Fig. 8.1
Structure of nuclear metaphase chromosome. The chromatin is a complex of unbroken long double-stranded helical DNA carrying genes and proteins. The nucleosome is the basic chromatin subunit composed of DNA wrapped around histones. (Reprinted from [12], p. 114. With permission from Jaypee Brothers Med. Publishers Ltd.)
Genes can be defined as stretches of DNA sequence that encode different functions including protein synthesis after mRNA transcription and functional RNA synthesis. There are about 40 million genes in the human genome [12]. Very few genes exist as continuous coding sequences (called “exons”); the vast majority is interrupted by noncoding regions called “introns.” Introns are initially transcripted to RNA in the nucleus; however, mature mRNA is not found in the cell cytoplasm and therefore introns are not represented in the protein end product. Although some genes lack introns, the majority contains at least one or several of them [13]. Interestingly, introns rather than exons constitute the greater part of the gene length. Introns are removed from synthesized RNA by splicing. This process also joins exons to form mRNA that codes-specific protein synthesis.
The term “locus” defines the exact position a gene occupies on a chromosome while the term “allele” stands for a variant form of a specific gene. A gene is represented by two alleles. When both alleles are identical the individual is termed homozygous for that gene. However, when they are different the individual is termed heterozygous for such gene.
Genotype is the genetic code that determines physical and functional traits of an individual. The genotype is not sensitive to environmental factors . In contrast, phenotype refers to the peculiar characteristics and traits of an individual such as external appearance, biochemical or molecular properties, and behavior. In other words, phenotype is the sole expression of the genotype, and this correlation may be altered by environmental factors .
There are basically four DNA nucleotide sequence variations [12]. The first is the single-nucleotide polymorphism (SNP), which is a single-nucleotide alteration along the DNA sequence that occurs at a rate of 1 in every 1250 bases throughout the 3 billion base pairs of the human genome . Up to 20 million SNP exist, although not evenly distributed. As an example, SNP may alter the DNA nucleotide sequence from AAGGTAA to ATGGTAA, which is a variation found in at least 1 % of the human population, and that may occur in both coding (exons) and noncoding regions (introns). Most SNPs have no pathological significance; however, very few code for new amino acids or have a role as stop codon, that is, signal a termination in translation. The second variation of DNA nucleotide sequence is a mutation. Mutations are considered as a type of SNP by Hamada et al. [12]. However, unlike SNPs, mutations affect less than 1 % of the general population and most of them correlate with harmful events. The term “mutation” refers to a novel genetic change which was not previously known in a family and, sometimes, may allude to an abnormal allele. Alterations in DNA sequence may contribute to diseases or adverse effects on the host or offspring. Mutations may occur in germ cells during gametogenesis (sperm or oocyte formation) and are usually transmitted to the offspring or render the host infertile. Transmitted mutations may determine different degrees of disease severity ranging from minor physiologic modifications to death. Somatic mutations usually alter the genetic load after conception and play a key role in the pathogenesis of human diseases, notably cancer. In other cases, mutations solely represent phenotype variations. The third variation of DNA nucleotide sequence is short tandem repeats (STR), or in other words, sequences of two to four base pairs such as CG and CAG that repeat in tandems and may occur in exons, introns, and 5′ genomic sequences. Expansion of such repeats may be associated with neurodegenerative disorders and fragile X syndrome [12]. Finally, the last one is the copy number variation (CNV), a relatively common type of structural genetic sequence change that affects 8–12 % of human genome [14]. The CNV requires a long DNA segment of over 1000 bases to be inserted or deleted. The CNV may affect one gene or a complete set of genes and usually results in an increased frequency of its expression or in the amount of the coded protein. Although harmless in the vast majority of cases, CNV has been associated with cancer and increased susceptibility to systemic lupus erythematous [12].
Genetic diseases can be didactically classified in four major categories: chromosomal disorders, single-gene mutation-related disorders, multifactorial disorders, and mitochondrial genetic disorders [12]. Chromosomal disorders are chromosomal numeric abnormalities (aneuploidy) or structural changes. Single-gene disorders occur in a Mendelian fashion or can be sex linked. Multifactorial disorders involve alterations in the expression of multiple genes and are likely influenced by environmental factors affecting the resulting phenotype. Mitochondrial genetic disorders are maternal related and contribute to certain debilitating states.
Role of Genetics in Male Fertility
Sperm production is controlled by genetic factors and involves three distinct phases: (i) mitotic proliferation of spermatogonial cells into primary spermatocytes; (ii) meiotic division of spermatocytes generating haploid secondary spermatocytes after the first meiotic division, and haploid spermatids after second meiotic division; and (iii) spermiogenesis of the haploid spermatids. Hence, a single-diploid spermatocyte will eventually generate four haploid spermatids (Fig. 8.2). Spermatogonial series are kept in a latent state inside the fetal testis until puberty when they increase in number by repeated mitotic divisions. The full process of spermatogenesis starts in adolescence (between 13 and 16 years). It has been estimated that 2000 genes are essential for spermatogenesis and spermiogenesis, from which only 30 genes are present in the Y chromosome [15, 16].
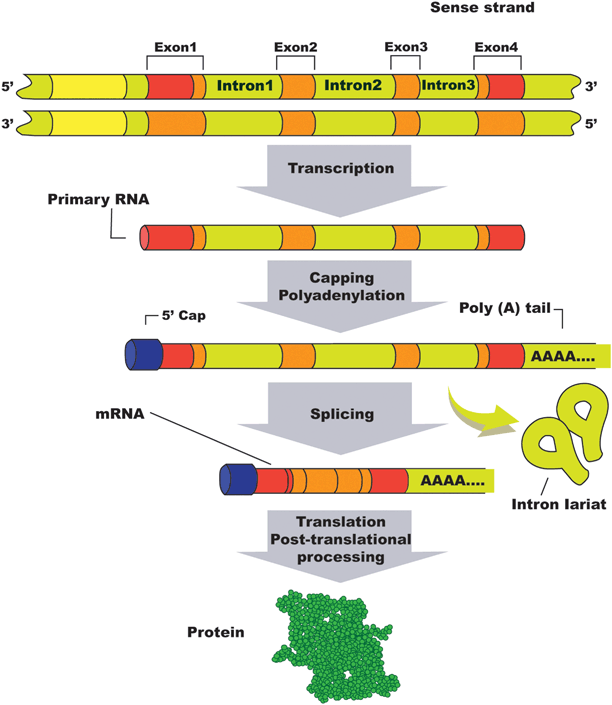
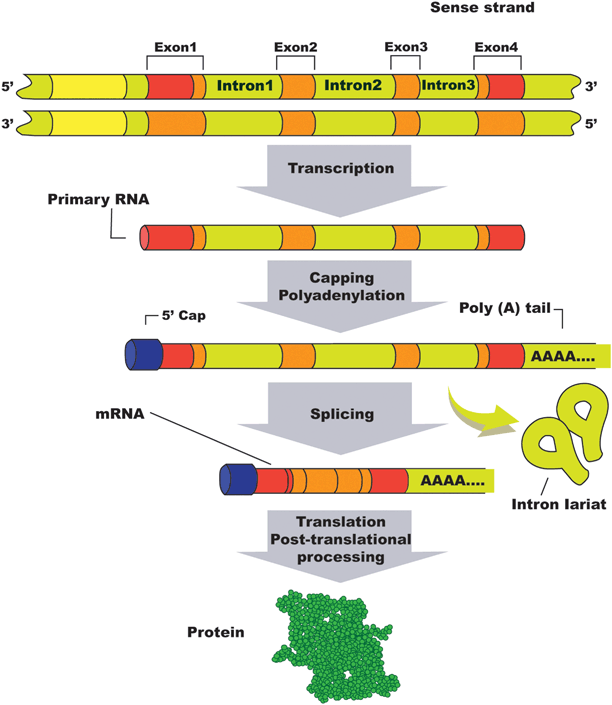
Fig. 8.2
Gene organization, splicing process, and post-translational protein synthesis. (Reprinted from [12], p. 115. With permission from Jaypee Brothers Med. Publishers Ltd.)
Genetic diversity takes place during the prophase of the first meiotic division. Sister chromatids of paired homologous chromosomes form areas of synaptonemal contact during the pachytene stage. These contacts enable chromatids to exchange segments of genetic material between homologous chromosomes resulting in the formation of new chromosomes. Next, separation of the homologous chromosomal pairs is followed by the second round of meiotic division which results in spermatid formation. During the last phase of spermatogenesis , round spermatids undergo cytologic transformations in a process called “spermiogenesis” to form elongated spermatozoa. Nuclear chromatin condensation, acrosomal cap formation and midpiece and flagellar structure development are the three essential steps of spermiogenesis [12].
Chromatin modification and condensation during spermiogenesis are essential for sperm function. The coiling of human sperm DNA material is mediated by specific proteins which control condensation and decompression in a time-dependent manner. During the condensation process, 90–95 % of histones are replaced by protamines [17]. Protamination of sperm chromatin not only facilitates the nuclear compaction necessary for adequate sperm motility but also helps to protect the genome from oxidizing and harmful molecules within both the male and female reproductive tracts [17]. It also diminishes DNA transcriptional activity. Transition proteins 1 and 2 (TP1 and TP2) are proteins of intermediate basicity that bind to the DNA and facilitate removal of histones. Finally, transitional proteins are completely replaced by protamines [18].
Two types of protamines (P1 and P2) are expressed in humas in an equally balanced amount [19]. Improper processing of protamine transcripts and altered P1/P2 ratio are associated with subfertility [20, 21]. Chromatin compaction is the result of disulfide bonds among protamines, which provide the formation of toroid chromatin structures with high resistance to mechanical disruption [22]. Protamines contain large bands of positively charged arginine residues which neutralize the negative phosphodiester backbone of the DNA. Such configuration minimizes DNA repulsion allowing it to double back and fold up onto itself, thus creating the highly compact toroid [23]. Toroids are the most compressed form of sperm DNA. They are stacked side-by-side in a way to provide maximum surface area allocation (Fig. 8.3). Tight condensation makes the DNA resistant to endonuclease digestion [24]. Matrix attachment regions (MARs) are located between each protamine toroid, anchoring them in place (toroid linkers). MARs are very sensitive to nuclease activity as they contain histones. In addition to providing the link between DNA and the nuclear matrix, MARs also serve as a checkpoint for sperm DNA integrity. Protamination not only protects sperm chromatin from damage during transport but also plays an epigenetic role in silencing protamine-bounded genomic regions until time comes for transcription [25]. Once the spermatozoon fuses with the oocyte, protamines are completely replaced by histones from the oocyte within the first 4 h.
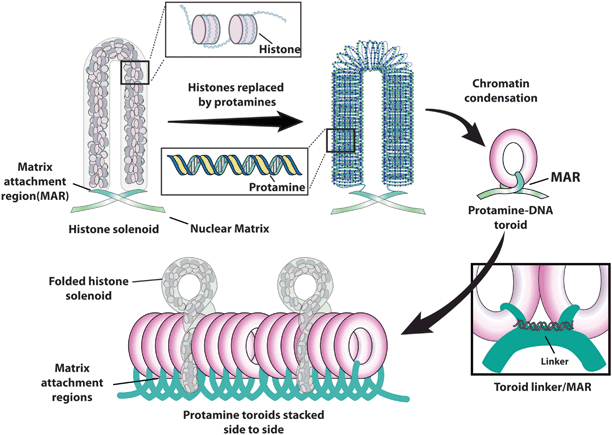
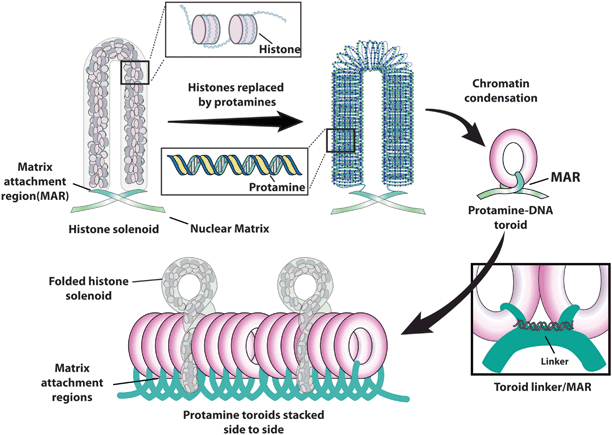
Fig. 8.3
Sperm DNA structure. (Adapted from [12], p. 119. With permission from Jaypee Brothers Med. Publishers Ltd.)
The second most prevalent form of sperm DNA structure is histone-bound DNA, accounting for about 4 % of the DNA in mature sperm. Histones are primarily found in association with gene promoter sites. Genes vital for spermiogenesis and early fertilization events are preferentially associated with histones [26, 27]. Human histones are more precisely associated with miRNA clusters, HOX gene clusters, and the promoters of stand-alone developmental transcription and signaling factors [26]. Since sperm DNA histones are not replaced by those of the oocyte after fertilization , it is likely that inflicted damage to histone-bound sperm DNA will be transmitted to the embryo without detection and possible modification.
Genetic abnormalities including chromosomal aberrations and monogenic diseases have been estimated to respond for 10–15 % of human infertility cases [28]. Infertile men with genetic alterations usually present with impaired spermatogenesis , genital structural abnormalities, reduced testicular size, hypogonadism and sperm dysfunction, although no detectable abnormalities can also be seen. One important genetic aspect associated with infertility and normal phenotype is sperm chromatin damage [29–33]. A wide range of clinical scenarios may arise from sperm DNA damage including infertility, miscarriage, and increased risk of defects in the offspring [34–37]. Infertile males usually have high rates of sperm DNA damage which are mainly associated with oxidative stress (OS). A chapter in this book is dedicated to OS in unexplained male infertility .
Genetic Disorders Associated with UI
Genetic abnormalities with possible roles in unexplained male infertility can be grouped into five main categories: (i) chromosomal defects in the somatic cells, (ii) gene mutations and polymorphisms in the somatic cells, (iii) sperm DNA damage, (iv) sperm chromosomal abnormalities, and (v) epigenetic disorders. The first two categories affect individuals with abnormal genotypes in somatic cells while sperm DNA damage and epigenetic disorders affect individuals with normal genotypes in somatic cells. Sperm chromosomal abnormalities can be originated from both individuals with abnormal and normal genotypes.
Chromosomal Defects
Chromosomal defects are the most common genetic abnormalities in infertile males, accounting for 2.1–15.5 % of cases [38]. Klinefelter syndrome, chromosome translocations, inversions, and deletions fall in this category, and the vast majority of affected individuals display severely compromised semen quality. Translocation carriers, however, may present with different sperm production phenotypes, varying from normal spermatogenesis to inability to produce spermatogonia [39]. Chromosomal translocations occur when nonhomologous chromosomes exchange segments. Translocations involve both sex chromosomes and autosomes, which can be either balanced or unbalanced; most often they are either associated with severe sperm abnormalities or lethal for fetuses. Robertsonian translocation (RT) represents a translocation category in which two acrocentric chromosomes fuse at the region next to the centromere causing loss of their short arms. The resulting balanced karyotype has only 45 chromosomes including the chromosome with the translocation, which is actually constituted by long arms of two chromosomes. As the short arms of the five acrocentric chromosomes (chromosomes 13, 14, 15, 21, and 22) harbor multiple copies of ribosomal RNA, the loss of their short arms is not harmful. RTs are the most frequent structural chromosome abnormalities in humans, affecting the fertility status of one in 1000 men [28]. Although their prevalence in subfertile males is estimated to be only 0.8 %, it is nine times higher than in the general population [40]. In heterozygous carriers, RT chromosomes and their acrocentric homologues may undergo either alternate segregation or adjacent segregation at meiosis. Alternate segregation results in the formation of balanced gametes carrying either RT or normal karyotype, whereas adjacent segregation leads to the formation of aneuploidy gametes. Carriers of RTs may exhibit normal phenotype but otherwise be infertile due to more or less severe oligozoospermia [38]. In addition, RT may predispose to abnormal embryo development that account for few cases of UI [41]. There is also a risk of unbalanced gamete production and therefore an increased risk of spontaneous abortion and unbalanced offspring. The most relevant clinical situation involves carriers of translocations in chromosome 21 as they are at risk of producing a child with Down syndrome due to a 21q trisomy inheritance.
Gene Mutations
Like chromosomal defects, gene mutations are usually related to severely abnormal sperm production phenotypes. Microdeletions in the Y chromosome azoospermia factor (AZF) region, mutations in the cystic fibrosis gene, and mutations and polymorphisms of the androgen receptor gene are classic examples of genetic abnormalities in this disease category. In the context of UI, however, abnormalities of interest include mutations of cation channel of sperm (CatSper) and sperm mitochondrial genes.
CatSper Gene
In humans, sperm hyperactivation is not as well defined as it is for other species, and only a small part of the sperm population undergoes hyperactivation at each time. The proportion of sperm exhibiting hyperactivated motility is positively correlated with the extent of zona binding, acrosome reaction, zona-free oocyte penetration, and fertilization capacity in vitro. Studies have demonstrated that voltage-gated calcium channels (Cation CatSpe; CatSper 1–4) and proton pumps located in the principal piece of the sperm flagellum regulates hyperactivation [42, 43]. Intracellular calcium entry is induced by intracellular alkalinization secondary to H+ extrusion through voltage-gated proton pumps [43]. Variations in intracellular pH and calcium also regulate the sperm ability to fertilize the egg [42]. CatSper ion channel is a recently discovered protein complex composed of six subunits. Among these, four are α subunits (CatSper 1-4) with calcium-selective pore while two are transmembrane proteins with large extracellular domains and unknown function (CatSper beta and CatSper gamma) [44]. In a study involving infertile men with normal semen parameters, Avenarius et al. noted that patients with mutated CatSper1 gene had an abnormally low proportion of sperm exhibiting hyperactivation [45]. In addition, a study in mice has shown that mutations in each CatSper (1–4) ion channel protein led to infertility despite normal seminal parameters and testicular development [46]. CatSper 1, 2, 3, and 4 have been mapped to chromosomes 11q12.1, 15q13-q15, 5q31.2, and 1p35.3, respectively. Further studies are needed to clarify the genetic and molecular nature of fertilization in patients with defective hyperactivation and UI . Also, the impact of minor mutations in human CatSper (1–4) genes in men with UI is yet to be determined.
Sperm Mitochondrial DNA (mtDNA) Mutations
Spermatozoa mitochondria are located around the midpiece in a helical arrangement containing 1–2 mtDNA. mtDNA encodes 37 genes that regulate oxidative phosphorylation. It differs from nuclear DNA in respect to replication, repair mechanism, genome packing, and position. In addition, mtDNA is not protected by histones and is physically associated with the inner mitochondrial membrane, where highly mutagenic oxygen radicals are generated in the respiratory chain [47, 48]. The leakage of these free radicals makes mitochondria a major source of reactive oxygen species (ROS). These features may explain why mtDNA is more prone to mutations than nuclear DNA [38, 49]. Besides, excess production of ROS may induce the opening of the membrane permeability transition pore and release of free radicals, cytochrome C, and other factors that may ultimately lead to sperm apoptosis.
Most studies focusing on sperm mtDNA mutations and infertility have shown that sperm phenotypes display motility abnormalities related to dysfunctional axonemes [50]. However, Jensen et al. studied the mtDNA polymerase gamma gene (POLG) in patients with UI and found that CAG repeat lengths ranged from 8 to 13 triplets. Homozygotes with ten repeats in both alleles (10/10) were the most common, and constituted 75 % of all subjects. These authors found that 14.3 % of men with UI were homozygous not10/not10 compared with only 2.3 % in the unselected control group ( P = 0.001) and 0.9 % in the fertile group ( P = 0.0001). The odds ratio of having not10/not10 and being normozoospermic and infertile was 18.1 (95 % CI 3.3–184) [51]. Despite the undefined role of determining mtDNA mutations as a marker of male infertility, prognosis for pregnancy is good in cases treated with ART since mtDNA is not transmitted to the offspring [38].
Sperm DNA Damage
Sperm chromatin integrity is crucial to normal reproductive outcomes and its disturbance has been associated with infertility, decreased embryo quality and implantation in ART, and increased miscarriage [30, 31, 34–36]. A normally packaged chromatin in the nucleus of mature spermatozoa is important not only for DNA accommodation but also DNA protection. DNA-strand breaks are often seen as a result of oxidative stress during sperm DNA packaging; however, such breaks are corrected by a complex mechanism involving phosphorylation and polymerization. It has been hypothesized that OS may disrupt spermiogenesis and result in the production of sperm with poorly remodeled chromatin [52]. These defective gametes are released from the testes carrying a tendency to undergo apoptotic events that include the activation of free radical generation by mitochondria. As a consequence, lipid peroxidation and oxidative DNA fragmentation are induced, ultimately resulting in cell damage. Although the likely result is infertility, these defective cells may still fertilize oocytes.
In general, oocytes have repair mechanisms that can alleviate the mutational load to be carried by the embryo. However, the effectiveness of such repair mechanisms is negatively affected by female age [53] . When sperm DNA fragmentation repair fails, de novo genetic mutation will be transmitted to the embryo. Such mutations may lead to different scenarios including embryo development arrest, increased risk of miscarriage, and increased risk of infertility, childhood cancer and imprinting diseases in the offspring [54] .
DNA damage may result from both intrinsic and extrinsic disturbances usually associated with OS . Intrinsic causes include, for example, protamine deficiency and DNA packaging defects [55]. A small, nonclinically significant percentage of spermatozoa from fertile men possess detectable levels of DNA damage. However, sperm DNA damage is increased in infertile men [31, 56]. It has been shown that approximately 5–15 % of the latter have complete protamine deficiency thus rendering DNA vulnerable to attacks such as those inflicted by ROS [17, 20]. External factors include genital inflammation, heat, radiation, chemotherapy, and cigarette smoking [57]. Cigarette smoking generates high levels of OS, directly increasing seminal leukocyte concentrations and seminal ROS generation, which are known to induce OS. Smoking also reduces the concentration of antioxidants in the seminal plasma thus decreasing its oxidant scavenging capacity [58–60].
Rybar et al. studying sperm DNA fragmentation index (DFI), found that the proportion of sperm with fragmented DNA was significantly higher in normozoospermic men from couples with UI compared with young men with no infertility history (DFI 17.4 % ± 10.8 % versus 12.2 % ± 7.3 %; P< 0.01) [7] . Earlier studies have also suggested that sperm chromatin damage in male partners from couples with UI was higher than fertile males (1.9 % ± 1.1 % versus 0.3 % ± 0.4 %, P< 0.05), but its association with semen quality as conventionally assessed had been ambiguous [61–63]. Abnormally elevated levels of sperm DNA damage have been observed in approximately 5 % of infertile men with normal semen analysis in comparison with 25 % of counterparts with abnormal semen analysis. At present, it seems sound to assume that the poor-quality sperm chromatin is indicative of male subfertility regardless of number, motility, and morphology of spermatozoa [31, 32, 56, 64]. In fact, in a prospective study involving 165 couples (presumably fertile) seeking fertility counseling in the USA, sperm chromatin structural assay (SCSA), which is one of the methods for sperm DNA integrity assessment, has proved to be the best predictor for successful pregnancy in first pregnancy planners as well as in couples undergoing intrauterine insemination (IUI) [30]. Currently, sperm DNA fragmentation assessments are considered useful tools in the investigation, counseling, and treatment of infertile couples [65] .
Sperm Chromosomal Abnormalities
It has been estimated that there is a threefold increase in sperm aneuploidy frequencies in infertile men compared with fertile men [66]. Sperm aneuploidies have been associated with severe sperm defects, infertility, miscarriage, in vitro fertilization (IVF) failure and increased risk of chromosome abnormalities in newborns. Albeit counterintuitive, there is little or no evidence to support the argument that an aneuploidic spermatozoon is at disadvantage in fertilizing an oocyte compared with normal haploid sperm [67]. There is, however, an obvious correlation between the increased frequency of aneuploidy observed in sperm (around 3 % in infertile men) and paternally derived de novo chromosome abnormalities in embryos, fetuses, and newborns conceived using intracytoplasmic sperm injection (ICSI)) . This increase in de novo abnormalities after ICSI is estimated to affect 2–3 % of conceptions [68, 69], which represents an approximate threefold increase compared with natural conceptions.
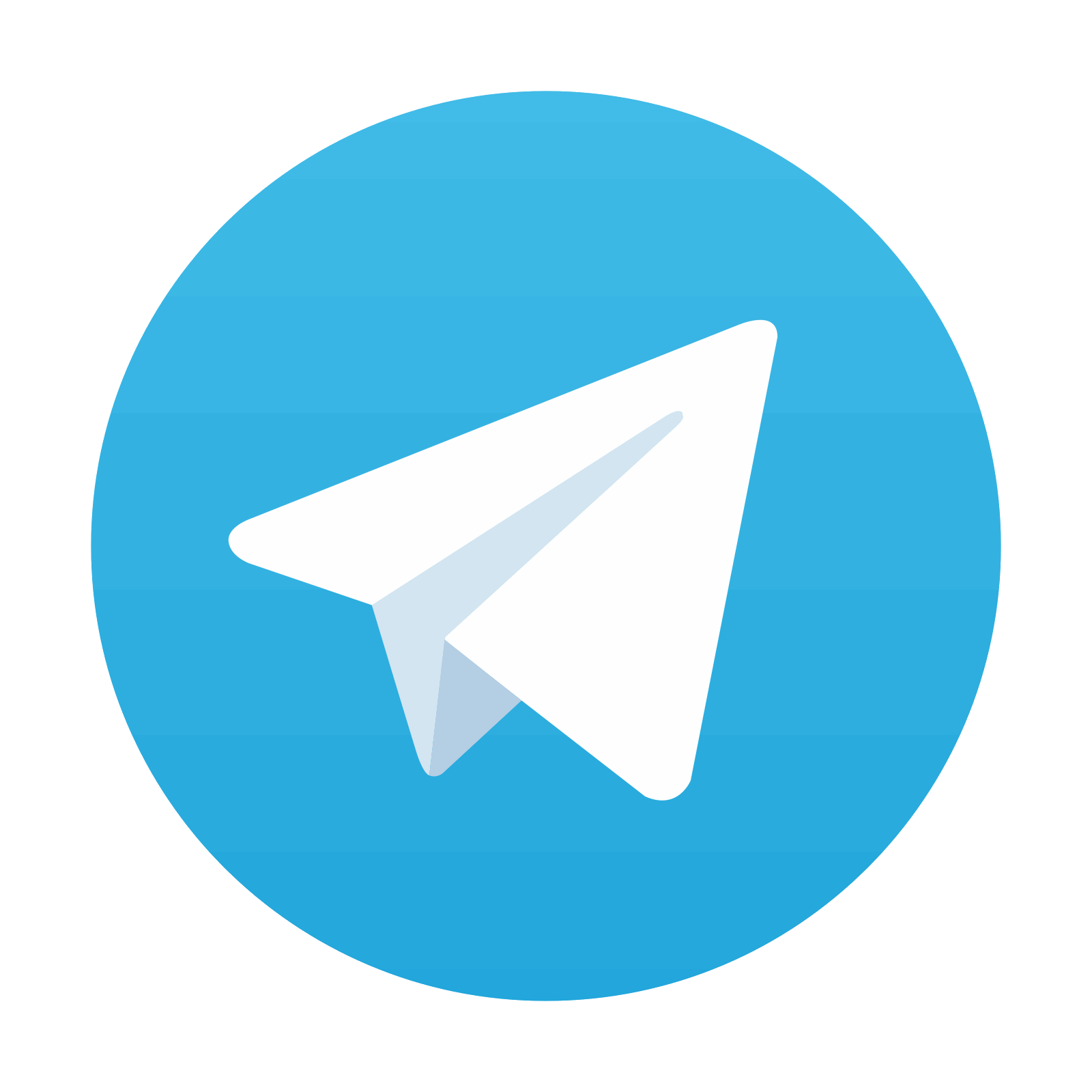
Stay updated, free articles. Join our Telegram channel

Full access? Get Clinical Tree
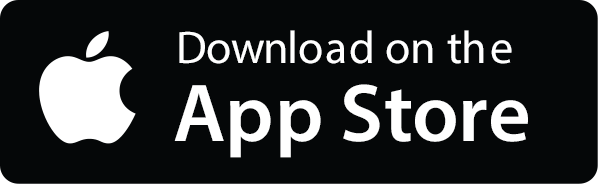
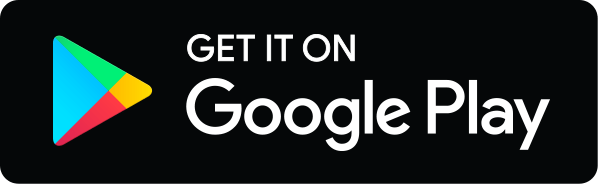