Risk classification
Stage
PSA
Gleason
Low risk
T1-2a
≤10 ng/ml
≤6
Intermediated risk
T2b
>10 and ≤ 20 ng/ml
7
High risk
T2c-3a
>20 ng/ml
≥ 8
The aim of focal therapy is to treat cancer within the prostate, whilst leaving benign prostate and surrounding normal structures intact, in order to offer oncological control with minimum impact on genitourinary and rectal function. This concept is not forgetting the multifocal nature of PC but is based on the assumption that only the index lesion is eventually responsible for severe consequences for patients’ life; the remaining lesions are over-treated as they could be successfully managed conservatively. As a consequence, a variety of focal therapies have been proposed and are under clinical evaluation (Laser, CryoAblation, etc.). High-Intensity Focused Ultrasound (HIFU) is emerging as an alternative to active surveillance for the management of low risk of prostate cancer, noninvasively. It represents an innovative technique that may selectively ablate known disease while preserving existing functions [12]. The favorable physical interaction between ultrasound (US) waves and biological tissue promises some very interesting and unique therapeutic approaches. In fact, the conservative approach (sparing also the neurovascular bundle) leads to a low rate of genitourinary side effects. HIFU features high energy ultrasound focused to thermally ablate target tissue by raising the local temperature over 60 °C, leading to coagulative necrosis [13]. First HIFU ablation of PC have been performed under conventional ultrasound (US) guidance [12, 14, 15] more than 15 years ago; at present, HIFU has been presented as an alternative therapeutic options for localized PC in the Guidelines of American Urological Association (AUA) in 2007 [5] and of European Association of Urology (EAU) in 2008 [16]. Recently, Magnetic Resonance Imaging (MRI) has been proposed as a guidance and monitoring modality (Magnetic Resonance guided Focused Ultrasound Surgery—MRgFUS) [17], introducing some relevant advantages over other imaging options, especially superior anatomical resolution and tumor detection, thanks to dynamic contrast enhanced imaging (DCE-MRI), spectroscopy, Diffusion-Weighted Imaging (DWI) [18–20], and fast thermal mapping with the proton-frequency shift (PRF) method, to control temperature rising and confirm ablation in real time during the treatment[21]. MRI can be also performed as posttreatment monitoring technique for immediate evaluation of procedure efficacy [22, 23].
11.3 Focused Ultrasound Principles
High-intensity focused ultrasound therapy is a novel, emerging, therapeutic modality that uses ultrasound waves, propagated through tissue media, as carriers of energy. This completely noninvasive technology has great potential for tumor ablation as well as hemostasis, thrombolysis, and targeted drug/gene delivery [24]. Ultrasound is a pressure wave with a frequency above the audible range of a human ear (18–20 kHz); it is generated by a mechanical motion that induces the molecules in a medium to oscillate around their rest positions. The motion causes compressions and rarefactions of the medium and thus a pressure wave travels with the mechanical disturbance. As a result, an ultrasound wave requires a medium for propagation. Ultrasound is generated by applying radiofrequency (RF) voltage across a material that is piezoelectric, i.e., it expands and contracts in proportion to the applied voltage. For many applications of ultrasound therapy, transducers capable of producing high-power, single-frequency, and continuous waves are needed. In order to be able to use ultrasound for therapy, it is essential to know the ultrasonic properties of tissues. The temperature elevation induced at the focus is partially dependent on the ultrasound attenuation, while the beam propagates through the overlying tissues, and the tissue absorption coefficient at the target site. The speed of ultrasound is not frequency dependent and has a similar average magnitude of 1,550 m/s in all soft tissues (excluding lung). The velocity in fatty tissues is less than that in other soft tissues, being about 1,480 m/s while in the lungs the air spaces reduce the velocity to about 600 m/s. The highest values have been measured in bones, between 1,800 and 3,700 m/s depending on the density, structure, and frequency of the wave. The effect of the temperature-dependent sound speed is small on the field shape and can be ignored when sharply focused fields are used. Ultrasonic attenuation in tissues is a sum of the losses due to absorption and scattering, and it determines the penetration of the beam into the tissue. Ultrasound interacts with tissue through the particle motion and pressure variation associated with wave propagation. First, all ultrasound waves are continuously losing energy through absorption resulting in an increase in temperature within the tissue. If the temperature elevation is large enough and is maintained for an adequate period, the exposure causes tissue damage. This thermal effect that can be used for tissue coagulation or ablation is similar to that obtained using other heating methods with equal thermal exposure. Second, at high-pressure amplitudes, the pressure wave can cause formation of small gas bubbles that concentrate acoustic energy. This type of interaction between a sound wave and a gas body is called cavitation and it can cause a multitude of bioeffects from cell membrane permeability changes to complete destruction of tissue. Finally, the mechanical stress and strain associated with wave propagation may sometimes cause direct changes in a biological system. The thermal effects produced by ultrasound have been utilized in hyperthermia as a cancer therapy as well as in many ultrasound surgery applications. In order to induce thermal tissue damage, the exposure at a given temperature has to exceed a threshold time below which the tissue recovers. The thermal damage threshold depends among other things on tissue type and physiological factors (pH and O2). The temperature elevation in a tissue depends on the absorption and attenuation coefficients of the tissue, the size and shape of the ultrasound field (thermal conduction effects), and also strongly on the local blood perfusion rate. For HIFU ablation, a piezoelectric transducer with a typical center frequency of between 1 and 7 MHz is used to generate a focused ultrasound field. This field is coupled into the body and aimed at the target region. By propagation through the tissue, the US wave will be absorbed and the acoustic energy will be transformed to heat. Inside the focal zone the high energy density will cause temperature increases to more than 60 °C within seconds, and the tissue proteins to coagulate. The surrounding tissue and the overlying structures remain unaffected due to the low acoustic energy density in this area [15] (Fig. 11.1). Combining MR imaging to define the target and to control and monitor the ablation, and an ultrasound transducer that controls and delivers the focused ultrasound beam, MRgFUS allows a noninvasive approach to the treatment of many malignant or not pathologies. At present, MRI is one of the most accurate techniques to investigate local anatomy of male pelvis in detail and is particularly helpful for localization and staging of PC. Traditional prostate MRI has been based on morphologic imaging with standard T1-weighted and T2-weighted sequences, which has limited accuracy. Recent advances including DWI and perfusion imaging allow extension of the obtainable information beyond anatomic assessment [18]. All these techniques have shown their potential value in distinguishing malignant from benign PC; however, none of them used alone is capable of optimally characterizing tumors in the prostate [25]. For guiding and monitoring HIFU ablation, MRI could be considered more accurate than US as it offers clear advantages: first, it provides high-resolution imaging in any orientation for planning treatment and evaluating relative effects thanks to soft tissue contrast; second, MRI is the only currently available technique with proven capabilities to create quantitative temperature maps. MR-based thermal imaging provides a means to ensure that the proper ultrasound exposures are being applied for a safe and effective ablation on the target volume without effects to surrounding tissues [23, 26]. Magnetic resonance PRF thermometry allows noninvasive temperature monitoring during ultrasound thermal ablation. The method uses the temperature dependence of the PRF which can be determined from the phase in gradient echo images [26, 27]. In order to provide volumetric and rapid thermometry, the acquisition sequences are multislice, gradient-recalled, single-shot, echo-planar imaging (EPI). Moreover, developments in MR imaging hardware and software (3-T vs. 1.5-T imaging) continue to improve spatial and temporal resolution and the signal-to-noise ratio of MR imaging examinations [19].
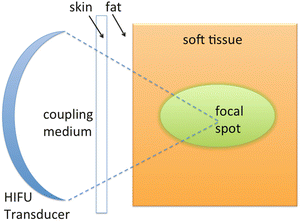
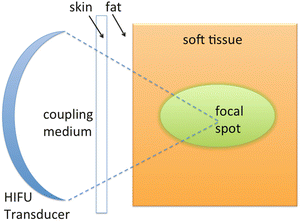
Fig. 11.1
Schematic representation of piezoelectric transducer used to generate a focused ultrasound field. The US wave is absorbed and the acoustic energy is transformed to heat in the focal zone, that rise in temperature over 60 °C. The surrounding tissue and the overlying structures remain unaffected thanks to the low acoustic energy density in this area
11.4 MRgFUS: Our Experience
After the wide experience obtained with US-guided HIFU all over the world in the last 15 years, MRgFUS has been successfully tested in small patients groups for the focal therapy of prostate cancer in the last years. At present, in our Department, six patients with unifocal, biopsy proven PC, indicated to RP, underwent MRgFUS prior to surgery in a phase I, controlled trial. All patients included in this study had localized disease (stage T1–T2, Nx–N0, M0), Gleason score 6 (3 + 3), PSA level less than 10 ng/ml, and evidence of cancer lesions on MRI. Target lesions were identified with Turbo Spin Echo T2-weighted, DCE T1-weighted, and DWI sequences and served as planning for subsequent MRgFUS ablation (Fig. 11.2). Patients with a Gleason score ≥8, multifocal or bilateral prostate cancer, with a previous pelvic or rectal cancer, and with an American anesthesiological (ASA) score ≥3 were excluded from the study. All subjects underwent MRgFUS treatment and a subsequent open radical prostatectomy within 2 weeks.