Detailed knowledge of the community of organisms in the gut has become possible in recent years because of the development of culture-independent methods. Largely based on latest DNA sequencing platforms, it is now possible to establish the composition of the microbiota and the repertoire of biochemical functions it encodes. Variations in either or both of these parameters have been linked to intestinal and extraintestinal disease. This article summarizes how these methods are applied, with special reference to gastroenterology, and describes the achievements and future potential of microbiota analysis as a diagnostic tool.
Key points
- •
Sequence-based microbiota analyses provide fast and forensically detailed insights.
- •
Costs are becoming more affordable, but true metagenomic sequencing is still prohibitively expensive for routine application.
- •
Contrary to earlier impressions, a majority of intestinal bacteria can be cultured in the laboratory, but their fastidious nature makes it challenging.
- •
Alterations in microbiota composition already show promise as biomarkers for colorectal cancer (CRC), obesity, metabolic disease, and irritable bowel syndrome.
Introduction
Studies of the community of bacteria in the human intestine have had a major impact on gastroenterology research in the last decade. As summarized elsewhere in this issue, it is now appreciated that altered microbial communities in the gut are associated with a broad range of functional gastrointestinal disorders as well as being increasingly linked to extraintestinal diseases. It is important to point out that, at the time of writing, there are no examples other than Clostridium difficile– associated diarrhea (CDAD) where an altered microbiota is responsible for a disease and where the disease can be cured by restoring a normal microbiota. There is mounting evidence, however, for microbiota involvement in obesity, inflammatory bowel disease (IBD), irritable bowel syndrome, type 2 diabetes mellitus, and metabolic syndrome, and many microbiome start-up companies are scrambling to develop first-to-market therapeutics based on these conditions. The practicing gastroenterologist, therefore, benefits from an understanding of how the microbiota is studied and what the data mean. Microbiota profiling and microbiome-based diagnostics, however, will soon reach routine clinical application, because even if altered microbiota compositions do not ultimately prove responsible for the named or other diseases, the respective microbiota changes are being validated as novel biomarkers for disease risk. The purpose of this review is, therefore, to explain how microbiota composition and function are analyzed, to outline the scale of the technical and infrastructural investment required to start microbiota analysis from scratch, to convey the powers and limits of microbiota analysis by culture-dependent and independent analysis, and finally to show the potential for clinical application of microbiome analysis to identify disease risk.
Introduction
Studies of the community of bacteria in the human intestine have had a major impact on gastroenterology research in the last decade. As summarized elsewhere in this issue, it is now appreciated that altered microbial communities in the gut are associated with a broad range of functional gastrointestinal disorders as well as being increasingly linked to extraintestinal diseases. It is important to point out that, at the time of writing, there are no examples other than Clostridium difficile– associated diarrhea (CDAD) where an altered microbiota is responsible for a disease and where the disease can be cured by restoring a normal microbiota. There is mounting evidence, however, for microbiota involvement in obesity, inflammatory bowel disease (IBD), irritable bowel syndrome, type 2 diabetes mellitus, and metabolic syndrome, and many microbiome start-up companies are scrambling to develop first-to-market therapeutics based on these conditions. The practicing gastroenterologist, therefore, benefits from an understanding of how the microbiota is studied and what the data mean. Microbiota profiling and microbiome-based diagnostics, however, will soon reach routine clinical application, because even if altered microbiota compositions do not ultimately prove responsible for the named or other diseases, the respective microbiota changes are being validated as novel biomarkers for disease risk. The purpose of this review is, therefore, to explain how microbiota composition and function are analyzed, to outline the scale of the technical and infrastructural investment required to start microbiota analysis from scratch, to convey the powers and limits of microbiota analysis by culture-dependent and independent analysis, and finally to show the potential for clinical application of microbiome analysis to identify disease risk.
Sample types, collection, transport, and storage
Many studies of the gut microbiota/gut microbiome actually examine the fecal microbiota. The main reason for this is that it is difficult to collect luminal samples or mucosal biopsies from healthy people because they would be required to undergo colonoscopy, a request that usually does not receive ethical approval. So although it is well established that the fecal microbiota is different from that of intestinal mucosa, analysis of fecal samples is a pragmatic and widely accepted compromise in many studies. Mucosal samples still provide interesting and uniquely informative data when available, such as jejunoscopy in small volunteer studies of probiotic effect on host gene transcription, rectal biopsies offering microbiome-based diagnostic potential in pediatric Crohn disease, and the presence of bacterial biofilms in right-sided colorectal biopsy tissue. If designing a microbiome study of a clinical syndrome for the first time, it is prudent to include relevant mucosal biopsy samples if possible.
Mucosal biopsy samples may be conveniently collected during colonoscopy or bowel surgery; polyps are typically too small to allow microbiota analysis in addition to routine histology requirements. Fecal samples can be collected by subjects in their own homes using a range of commercial kits that overcome the objectionable nature of this task for a majority of subjects. The authors endeavor to have the stool sample brought to the research laboratory for DNA extraction within 3 hours, without freezing, because freezing has modest but detectable effects on apparent microbiota composition.
If a microbiome study is conducted in a single facility or geographic center, freezing samples at −80°C as they are collected over time is a convenient way to bulk them and process them in batches. For studies that involve multiple clinical centers and sending samples to a single processing laboratory, the authors and other investigators have validated a commercial transport medium, which has a negligible effect on microbiota composition, providing an alternative to shipping samples on dry ice. These samples can be processed, however, for nucleic acid extraction only and currently appear unsuitable for analyses, such as metabolomics, which would still be possible with frozen whole stool.
Culture-based analysis of the gut microbiome
An often-stated advantage of culture-independent microbiota analysis is that it solves the problem of the supposed unculturability of up to 70% of the microbiota. Studies featuring engraftment of human microbes to mice via a culture step and several recent exhaustive bacteriologic culture studies, however, have shown that it is possible to culture more than 95% of microbiota members present at more than 0.1% relative abundance. These were essentially targeted studies, where the goal was to capture as much of the phylogenetic diversity of the microbiota as possible. Other studies have pragmatically focused on culturing of sufficient microbiota diversity to allow laboratory isolates to be blended in an artificial stool cocktail that effectively replaced whole feces in fecal microbiota transplantation (FMT) to successfully treat CDAD. Bacteriologic culture of fastidious anaerobic organisms is an undertaking for specialist laboratories, requiring anaerobic cabinets, dedicated gas equipment, and a level of operating skill surpassing that required for routine bacteriologic analysis in the clinic. Achieving comprehensive species coverage is also time consuming and expensive; 1 recent study used 33 different culture media. Whether or not this investment is feasible or worthwhile depends on the value to the investigator of recovering microbial taxa from the condition of interest. Fecal collection from the donor must be accomplished quickly and the stool must be moved into an anaerobic device for transport to the anaerobic cabinet in the laboratory. Whether or not the sample is made into a suspension and frozen and in what cryoprotectant requires consideration.
The significant advantage of culture-based studies is that the cultured organisms become available for biological studies and potential exploitation as therapeutics. Examples of cultured gut commensals include Faecalibacterium prausnitzii , which was identified as being depleted in the microbiota of IBD patients and is being developed as a therapeutic, and Eubacterium hallii , which is less abundant in subjects with metabolic syndrome but is restored during an FMT that provides some restoration of insulin sensitivity. Successful culture of fastidious anaerobes will add to the increasing commercial interest in exploiting the microbiome for novel therapeutics, evidenced by the numbers of new start-up companies in this area.
DNA extraction methods
Choosing an appropriate DNA extraction technique is critical for successful analysis of microbial ecosystems using next-generation sequencing workflows. To achieve optimal cell disruption, particularly of archaeal and gram-positive bacterial cell walls, a mechanical lysis step needs to be included in the protocol. This usually involves the addition of glass or silica beads of various sizes (0.1 mm, 1 mm, and 4–5 mm) to fecal samples and homogenizing them with a bead-beater device. Different extraction methods can lead to different sequencing results, so it is thus important to use the same protocol for all samples in a study. The International Human Microbiome Standards project ( http://www.microbiome-standards.org/ ) aims to define the best extraction method for fecal samples. The outcome will be important for the research community because it will provide valid recommendations for standardized protocols, which are a prerequisite for applying outcomes to clinical settings. Many different extraction methods are currently available and several companies sell nucleic acid extraction kits optimized for various sample types, such as stool, saliva, tissue, or skin. The general principles for extraction of nucleic acids from such different tissue types are similar but, for example, stool contains many polymerase chain reaction (PCR) inhibitors that are effectively removed with dedicated commercial products.
Microbiota composition determination
The simplest and cheapest way to study the microbiota is to generate a list of the bacteria present by sequencing a pool of amplicons generated by a PCR reaction targeting a region of the 16S ribosomal RNA gene. This gene is present in all cells (including in mammalian cells where it is called 18S) and it encodes an RNA strand that forms part of the ribosome involved in transcript binding to initiate translation. An adaptation of the protocol is to hybridize the amplicon pool to a chip bearing oligonucleotides specific for individual bacterial taxa, and this protocol seems to yield substantially similar data to amplicon sequencing, although it is more laborious and it is slower throughput compared with current sequencing technologies. Most investigators use the same universal primers for all samples, purify the PCR product, and perform a second index PCR with a primer pair featuring a unique barcode identifier in the primer for each sample. These amplicons are then purified and mixed in equimolar amounts for sequencing on a massively parallel instrument.
A vast array of 16S amplification primers and protocols for amplicon sequencing have been developed in multiple laboratories over the past decade, often accompanying changes in the dominant sequencing platform and the read-length available. For a new user investigating the microbiome in a clinical context, there is a bewildering choice of protocols available. For a detailed technical analysis of current options and protocol recommendations, readers are referred to an excellent study by Gohl and colleagues and an imminent publication from the International Human Microbiome Standards project ( http://www.microbiome-standards.org/ ).
Currently the most popular sequencing platform is probably the Illumina MiSeq (Illumina, San Diego, California, USA), which offers 2 × 300 base pair paired end reads and a run cost for 384 samples that brings the pure sequencing cost to below €10 per sample. This platform was recently used in a demonstration of how microbiome analysis can be accelerated to a 48-hour test. In practice, in the authors’ laboratory, 285 samples per run is not exceeded, and the per-sample cost does not include reagent, primer, and staff costs for amplicon preparation. Nevertheless, compared with 10 years ago and the original pyrosequencing protocols, this is a huge cost improvement.
No amplicon sequencing methods are completely free of bias and the best solution is to apply shotgun metagenomics sequencing (discussed later). This is a more challenging prospect for new users because the per-sample cost may be 20 to 30 times higher than amplicon sequencing, the data sets are much larger, and current significant data storage and analysis present challenges.
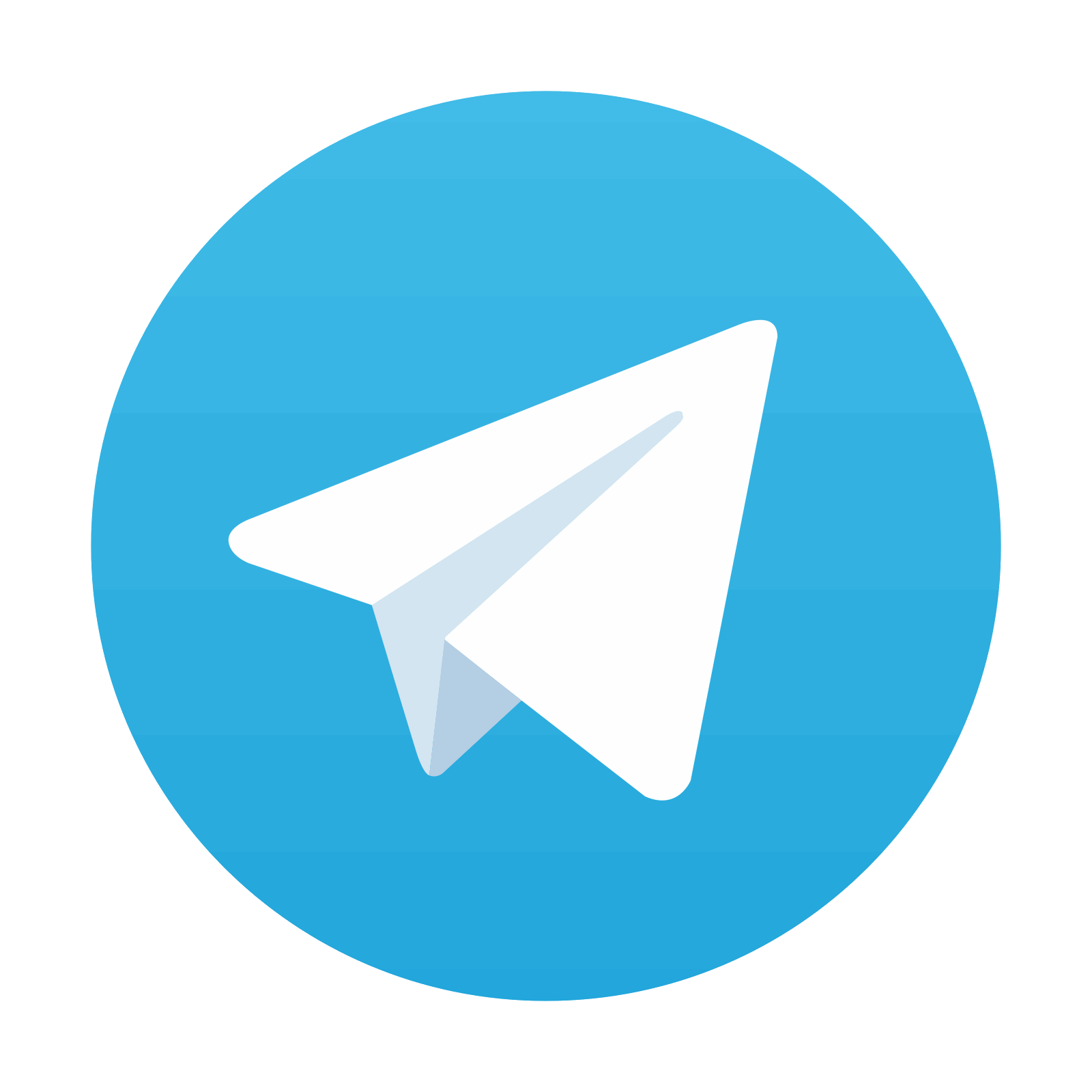
Stay updated, free articles. Join our Telegram channel

Full access? Get Clinical Tree
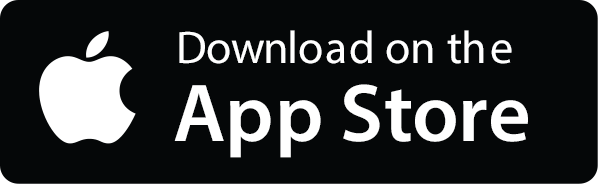
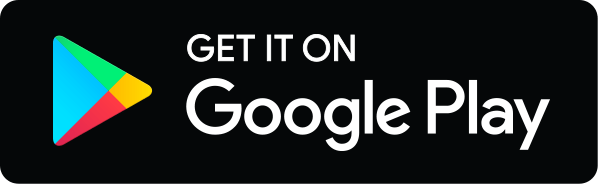