Abstract
The elucidation of monogenic diseases of tubular function has provided valuable insights into mechanisms of kidney electrolyte transport. Proximal tubular disorders include X-linked and autosomal hypophosphatemic rickets caused by mutations in PHEX , FGF23 , DMP-1 , and ENPP1 , all of which interact in the regulation of phosphate transport and bone mineralization. Mutations in NBC1 cause selective proximal renal tubular acidosis (RTA). Generalized proximal dysfunction occurs in hereditary fructose intolerance (aldolase b), Dent disease (CLC-5 and OCRL1), and Lowe syndrome (OCRL1). In the thick ascending limb of the loop of Henle, dysfunction in any of five distinct gene products (NKCC2, ROMK, CLC-Kb, barttin, and the calcium-sensing receptor [CaSR]) produces Bartter syndrome, and loss- or gain-of-function of the CaSR leads to hyper- and hypocalcemic syndromes, respectively. Distal tubular disorders include Gitelman syndrome, caused by mutations in the gene encoding NKCC2, and several syndromes of hypomagnesemia. The latter reflect dysfunction of a wide range of proteins including a Mg 2+ channel (TRPM6), two K + channels (Kir4.1 and Kv1.1), EGF, the γ subunit of the Na + /K + -ATPase, the cyclin M2 basolateral protein, and a mitochondrial tRNA. Collecting duct dysfunction disturbs volume and blood pressure (BP) regulation, urinary acidification, and water reabsorption. Thus Liddle syndrome reflects constitutive activation of the epithelial sodium channel (ENaC); pseudohypoaldosteronism results from inactivation of ENaC, the mineralocorticoid receptor, WNK1 and WNK4 kinases, the Cullin-3 ligase, and the Kelch3 protein; and two forms of pseudohyperaldosteronism reflect dysfunction in 11β-hydroxysteroid dehydrogenase. Mutations in the AE1 anion exchanger, carbonic anhydrase II, or the β1-subunit of the H + -ATPase produce distal RTA, and nephrogenic DI results from inactivation of the V2 vasopressin receptor or aquaporin-2. In some of the above-mentioned diseases, these genes do not account for all cases, and further discoveries will be needed to explain these and other conditions.
Keywords
renal tubular electrolyte transport, hypophosphatemic rickets, Fanconi syndrome, renal tubular acidosis, hypomagnesemia, diabetes insipidus, Bartter, Gitelman, Dent, Lowe, Liddle, Gordon
The coming of age of clinical chemistry in the latter half of the 20th century, bringing with it the routine measurement of electrolytes and minerals in patient samples, produced descriptions of distinct inherited syndromes of abnormal tubular transport in the kidney. Clinical investigation led to speculation, often ingenious and sometimes controversial, regarding the underlying causes of these syndromes. More recently, the tools of molecular biology made possible the cloning of mutated genes found in patients with monogenic disorders of renal tubular transport. These diseases represent experiments of nature, and for anyone interested in pathophysiology and specifically for renal physiologiststs, the insights they reveall are exciting. Some provide gratifying confirmation of our existing knowledge of transport mechanisms along the nephron. Examples include mutations in diuretic-sensitive transporters in the Bartter and Gitelman syndromes. In other cases, positional cloning led to the discovery of previously unknown proteins, often surprising ones that appear to play important roles in epithelial transport. For example, the chloride transporter CLC-5 (gene name CLCN5 ), the tight junction claudin 16 (paracellin 1), and the phosphaturic hormone fibroblast growth factor 23 (FGF23) were discovered through positional cloning in the study of Dent disease, inherited hypomagnesemic hypercalciuria, and autosomal-dominant hypophosphatemic rickets (ADHR), respectively.
Table 38.1 summarizes genetic diseases of renal tubular transport for which the molecular basis is known. The diseases listed are explained by abnormalities in the corresponding gene product. They are all inherited in mendelian fashion, either autosomal or X-linked, with the single exception of a syndrome of hypomagnesemia with maternal inheritance that results from mutation in a mitochondrial tRNA rather than in the nuclear genome.
Inherited Disorder | Defective Gene Product |
---|---|
Proximal Tubule | |
Glucose-galactose malabsorption syndrome | Sodium-glucose transporter 1 |
Dibasic aminoaciduria | Basolateral dibasic amino acid transporter (lysinuric protein intolerance) |
XLHR | PHEX |
ADHR | FGF23 (excess) |
Autosomal recessive hypophosphatemic rickets |
|
HHRH | Sodium-phosphate cotransporter Npt2c |
Familial hyperostosis-hyperphosphatemia |
|
Fanconi syndrome | Aldolase B (hereditary fructose intolerance) |
Fanconi-Bickel syndrome | Facilitated GLUT2 |
Oculocerebrorenal syndrome of Lowe | Inositol polyphosphate-5-phosphatase (OCRL1) |
Dent disease (X-linked nephrolithiasis) |
|
Cystinuria |
|
Autosomal recessive proximal RTA | Basolateral sodium-bicarbonate cotransporter NBC1 |
TAL of Loop of Henle | |
Bartter syndrome | |
Type I | Bumetanide-sensitive Na-K-2Cl cotransporter NKCC2 |
Type II | Apical potassium channel ROMK |
Type III | Basolateral chloride channel ClC-Kb |
Type IV, with sensorineural deafness | Barttin (ClC-Kb–associated protein) |
Familial hypocalcemia with Bartter features | CaSR (activation) |
Familial hypomagnesemia with hypercalciuria and nephrocalcinosis | |
Without ocular abnormalities | Claudin-16 (paracellin-1) |
With ocular abnormalities | Claudin-19 |
Familial hypocalciuric hypercalcemia a | CaSR (inactivation) |
Neonatal severe hyperparathyroidism b | CaSR (inactivation) |
Autosomal dominant hypercalciuric hypocalcemia |
|
Familial juvenile hyperuricemic nephropathy | Uromodulin (Tamm-Horsfall protein) |
DCT | |
Gitelman syndrome | Thiazide-sensitive NaCl cotransporter NCCT |
Pseudohypoparathyroidism type Ia c | Guanine nucleotide-binding protein (Gs) |
Familial hypomagnesemia with secondary hypocalcemia | TRPM6 cation channel d |
Isolated recessive renal hypomagnesemia | EGF |
Autosomal-dominant hypomagnesemia with hypocalciuria |
|
SeSAME/EAST syndromes | Kir4.1 potassium channel |
Dominant hypomagnesemia with ataxia | Kv1.1 potassium channel |
Familial tubulopathy with hypomagnesemia | Mitochondrial tRNA isoleucine |
Dominant or recessive hypomagnesemia | CNNM2 (cyclin M2) |
Collecting Duct | |
Liddle syndrome | β and γ subunits of epithelial Na channel ENaC |
Pseudohypoaldosteronism | |
Type 1 | |
Autosomal recessive | α, β, γ subunits of ENaC |
Autosomal dominant | Mineralocorticoid (type I) receptor |
Type 2 (Gordon syndrome) |
|
Glucocorticoid-remediable aldosteronism | 11β-hydroxylase and aldosterone synthase (chimeric gene) e |
Syndrome of AME | 11β-hydroxysteroid dehydrogenase type II |
Distal RTA | |
Autosomal dominant | Basolateral anion exchanger (AE1) (band 3 protein) |
Autosomal recessive, with hemolytic anemia | Basolateral anion exchanger (AE1) (band 3 protein) |
Autosomal recessive (with hearing deficit) | β1 subunit of proton ATPase |
Autosomal recessive (hearing deficit variable) | α4 isoform of α subunit of proton ATPase |
Carbonic anhydrase II deficiency f | Carbonic anhydrase type II |
Nephrogenic diabetes insipidus | |
X-linked | AVP 2 (V2) receptor |
Autosomal | AQP-2 water channel |
a Results from heterozygous mutation.
b Results from homozygous mutation.
c Gene also expressed in proximal tubule where functional abnormalities are clinically apparent.
d Gene also expressed in intestine.
e Gene expressed in adrenal gland.
f Clinical phenotype can be of proximal RTA, distal RTA, or combined.
Disorders of Proximal Tubular Transport Function
Selective Proximal Transport Defects
Sodium resorption in the proximal tubule occurs through secondary active transport processes in which the entry of sodium is coupled either to the entry of substrates (e.g., glucose, amino acids, or phosphate) or to the exit of protons. Autosomal-recessive conditions of impaired transepithelial transport of glucose and dibasic amino acids have been shown to be caused by mutations in sodium-dependent transporters that are expressed in both kidney and intestine, resulting in urinary losses and intestinal malabsorption of these solutes. Other disorders with renal-selective transport defects result from mutations in transporters expressed specifically in kidney.
Impaired Proximal Phosphate Reabsorption
Hypophosphatemic rickets can be inherited in X-linked, autosomal-dominant, and autosomal-recessive patterns. All three modes are associated with renal phosphate wasting, with a reduced maximal transport capacity for phosphate. In all three, serum levels of the phosphate-regulating hormone (phosphatonin) FGF23 are elevated, and serum levels of 1,25-dihydroxyvitamin D are not elevated despite the stimulus of hypophosphatemia.
X-linked (dominant) hypophosphatemic rickets (XLHR) is the most common form of hereditary rickets. Mutations in XLHR involve a phosphate-regulating gene with homologies to a neutral endopeptidase on the X chromosome (PHEX) that is expressed in bone and is indirectly involved in the degradation and processing of FGF23. Elevations in FGF23 are sufficient to explain the renal phosphate wasting, but other factors independent of FGF23 appear to contribute as well to the bone demineralization and rickets.
The rare ADHR is associated with mutations in the gene encoding FGF23 that protects the phosphatonin from proteolytic cleavage. FGF23 inhibits renal phosphate reabsorption by inhibiting expression of two genes encoding sodium-dependent phosphate transporters in proximal tubule, SLC34A1 (encoding the Na-dependent phosphate transporter Npt2a) and SLC34A3 (encoding Npt2c). FGF23 inhibits the 1-hydroxylation of 25-hydroxyvitamin D, likely explaining why hypophosphatemia in these three conditions is not associated with either elevated levels of 1,25-dihydroxyvitamin D or hypercalciuria.
Autosomal-recessive inheritance of hypophosphatemic rickets has been reported in association with mutations in one of two genes. In ARHR type 1, the mutated gene is DMP1 , encoding the dentin matrix protein 1 (DMP-1), a bone matrix protein that appears to play a role with PHEX in regulating bone mineralization and FGF23 production. ARHR2 is associated with mutations in ENPP1 , encoding ectonucleotide pyrophosphatase/phosphodiesterase 1. Patients with either subtype resemble those with autosomal-dominant (i.e., FGF23 mutations) and X-linked (PHEX mutations) hypophosphatemic rickets.
Hereditary hypophosphatemic rickets with hypercalciuria (HHRH), an autosomal-recessive disorder, is different from XLHR, ADRH, and ARHR, all of which are associated with reduced urinary calcium excretion. In contrast, hypophosphatemia in HHRH is associated with appropriate elevations of 1,25-dihydroxyvitamin D levels, and FGF23 levels are normal or reduced. This profile in HHRH is consistent with a primary defect in phosphate transport, and indeed the disease is associated with mutations in SLC34A3 , encoding the proximal tubule phosphate transporter Npt2c. Expression of this transporter, as well as that of SLC34A1 encoding the more abundant transporter Npt2a, respond to physiologic stimuli such as parathyroid hormone (PTH) and dietary phosphate. Knockout of the mouse homologue of SLC34A1 reproduces the features of human HHRH except for rickets; a mouse knockout of SLC34A3 manifests hypercalcemia and hypercalciuria, but not hypophosphatemia, nephrocalcinosis, or rickets; and a double knockout of both genes produces mice with the full phenotype of hypophosphatemia, hypercalciuria, nephrocalcinosis, and rickets.
Excessive Proximal Phosphate Reabsorption
Inherited hyperphosphatemia in the familial hyperostosis-hyperphosphatemia syndrome represents a mirror image of ADHR and XLHR, with excessive renal phosphate reabsorption, persistent hyperphosphatemia, inappropriately normal levels of 1,25-dihydroxyvitamin D, and low levels of FGF23. This can result from mutations in one of three genes identified to date. These genes encode FGF23 itself; a Golgi-associated biosynthetic enzyme, N -acetylglucosaminyl (GalNac) transferase 3, that is involved in glycosylation of FGF23 and is necessary for its secretion; and Klotho, a transmembrane protein that complexes with the FGF23 receptor and regulates its affinity for FGF23. Together, these discoveries are fleshing out our understanding of the role of bone in the complex regulation of mineral metabolism.
Impaired Proximal Bicarbonate Transport
Selective proximal renal tubular acidosis (RTA) is inherited in an autosomal recessive manner and is associated with mutations that inactivate the basolateral sodium bicarbonate cotransporter NBC1, encoded by the gene SLC4A4 . These patients typically exhibit short stature and often suffer blindness from ocular abnormalities, including band keratopathy, cataracts, and glaucoma; these ocular manifestations probably are a consequence of impaired bicarbonate transport in the eye. Mutations in the SLC4A4 gene result in impaired transporter function or aberrant trafficking of the protein to the basolateral surface. This gene belongs to the same group as the gene encoding the anion exchanger AE1 (now designated SLC4A1 ), which is mutated in distal RTA. Proximal RTA can also be inherited in an autosomal-dominant fashion, though the responsible gene(s) have not been identified.
Inherited Fanconi Syndrome: Hereditary Fructose Intolerance, Lowe Syndrome, and Dent Disease
The renal Fanconi syndrome represents a generalized impairment in reabsorptive function of the proximal tubule and comprises proximal RTA with aminoaciduria, renal glycosuria, hypouricemia, and hypophosphatemia. Some or all of these abnormalities are present in individual patients with Fanconi syndrome. Inherited causes of partial or complete Fanconi syndrome include hereditary fructose intolerance, Lowe syndrome, and Dent disease.
Hereditary fructose intolerance is caused by mutations that result in deficiency of the aldolase B isoenzyme, which cleaves fructose-1-phosphate. Symptoms are precipitated by intake of sweets. Massive accumulation of fructose-1-phosphate occurs, leading to sequestration of inorganic phosphate and deficiency of adenosine triphosphate (ATP). Acute consequences can include hypoglycemic shock, severe abdominal symptoms, and impaired function of the Krebs cycle that produces metabolic acidosis; this is exacerbated by impaired renal bicarbonate reabsorption. ATP deficiency leads to impaired proximal tubular function in general, including the full expression of the Fanconi syndrome with consequent rickets and stunted growth. ATP breakdown can be so dramatic as to produce hyperuricemia, as well as hypermagnesemia from the dissolution of the magnesium-ATP complex. Avoiding dietary sources of fructose can minimize acute symptoms and chronic consequences such as liver disease.
Characteristic features of the oculocerebrorenal syndrome of Lowe include congenital cataracts, mental retardation, muscular hypotonia, and the renal Fanconi syndrome. In contrast, Dent disease is confined to the kidney. In both syndromes, low-molecular-weight (LMW) proteinuria is a prominent feature along with other evidence of proximal tubulopathy such as glycosuria, aminoaciduria, and phosphaturia. One important and puzzling difference is that proximal RTA with growth retardation can be severe in patients with Lowe syndrome, but it is not a part of Dent disease. Some patients with Lowe syndrome or Dent disease may have rickets, which is thought to be a consequence of hypophosphatemia and, in Lowe syndrome, of acidosis as well. Hypercalciuria is a characteristic feature of Dent disease and is associated with nephrocalcinosis in most and kidney stones in many patients with Dent disease; nephrocalcinosis and nephrolithiasis are less common in Lowe syndrome. Kidney failure is common in both of these conditions, typically occurring in young adulthood in Dent disease and even earlier in patients with Lowe syndrome.
Dent disease is caused in most cases by mutations that inactivate the chloride transporter CLC-5. This transport protein is expressed in the proximal tubule, the medullary thick ascending limb (MTAL) of the loop of Henle, and the alpha-intercalated cells of the collecting tubule. In the cells of the proximal tubule, CLC-5 co-localizes with the proton-ATPase in subapical endosomes. These endosomes are important in the processing of proteins that are filtered at the glomerulus and taken up by the proximal tubule through adsorptive endocytosis. The activity of the proton-ATPase acidifies the endosomal space, releasing the proteins from membrane-binding sites and making them available for proteolytic degradation. CLC-5 mediates electrogenic exchange of chloride for protons in these endosomes, facilitating endosomal acidification. Mutations that inactivate CLC-5 in patients with Dent’s disease interfere with the mechanism for reabsorption of LMW proteins and explain the consistent finding of LMW proteinuria. Glycosuria, aminoaciduria, and phosphaturia occur commonly but less consistently and may reflect altered membrane protein recycling, as CLC-5 interacts directly with several trafficking proteins. Hypercalciuria appears to reflect a dysregulation of renal 1-hydroxylation of 25(OH)-vitamin D.
Lowe syndrome is associated with mutations in OCRL1, which encodes a phosphatidylinositol-4,5-bisphosphate-5-phosphatase. In renal epithelial cells, this phosphatase is localized to the trans -Golgi network, which plays an important role in directing proteins to the appropriate membrane. The CLC-5 protein and the OCRL1 phosphatase interact with the actin cytoskeleton and are involved in assembly of the endosomal apparatus. Similarities in the kidney features of these two syndromes may be the result of defective membrane trafficking. A subset of patients with mutations in OCRL1 have no cataracts or cerebral dysfunction and no RTA (“Dent 2” disease). Such mutations occur predominantly in the 5′ end of the gene and are critical to expression of the gene in the kidney, but not to expression of transcripts in eye or brain.
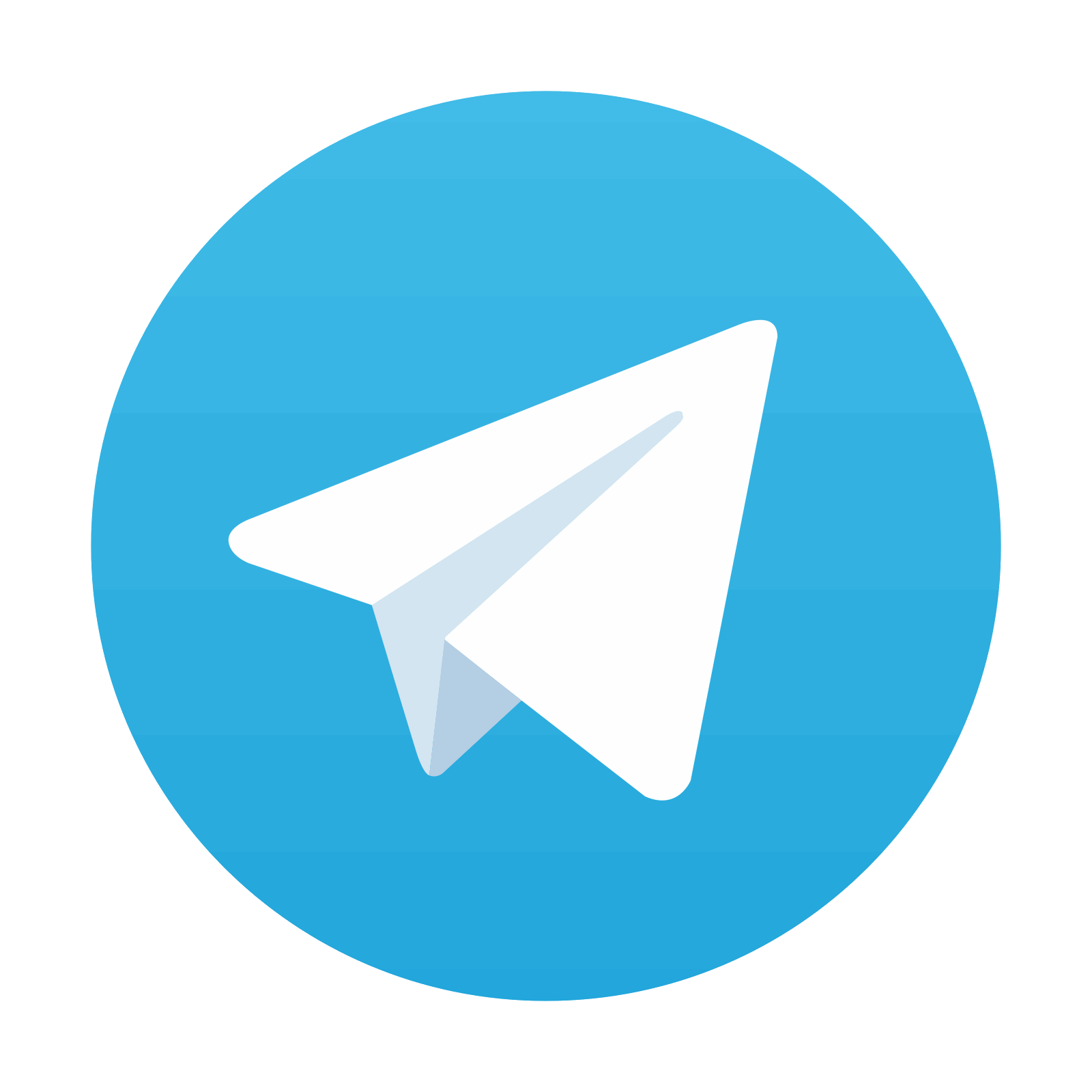
Stay updated, free articles. Join our Telegram channel

Full access? Get Clinical Tree
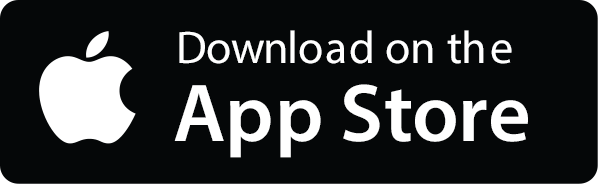
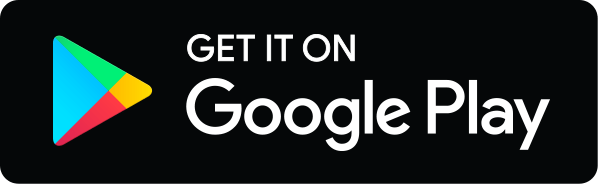