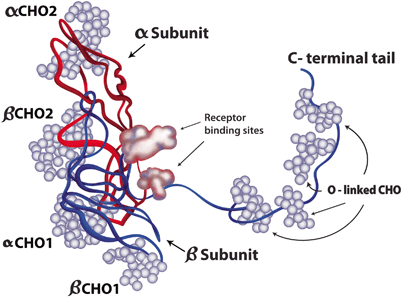
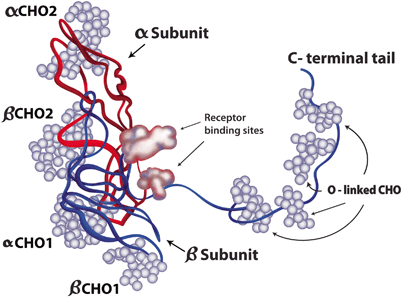
Fig. 28.7
Human chorionic gonadotropin molecule. hCG is similar in its structural attributes to LH. A notable exception is the presence of a long carboxy-terminal segment that is O-glycosylated (O-linked CHO), conferring longer half-life to hCG. The alpha and beta subunits are represented in red and blue strands, respectively, whereas the carbohydrate chains are represented by the light blue balls
Table 28.2
Structural characteristics of endogenous FSH, LH, and hCG
Alpha subunit | Beta subunit | N-linked glycosylation sites (alpha) | N-linked glycosylation sites (beta) | O-linked glycosylation sites (beta) | Carboxyl terminal segment | Half-life | ||
---|---|---|---|---|---|---|---|---|
FSH | 92 AA | 111 AA | 2 | 2 | – | Absent | 3–4 h | |
LH | 92 AA | 121 AA | 2 | 1 | – | Absent | 20–30 min | |
hCG | 92 AA | 145 AA | 2 | 2 | 4 | Present | 24 h |
Landmark Studies in the Development of Exogenous Gonadotropins
Early Understanding of the Hypothalamic–Pituitary–Ovarian Axis
The development of gonadotropin preparations began in 1910, when experimental evidence suggested that the pituitary had a role in the regulation of gonadal stems. Crowe et al. were the first to show that partial pituitary ablation resulted in gonadal atrophy in adult dogs and persistence of infantilism in puppies. Two years later, Aschner confirmed these findings and postulated that pituitary function depended upon the function of higher centers in the brain. This author was the first to suggest that the gonads were affected by pituitary extracts and that their use might have clinical applications [2].
In the late 1920s, numerous studies with different species showed that implantation of anterior pituitary tissue from sexually mature females into sexually immature counterparts induced precocious sexual maturity, marked enlargement of the ovaries and superovulation. In contrast, reproductive function was lost after complete pituitary ablation in both sexes [2, 3].
In 1929, Zondek proposed that two hormones were secreted by the pituitary and had stimulatory effects to the gonads. These hormones were named “Prolan A” and “Prolan B” which are now known as FSH and LH, respectively. These authors described the relationship between the pituitary and gonads and the cyclical secretory dynamics of the two gonadotropins in women [2, 3].
Discovery of hCG, PMSG, and Animal Pituitary Extracts
Zondek, in collaboration with Ascheim (1927), demonstrated that the blood and urine of pregnant women contained a gonad-stimulating substance capable of inducing both follicular maturation and ovarian stromal luteinization when injected into immature mice. They believed that this substance was produced by the anterior pituitary. Subsequently, it has been shown that this substance was human chorionic gonadotropin (hCG) being produced in the placental tissue of the syncytiotrophoblast [2, 3, 69]. In vitro production of hCG was then possible by culturing placental tissue. hCG was commercially launched in 1931 under the label of Pregnon®, later changed to Pregnyl® [2, 3].
In 1930, following the discovery of hCG, a substance found in the maternal–fetal interface of pregnant mares and extracted from their blood was purified, stabilized as a powder, and sterilized for use in laboratory and clinical studies. This substance was named “pregnant mare’s serum gonadotropin” (PMSG; [3]). Early observations revealed that hCG administered alone in the follicular phase failed to promote follicular development and ovulation , indicating that hCG had no effect in the absence of FSH [2, 70]. In contrast, clinical trials demonstrated that PMSG was able to induce an ovarian response, but attempts to fully induce ovulation produced inconsistent results [71]. Concomitantly, researchers sought other gonadotropic animal extracts to be used in the treatment of infertile patients suffering from gonadotropin insufficiency. In the 1930s, gonadotropins extracted from swine and sheep pituitaries were tested clinically to treat such patients [2].
The concept of using PMSG, hog or sheep pituitary gonadotropins to stimulate follicular development and hCG to trigger ovulation (two-step protocol) was introduced in 1941 [72]. Pituitary animal extracts and PMSG were used in both Europe and the USA until the early 1960s, despite the findings that such treatments resulted in the production of neutralizing antibodies (antihormones), which rendered the ovaries unresponsive to repeated stimulation [2, 3, 73]. Due to the aforementioned effect, PMSG was withdrawn from the market in the early 1970s. Nevertheless, animal-derived gonadotropins were still available in some eastern European countries until 1998 [2].
Human Pituitary Gonadotropin
The recognition that animal gonadotropins induced the production of antihormone antibodies, which could neutralize not only the preparation administered but also endogenous gonadotropins, had been the driven forces of scientific and technological efforts to extract and purify gonadotropins from human sources. In this sense, a special interest group, named “G club,” was formed in 1953 to coordinate and promote the development of specific assay procedures as well as bioassay standards and purification methods to obtain gonadotropin preparations suitable for therapeutic purposes [2].
Human pituitary gonadotropin (hPG) was first isolated in 1958 by Carl Gemzell. Between 1958 and 1988, hPG preparations were successfully used for ovulation induction worldwide [74, 75]. However, it soon became clear that the supply of human pituitaries was too limited to fulfill its constantly growing demand [1–3]. Pituitary glands from ten individuals were needed to yield sufficient quantities of gonadotropin to stimulate one patient for one cycle. In addition, there were constant problems with purification and dose standardization [1]. By the mid—1980s, cases of dementia and death due to iatrogenic Creutzfeldt-Jacob disease (CJD) were identified in Australia, France, and the UK, and were linked to the use of hPG and human pituitary growth hormone. As a consequence, hPG was banned from the market approximately 20 years after its introduction [2, 3].
Gonadotropin Preparations Currently Available for Clinical Use
Human Menopausal Gonadotropin
hMG, or menotropin, was first extracted from the urine of postmenopausal women in 1949 [2]. Urine was originally obtained from an Italian nunnery, and early preparations contained varying amounts of FSH, LH, and hCG in only 5 % pure forms [3]. Improvements in the purification techniques standardized FSH and LH activities to 75 IU for each type of gonadotropin in 1963, as measured by standard in vivo bioassays (Steelman–Pohley assay). The first hMG preparation was registered in Italy in 1950, but clinical trials only started 10 years later [3]. hMG preparations have both FSH and LH activity, but the latter is primarily derived from the hCG component present in postmenopausal urine and concentrated during purification [2, 13, 14]. Sometimes hCG is added to achieve the desired amount of LH-like biological activity [2]. In 1999, purified hMG gonadotropins were introduced, allowing its subcutaneous (SC) administration [3, 4]. At present, both conventional hMG and highly purified hMG (HP-hMG) are commercially available in a FSH:LH ratio of 1:1 [4].
Urinary FSH
In the 1980s, pure urinary FSH preparations were produced by removing LH with polyclonal antibodies. The production process was essentially passive since LH was separated from the bulk material, and FSH, together with some other urinary proteins, was collected and lyophilized. Despite being a biologically purer urinary gonadotropin, urofollitropin, or purified urinary follicle-stimulating hormone (hFSH-P) still contained high amounts of urinary proteins [76]. Further technological advances made it possible to use highly specific monoclonal antibodies to extract FSH and produce HP-hFSH. The latter became commercially available in 1993 and is available to date. Such preparations contain < 0.1 IU of LH and < 5 % of unidentified urinary proteins. FSH specific activity is approximately 10,000 IU/mg protein compared to 100–150 IU/mg protein in the earlier urinary hMG preparations (Table 28.3). Likewise HP-hMG, the enhanced purity of HP-hFSH enabled SC delivery [3]. Subcutaneous gonadotropin administration represented an important gain for patients. Consistently better tolerability (lower pain at injection site) was reported with SC injections compared with the intramuscular route. More importantly, it allowed self-administration which is more convenient and less time-consuming, as patients need fewer visits to the clinic or hospital for injections [77, 78].
Purity (FSH content) (%) | Mean specific FSH activity (U/mg protein) | LH activity (IU/vial) | Injected protein per 75 IU (mcg) | |
---|---|---|---|---|
hMG | < 5 | ~ 100 | 75 | ~ 750 |
HP-hMG | < 70 | 2000–2500 | 75 | ~ 33 |
rec-hFSH | ||||
Follitropin beta | > 99 | 7000–10,000 | 0 | 8.1 |
Follitropin alfa | > 99 | 13,645 | 0 | 6.1 |
Recombinant FSH Preparations
Recombinant technology has met the need for a more reliable source of FSH. Under appropriate conditions, the genes coding for the human FSH alpha subunit and betasubunit have been incorporated into the nuclear DNA of a host cell via a plasmid vector, using spliced DNA strings containing the FSH gene and segments of bacterial DNA [2, 3, 79]. Early recombinant technology focused on producing biological molecules in bacterial cells, usually Escherichia coli, as for insulin production. However, due to the complex structure of human gonadotropins and the need of post-translational glycosylation, which define the degradation time and bioactivity, production of functional FSH was not possible using prokaryotes. Certain mammalian cell lines have been otherwise used to produce recombinant complex proteins, including erythropoietin and gonadotropins. The Chinese hamster ovary (CHO) cell line has been chosen to produce gonadotropins because it is genetically stable, fully characterized and easily transfected with foreign DNA. Furthermore, it can be grown in cell cultures on a large scale and produce adequate levels of biologically active rec-hFSH [2, 79].
In 1995, the first rec-hFSH (follitropin alfa) was licensed for clinical use in the European Union. One year later, a similar rec-hFSH (follitropin beta) was made available [2]. In the manufacturing process of follitropin alfa, two separate vectors, one for each subunit, are used to build the master cell bank of FSH-producing cell line, unlike follitropin beta in which a single vector contains the coding sequences of both subunit genes [79, 80]. The subsequent production steps are similar for both preparations (Fig. 28.8). First, a working cell bank is established by growing cells from a single vial that contains identical cell preparations. An aliquot from the selected clone of CHO cells is grown in T-flasks, then subcultured into roller bottles and allowed to expand for up to 36 days. The cells are then mixed with a suspension of microcarrier beads and transferred to a bioreactor vessel with continuous culture media infusion for an average of 34 days. The cell culture supernatant medium, containing the proteins secreted by the cells, is collected from the bioreactor. The harvested “crude FSH” is stored at 48 °C until purification [2]. Lastly, the protein is purified by chromatography, followed by ultrafiltration. The downstream purification process differs for the two commercially available recombinant FSH preparations. The follitropin beta process uses a series of anion and cation exchange chromatography steps, hydrophobic chromatography and size exclusion chromatography. A similar series of chromatography steps are used in the production of follitropin alfa, in addition to an immunoaffinity step with a specific monoclonal antibody that is similar to the one used in the production of HP-hFSH [2, 79]. Each purification step is rigorously controlled in order to ensure batch-to-batch consistency of the final purified product [2]. While the production of urine-derived gonadotropins is often performed in open, nonsterile environments, the production of rec-hFSH takes place in closed, sterile environments, such as the bioreactor. Both the production and the purification of rec-hFSH are subject to continuous quality control assessments, ensuring a pure, consistent and high-quality product [79]. These same concepts highlighted above are now used in the manufacturing process of other recombinant gonadotropins including LH and hCG [2, 3].
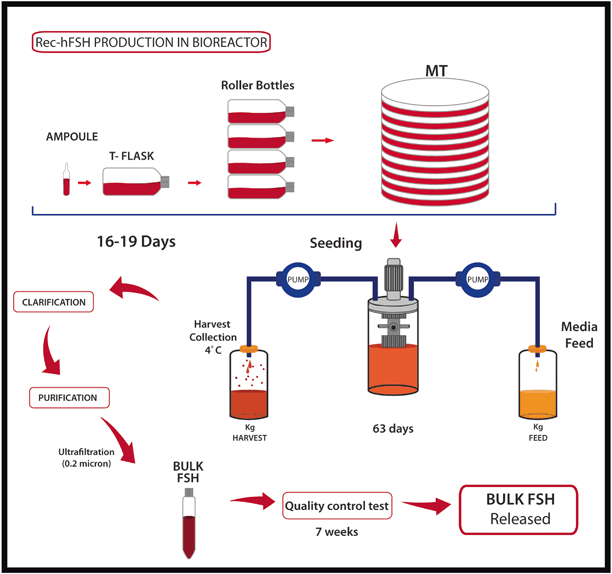
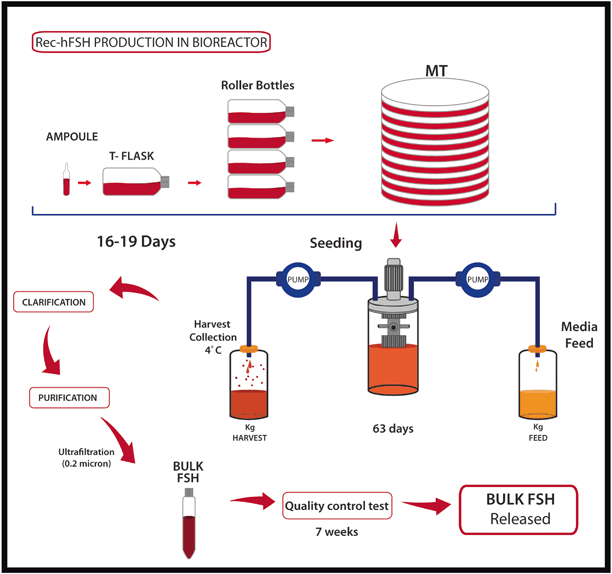
Fig. 28.8
Recombinant technology: how does it work? Chinese hamster ovary cells are first grown in T-flasks, then subcultured in roller bottles and allowed to expand for up to 36 days. Then, cells are mixed with a suspension of microcarrier beads and transferred to a bioreactor vessel perfused continuously with growth promoting medium for an average period of 34 days. The cell culture supernatant medium, containing “crude glycoprotein” is collected from the bioreactor and stored at 48 °C until purification. The protein is purified by chromatography, followed by ultrafiltration. The final product is released after extensive quality control testing over a period of 7 weeks
Both recombinant FSH preparations are structurally similar to native FSH. Despite being named follitropin alfa and follitropin beta, each one comprises alpha and beta glycoprotein chains [3]. However, due to the slight differences in their production and purification procedures the preparations are not identical, with variations in posttranslational glycosylation that result in different sialic acid residue compositions and different isoelectric coefficients [3, 81, 82]. Follitropins alfa and beta are similar to the native FSH isoforms found in the blood around mid-cycle (more basic isoforms), but they differ slightly in the charge heterogeneity as follitropin alfa has slightly more acidic glycoforms than follitropin beta [58, 81]. Despite these differences, both preparations have equivalent immunopotency, in vitro biopotency and internal carbohydrate complexity. Immunoassay showed that initial and terminal half-lives after administration of 150 IU recombinant FSH were 2 and 17 h, respectively. Given their intrinsically similar structures, clinical efficacy is expected to be equally similar [3, 81, 83].
Long-Acting FSH Preparations
Due to the relatively short half-life of FSH (about 1 day), daily FSH injections are needed during the stimulation period to prevent the drop of serum FSH levels below the threshold which cause follicular growth arrest [84]. After each injection, peak serum FSH levels are reached within 10–12 h, and then FSH levels decline until the next injection. Steady state levels are reached only after 3–5 days of treatment, thus dose adjustments before day 5 of stimulation are not advised [85].
A number of technological approaches have been used to develop longer-acting FSH molecules, most of which have involved altering the structure of the FSH molecule itself [85]. Recently, a novel long-acting gonadotropin molecule has been developed by combining rec-hFSH with the C-terminal peptide of hCG using site-directed mutagenesis and gene transfer techniques. It is based on the principle that the highest half-life of hCG is given by the long carboxy terminal segment called C-terminal peptide (CTP) of the beta subunit. The hCG-CTP includes four additional O-linked carbohydrate side chains, each with two terminal sialic acid residues that confer long half-life to hCG [64, 86, 87]. The new molecule has been created using a chimeric gene containing the sequence encoding the CTP fused to the translated sequence of the human FSH beta subunit. The chimera was then transfected with the common glycoprotein alpha subunit and expressed in CHO cells. It has been demonstrated that the presence of the CTP sequence had no significant effect on the assembly or secretion of the intact dimer by stable cell lines. The chimeric recombinant molecule has shown to have similar in vitro receptor binding and steroidogenic activity compared with wild-type FSH, but with significantly enhanced in vivo activity and plasma half-life [85, 88].
The generation of a new CHO cell line expressing the aforementioned FSH hybrid molecule has led to the development of corifollitropin alfa, which was launched in the market in 2010. Corifollitropin alfa is devoid of LH activity. As such, it interacts exclusively with FSH receptors and has a plasma half-life of 65 h [9, 88]. The optimal corifollitropin dose has been calculated to be 100 mcg for women with a body weight ≤ 60 kg and 150 mcg for women with a body weight > 60 kg. From phase II and III studies, it was concluded that a single injection of corifollitropin alfa would replace the first seven daily injections of standard gonadotropins, and that stimulation could be continued with daily FSH injections until the final oocyte maturation had been reached [89]. As the aim of corifollitropin alfa is to simplify treatment and reduce burden associated with multiple injections, it has been developed to be used in GnRH antagonist cycles [85].
Gonadotropin Preparations Containing LH Activity
Currently, there are three groups of commercially available gonadotropin preparations containing LH activity, that is, (i) urinary hMG, in which LH activity is dependent on hCG rather than on pure LH glycoprotein, (ii) pure LH glycoprotein produced by recombinant technology (lutropin alfa), and (iii) a combination of pure FSH and LH glycoproteins in a fixed ratio of 2:1 also manufactured by recombinant technology (Table 28.4; [3]).
Purity (LH content) | FSH activity (IU/vial) | LH activity (IU/vial) | hCG content (IU/vial) | Specific activity (LH/mg protein) | |
---|---|---|---|---|---|
Lutropin alfa | > 99 % | 0 | 75 | – | |
Follitropin alfa + lutropin alfa 2:1 | > 99 % | 150 | 75 | – | 9,000 |
HP-hMG | Unknowna | 75 | 75a | ~ 8 | – |
While hMG has been used for ovarian stimulation since 1960, rec-hLH (lutropin alfa) was introduced in the market in the year 2000 for use in women with gonadotropin insufficiency. The manufacturing process of rec-hLH is similar to rec-hFSH. Lutropin alfa is highly pure and has high-biological activity (9000 IU/mg protein; [3]). It is intended to be used subcutaneously in daily injections. Up to date, lutropin alfa is the only recombinant form of human LH developed for use in ovarian stimulation. It is presented in vials of 82.5 IU lyophilized pure glycoprotein powder to be reconstituted with diluent before administration using a conventional syringe and needle (75 IU of lutropin alfa is delivered per vial) [90].
At present, rec-hLH is used not only to support follicular development during COS in hypogonadotropic hypogonadic woman but also in other categories of female infertility [2, 91, 92]. Recombinant LH has three major differences compared to urinary products. First, it has higher purity and specific activity because it is manufactured using recombinant technology. Second, it is associated with better dose precision due to FbM technology that virtually eliminates batch-to-batch variation and will be discussed later in this chapter [2, 6, 93]. Third, LH activity is derived directly from pure LH glycoprotein unlike hMG, in which hCG is concentrated during purification or added to achieve the desired amount of LH-like biological activity [2]. LH and hCG differ in the composition of their carbohydrate moieties which, in turn, affect bioactivity and half-life. As mentioned earlier, LH activity in serum is 30 times higher when hCG is used due to its higher binding affinity to LH receptors. After administration, recombinant human LH is eliminated with a terminal half-life of 9–12 h in contrast to 23–31 h of hCG [64, 94]. It has been shown that the expression of LH/hCG receptor gene, as well as genes involved in the biosynthesis of cholesterol and steroids in granulosa cells, are lower in patients treated with hMG preparations [95]. Such effects are caused by a constant ligand exposure during the follicular phase due to longer half-life and higher binding affinity of hCG compared with rec-hLH. In animal models, down-regulation of LH receptors is maintained for up to 48 h after hMG administration [96]. These findings indicate that the GCs have lower LH-induced cholesterol uptake, a decrease in the novo cholesterol synthesis and a decrease in steroid synthesis, thus explaining the observed lower serum progesterone levels achieved in patients treated with hMG [95, 97]. The clinical implications of these findings, however, have not been fully elucidated [98].
In 2007, a new fixed combination of rec-hFSH and rec-hLH at 2:1 ratio was launched (follitropin alfa + lutropin alfa) as an alternative for those women who need LH supplementation [8]. The 2:1 ratio of FSH and LH in a fixed dose combination was obtained by recombinant technology and vial filling using protein mass (FbM). The use of FbM as opposed of filled-by-bioassay was possible because the specific activity, isoform distribution and sialylation profile of both gonadotropins are highly consistent among manufactured batches [6]. The bioequivalence of rec-hFSH and rec-hLH administrated alone or in combination has been similar [8, 99].
Human Chorionic Gonadotropin Preparations
During controlled ovarian stimulation , hCG administration has been the gold standard for ovulation induction as a surrogate for the mid-cycle LH surge for several decades [100] . Due to structural and biological similarities, hCG and LH bind to and activate the same LH/hCG receptor [101]. However, the half-life of hCG is much longer than LH, and serum LH activity of hCG is 30 times higher than LH. Therefore, the luteotropic activity of hCG exerts is markedly higher than LH [94, 102]. After hCG administration, approximately 36 h is required for completion of the meiotic process. In the absence of oocyte retrieval, ovulation will ensue approximately 4 h later [103] .
The first chorionic gonadotropin preparations were developed in 1931 from urine of pregnant women. At present, preparations of urinary hCG are marketed in lyophilized vials of 5000 or 10,000 IU to be used intramuscularly. Although improvements have been achieved in the purification process of urinary hCG, with highly purified presentations being introduced in 1976, urinary hCG is nowadays not recommended for subcutaneous administration. In 2001, hCG preparations using recombinant technology were launched (choriogonadotropin alfa). Recombinant hCG is available in prefilled syringes containing 250 mcg of pure hCG, which is equivalent to approximately 6750 IU of urinary hCG [3]. Recently, a new prefilled pen device has been introduced for administration of rec-hCG. Besides the benefits of higher purity, rec-hCG enables subcutaneous administration, unlike urinary hCG which requires intramuscular administration. Recombinant hCG is thus better tolerated and allows patient self-administration [104]. Nevertheless, the clinical efficacy of both urinary and recombinant preparations to induce final follicular maturation and resumption of oocyte meiosis does not seem to differ [105]. In a Cochrane meta-analysis including 11 randomized controlled trials (RCT) with a total of 1187 women, Youssef et al. compared rec-hCG versus urinary hCG for final oocyte maturation triggering in GnRH agonist down-regulated cycles for in vitro fertilization/intracytoplasmatic sperm injection (IVF/ICSI). There was no evidence of a statistically significant difference between rec-hCG and urinary regarding ongoing pregnancy/live birth rate (6 RCTs: odds ratio [OR] = 1.04, 95 % confidence interval [CI]: 0.79 to 1.37; I2= 0 %), incidence of ovarian hyperstimulation syndrome (OHSS; 3 RCTs: OR = 1.5, 95 % CI: 0.37 to 4.1; I2= 0 %) and number of retrieved oocytes (9 RCTs: Mean difference = − 0.04, 95 % CI: − 0.69 to 0.62; I2 = 18 %; [105]) .
A list of gonadotropin preparations currently available for clinical use is provided in Table 28.5 .
Table 28.5
List of gonadotropins available for clinical use
Product | Technology | Brand name | Manufacturer |
---|---|---|---|
hMG | Urine-derived | Menogon®; Repronex® | Ferring |
HP-hMG | Urine-derived | Menopur® Merional® | Ferring IBSA |
HP-hFSH | Urine-derived | Fostimon® Bravelle® Fertinex® | IBSA Ferring Serono |
u-hCG | Urine-derived | Choragon® Brevactid® Choriomon®, Gonasi HP® A.P.L® Biogonadyl® Primogonyl® Profasi® Pregnyl®; Predalon® Endocorion® Corion® | Ferring Ferring IBSA Wyeth Biomed-Lublin Schering Serono Organon Elea Win-Medicare |
rec-hFSH (follitropin beta) | Recombinant | Puregon®; Follistim® | MSD |
rec-hFSH (follitropin alfa) | Recombinant | GONAL-f® | MerckSerono |
long-acting FSH (corifollitropin alfa) | Recombinant | Elonva®
![]() Stay updated, free articles. Join our Telegram channel![]() Full access? Get Clinical Tree![]() ![]() ![]() |