Abstract
Our gastrointestinal tract is innervated by specific classes of extrinsic sensory afferents, which follow either vagal or spinal pathways. Vagal afferents have cell bodies located within the nodose or jugular ganglia and project centrally to the brainstem. In contrast, spinal afferents have cell bodies in thoracic or sacral dorsal root ganglia and project centrally to the dorsal horn of the spinal cord. Together, these afferent pathways detect ongoing changes in the physical and chemical environment within the lumen and wall of our gastrointestinal tract. Consequently, these afferents convey the full spectrum of information that underlies the initiation of autonomic reflex control and all manner of visceral sensations, including hunger, fullness and urge. In pathological states these afferents also represent the underlying basis of ongoing symptoms such as bloating, discomfort and chronic visceral pain. This chapter will map out the anatomy and function of specific classes of sensory afferents innervating the gastrointestinal tract. Furthermore, it will highlight the key molecular mechanisms underlying the hypersensitivity of extrinsic sensory afferents innervating the gastrointestinal tract, which provides potential opportunities for the therapeutic treatment of gastrointestinal disorders.
Keywords
Afferents, Pain, Nociceptors, Ion channels, Receptors, Inflammation, Infection, Hypersensitivity, Colon, Stomach, Intestine, Irritable bowel syndrome
Abbreviations
4-HNE
4-hydroxynonenal
5-HT-R
5-HT receptor
ASIC1
acid sensing ion channel 1
ASIC2
acid sensing ion channel 2
ASIC3
acid sensing ion channel 3
CGRP
calcitonin gene-related peptide
CNS
central nervous system
CRD
colorectal balloon distension
CVH
chronic visceral hypersensitivity
CVP
chronic visceral pain
DH
dorsal horn
DRG
dorsal root ganglia
DSS
dextran sodium sulfate
GABA
γ-aminobutyric acid
GABA B
γ-aminobutyric acid receptor B
GPCR
G-protein coupled receptor
GRK6
G protein coupled receptor kinase 6
H 1 R
histamine 1 receptor
H 4 R
histamine 4 receptor
IBD
inflammatory bowel disease
IBS
irritable bowel syndrome
IBS-A/M
irritable bowel syndrome with alternating or mixed motility
IBS-C
constipation-predominant irritable bowel syndrome
IBS-D
diarrhea-predominant irritable bowel syndrome
IGLEs
intraganglionic laminar endings
IL-1β
interleukin-1β
IL-6
interleukin-6
IMAs
intramuscular arrays
MIA
mechanically insensitive afferents
MPO
myeloperoxidase
Na V
voltage-gated sodium channel
NTS
nucleus of the solitary tract
OTR
oxytocin receptor
PAR-2
protease-activated receptor 2
PARs
protease-activated receptors
PBMCs
peripheral blood mononuclear cells
PBn
parabrachial nucleus
PI-IBS
postinfectious irritable bowel syndrome
TLESRs
transient lower esophageal sphincter relaxations
TNBS
trinitrobenzene sulfonic acid
TNF-α
tumor necrosis factor-α
TRP
transient receptor potential
TRPA
transient receptor potential ankyrin repeat
TRPM
transient receptor potential (TRP) melastatin related
TRPV
transient receptor potential (TRP) vanilloid binding
VMR
visceromotor response
VPL
ventral posterolateral
δ-OPR
delta-opioid receptor
κ-OPR
kappa-opioid receptor
μ-OPR
mu-opioid receptor
Acknowledgments
Stuart M. Brierley is a National Health and Medical Research Council of Australia (NHMRC), R.D Wright Biomedical Research Fellow ( APP1126378 ). Andrea M. Harrington received funding via the Australian Research Council (ARC) Discovery Early Career Research Award (DE130100223).
17.1
Introduction
Our ability to detect and perceive mechanical and chemical stimuli is vital in determining how we respond to environmental challenges. Detection of external stimuli by sensory afferents innervating our skin and joints is responsible for our senses of touch, proprioception, and aspects of pain. While we may not always be aware of it, specific classes of extrinsic sensory afferents also detect ongoing changes in the physical and chemical environment within the lumen and wall of our gastrointestinal tract. These sensory afferents are subdivided on the location of their soma. Vagal afferents have cell bodies located within the nodose/jugular ganglia and project centrally to the brainstem, while spinal afferents have cell bodies in multiple dorsal root ganglia (DRG) and project centrally to the dorsal horn of the spinal cord. These afferents convey information that underlies the initiation of autonomic reflex control and all manner of visceral sensations, including chronic visceral pain (CVP). This chapter has several aims: (1) To map out the anatomy of the sensory afferents innervating the gastrointestinal tract. (2) Summarize the key functional classes of afferent endings located throughout the gastrointestinal tract. (3) How the function of these afferents is altered in clinically relevant disease states. (4) Highlight the key molecular and cellular peripheral markers that are altered in samples from patients with clinical gastrointestinal disorders. (5) Highlight the key ion channels and receptors that are expressed by extrinsic sensory afferents innervating the gastrointestinal tract that underlie afferent hypersensitivity, but serendipitously present potential opportunities for the therapeutic treatment of CVP.
17.2
Anatomy of the Extrinsic Sensory Afferent Nerves Innervating the Gastrointestinal Tract
17.2.1
Vagal Afferents
The cell bodies of vagal afferents reside in either the bilateral nodose ganglia or the bilateral jugular ganglia, located at the base of the skull ( Fig. 17.1 ). The nodose and jugular ganglia are embryologically distinct, with nodose neurons arising from the epibranchial placodes, while jugular neurons derive from neural crest cells of the postotic hindbrain, more akin to spinal afferents. The central projections of gastric vagal afferents have their terminals predominantly within the subnucleus gelatinosus of the nucleus of the solitary tract (NTS). Within the NTS, esophageal afferents project to the subnucleus centralis, whereas afferents innervating the gastric mucosa and muscle project to overlapping regions of the NTS. Additionally, there is a small proportion of mucosal afferents that project into the medial subnucleus. Overall, there are diverse projections from the NTS into a large range of nuclei throughout the brain, which are involved with behavioral, cognitive, and autonomic processing. The axons of vagal afferents travel via the paired dorsal and ventral vagal nerve trunks before organ-specific branches project into thoracic and abdominal organs, including the esophagus, stomach, and small intestine. The density of vagal afferent endings is greatest within the stomach and esophagus, reduces in the small intestine, and are absent in the distal colon and rectum. Vagal afferents innervate all layers of the wall of the upper gastrointestinal tract. They include various types of mucosal endings, intraganglionic laminar endings (IGLEs), and intramuscular arrays (IMAs), which are discussed in detail below. In keeping with their distribution and function, vagal afferents are generally associated with signaling physiological events in the upper gastrointestinal tract, such as fullness and satiety.

17.2.2
Spinal Afferents
The cell bodies of spinal afferents are located within the cervical, thoracic, lumbar, and sacral DRG, located bilaterally adjacent to the spinal cord. The precise regional location of the DRG depends upon the gastrointestinal region. The spinal afferent innervation of the gastroesophageal region spans from the cervical to the thoracic and upper lumbar segment of the spinal cord via axons traveling through the thoracic spinal and greater splanchnic nerves ( Fig. 17.1 ). The spinal afferent innervation of the small intestine spans from the thoracic to the lumbar spinal cord via the greater splanchnic nerves ( Fig. 17.1 ). The proximal colon receives spinal innervation from the thoracic to the lumbar spinal cord via the lumbar splanchnic nerve, while the distal colon receives dual innervation from the lumbar splanchnic nerve and sacral pelvic nerves. The rectum also receives innervation from the lumbosacral spinal cord via the sacral pelvic nerves ( Fig. 17.1 ). Spinal afferents innervate all layers of the wall of the gastrointestinal tract. They include various types of mucosal endings, IGLEs, IMAs, and in particular afferents that wrap around blood vessels, which are discussed in detail below.
The central terminals of spinal afferents project into discrete laminae of the superficial dorsal horn and make synaptic contacts with second-order neurons within the dorsal horn of the spinal cord. Spinal afferents supplying the upper gastrointestinal tract, such as the esophagus and small intestine, are distributed along the cervical and thoracic spinal cord (C1-T12), with peaks of innervation present in C2-C6, T2-T4, and T8-T12. Lumbar splanchnic fibers innervating the colon terminate within the thoracolumbar spinal cord (T10-L1), whereas sacral pelvic afferents innervating the distal colon and rectum terminate within the lumbosacral spinal cord (L6-S2). The central terminals of afferents innervating the distal colon are localized within the thoracolumbar spinal cord. They project into the superficial dorsal horn lamina I and transverse along mid and lateral collateral pathways of Lissauer into lamina V. The projection pattern of pelvic nerves in the lumbosacral spinal cord differs from that observed in the thoracolumbar spinal cord for the splanchnic nerves, with studies showing terminals present in the lumbosacral superficial dorsal horn lamina I and more prominently in Lissauer’s lateral collateral tracts projecting into laminae V and VI and medial tracts projecting into the dorsal commissure and spinal dorsal columns. The types of dorsal horn neurons activated by visceral stimuli differ between spinal segments. Within the thoracolumbar spinal cord, lamina I projection neurons and interneurons are activated by visceral stimuli. In the lumbosacral spinal cord, a greater number of interneurons and dorsal column neurons are responsive to colorectal distension relative to that observed in the thoracolumbar spinal cord. From the spinal cord, dorsal horn lamina I projection neurons relay visceral specific sensory information to higher central nervous system (CNS) centers via the ascending spinothalamic tracts. In addition, lamina I spinoparabrachial projection neurons relay visceral input into the parabrachial nucleus (PBn), which transmits to higher-order centers for nociceptive processing. The dorsal column is also important in the transmission of visceral pain, with postsynaptic spinal dorsal column neurons activated by visceral stimuli and transferring input into the ventral posterolateral (VPL) nucleus of the thalamus. Dorsal column neurons responsive to visceral stimuli are prominent in the lumbosacral spinal cord, located in laminae III-IV, and around the central canal area. Given their distribution throughout the gastrointestinal tract and their function, spinal afferents are generally associated with higher-threshold sensations such as discomfort, bloating, urgency, and pain.
17.3
Anatomy and Function of the Peripheral Endings of Gastrointestinal Afferents
Vagal and spinal extrinsic sensory afferents innervating the gastrointestinal tract have been studied for decades in a variety of animal species including mice, rats, ferrets, cats, guinea pigs, sheep, rabbits, and also humans. Generally speaking, these vagal and spinal afferents have been recorded and subdivided on the basis of their mechanical responsiveness to a variety of stimuli, their mechanical activation thresholds, and their perceived location within the various layers of the gastrointestinal tract. In this chapter, for simplicity, we highlight five main functional classes of afferents, which include (1) low-threshold mucosal afferents that are highly sensitive to distortion of the gastrointestinal mucosa, (2) muscular (or tension) afferents that respond with a wide dynamic range to circular stretch/distension, (3) muscular/mucosal (or tension/mucosal) afferents that respond to both mucosal distortion and circular stretch, and (4) vascular endings that wrap around blood vessels in the mesentery, serosal, and submucosal space and respond to high-threshold stimuli ( Fig. 17.2 ). Notably, these four different classes of afferent have been confirmed to be present in recordings from human colon ( Fig. 17.3 ), which highlights the utility of preclinical studies. The fifth class, termed mechanically insensitive afferents (MIA) or silent afferents, gain the ability to respond to mechanical stimuli following activation by inflammatory or immune mediators. As discussed below, the majority of these afferent classes have conduction velocities in the C-fiber range and are predominantly peptidergic, while many afferent endings display specialized anatomical structures, rather than the traditionally perceived “free nerve endings” ( Fig. 17.4 ). These terminal structures, combined with a various arsenal of ion channels and receptors to regulate their neuronal excitability, underlie the vastly different sensory functions of spinal afferent classes.



17.4
Mucosal Afferent Endings
Vagal and spinal mucosal afferents are sensitive to light stroking or compression of the gastrointestinal mucosa. They generate a brief burst of action potentials each time the stimulus passes over its associated receptive field. Mucosal afferents constitute half of afferents in the upper gastrointestinal tract, and are generally silent at rest. They are not sensitive to distension or contraction of the gut, and given their exquisite sensitivity to low-threshold stimuli, they play a crucial role in detecting the particle size of luminal contents. Within the esophagus, mucosal afferents may provide feedback to reflexes controlling peristaltic contraction, while in the stomach and small intestine they likely regulate the initiation of satiety, nausea, vomiting, as well as regulating gastric emptying. Within the colon, mucosal afferents are rare, representing only 4%–23% of the total fibers recorded from the splanchnic nerves of mouse and rat. In the distal colon and rectum, mucosal afferents are more frequently observed in the pelvic innervation, representing 23%–38% of all afferents recorded. This increased role of mucosal afferents in the distal colon and rectum is important, due to a need for information about stool consistency as material passes distally. Accordingly, pelvic mucosal afferents are likely to be involved in the control of defecation and likely contribute to conscious perception of stool passage in the rectum and anal canal. The anatomy of mucosal afferents from the pelvic nerve has recently been identified in the distal colon of mice, with 11% of all the pelvic afferent endings projecting into the mucosa. These fibers consist of single or branched fine varicose axons that ramify within the mucosa, with the vast majority (> 90%) of mucosal endings found to be calcitonin gene-related peptide (CGRP) positive.
17.5
Tension or Muscular Afferents
Gastrointestinal afferents that are responsive to low-intensity stretch, distension, or contractions have been referred to as tension (vagal gastroesophageal), low-threshold and wide-dynamic range (jejunal afferents), or muscular (spinal colonic afferents). Vagal afferents in the upper gastrointestinal tract often have a resting discharge of action potentials that is increased in response to contractions and distension. Accordingly, tension afferents signal the amplitude, pattern, and direction of luminal contractions to the CNS, which is important in triggering reflexes controlling gastrointestinal function. Anterograde tracing studies have identified IGLEs as the anatomical equivalent of the tension receptor. IGLEs are lamellar structures that originate from a parent axon and extend into and cover the myenteric ganglia. Anatomically, IMAs have also been identified, which are specialized structures within the circular and longitudinal muscle layers that range in size from several hundred microns to several millimeters. Accordingly, IMAs have been suggested to be tension receptors, length receptors, or a combination of both. Although these endings are likely mechanosensitive, little physiological evidence exists for their actual function.
In the jejunum, three different subtypes of vagal or spinal afferent can be classified on the basis of their response to distension. They are termed low-threshold, wide-dynamic range, and high-threshold afferents. In terms of the afferents responsive to low-intensity distension, the low-threshold afferents probably represent vagal afferents, with the wide-dynamic range afferents representing spinal afferents. However, to date, their anatomy remains to be conclusively determined.
In the colon and rectum, muscular afferents respond to low-distension pressures (e.g., < 20 mmHg; or low-intensity stretch stimuli (< 3 g; ), within the physiological range caused by the propulsion of fecal matter. Muscular afferents are prevalent in the pelvic pathway (21% of all mechanosensitive afferents) and are found in both the distal colon and rectum. In contrast, muscular afferents are relatively rare in the splanchnic pathway (10% of all mechanosensitive afferents). Pelvic muscular afferents also have slower adaptation rates to maintained distension. These afferents recorded in ex vivo mouse preparations are similar to those recorded previously in vivo in the pelvic nerve of cats and rats and in the splanchnic nerves of cats. The anatomical transduction sites of rectal muscular afferents have been identified as flattened branching endings in the myenteric ganglia called rectal IGLEs. They are morphologically similar to vagal IGLEs, but are smaller, simpler, and display less extensive branching. As muscular afferents are responsive to contraction of both the circular and longitudinal muscle they are likely excited by physiological levels of distension in the distal colon and rectum that provide information that is important for reflex loops and defecatory pathways.
17.6
Tension/Mucosal or Muscular/Mucosal Afferents
While distinct classes of mucosal and muscular afferents have been clearly identified, there is a separate class of afferent that respond to both light mucosal distortion and by low-threshold distension or contraction. These afferents have been termed tension/mucosal in the upper gut, and muscular/mucosal in the distal colon and rectum. The vagal tension/mucosal afferents have been observed in the gastroesophageal regions of the ferret. These vagal afferents may have a specialized role in the detection of rapidly moving boluses of food and/or liquid along the esophagus. Spinal muscular/mucosal afferents have only been localized in the pelvic innervation of the distal colon and rectum of the mouse. They represent ~ 25% of the total pelvic innervation and respond in the same way as both mucosal and muscular afferents. Interestingly, these afferents have not been observed in the splanchnic innervation of the distal colon, suggesting that they may play a key role in spinal defecatory circuits and conscious sensation. Recent tracing studies have identified a remarkably complex array of different morphological types of pelvic afferent endings in many anatomical layers of the colorectum. While it has yet to be confirmed, there are identified endings within the submucosal ganglia, and innervating the Crypts of Lieburkuhn, that may be the anatomical correlate of muscular/mucosal afferents.
17.7
Vascular, Serosal, and Mesenteric Afferent Endings
Afferents with receptive fields on the mesenteric attachment and serosa were originally described several decades ago from recordings from the splanchnic nerve. These studies showed that mesenteric afferents responded to punctate mechanical stimulation of the mesentery or stretch of the mesenteric attachment and may have up to seven punctate receptive fields depending on the species recorded from. Mesenteric afferents are also capable of responding to distension with an adapting response, particularly at similar noxious levels of distension, and may therefore serve a role in signaling mechanically induced pain. Interestingly, mesenteric afferents appear specific to the splanchnic nerve, where they represent ~ 50% of all mechanosensitive afferents, and have not been identified from the pelvic innervation.
When they were first described in the splanchnic nerves, serosal afferents were not distinguished from mesenteric afferents in any way other than by the locations of the receptive fields. However, recent studies have shown they are distinct classes of afferent, with the term “serosal” a misnomer, as the anatomical correlate of serosal afferents resides along blood vessels, including those within the submucosa. Accordingly, these afferents have been referred to as serosal, vascular, high-threshold, and nociceptors in various studies. Vascular/serosal afferents are generally unresponsive to low-threshold stimuli and respond to much higher intensities of distension (> 40 mmHg; or stretch (< 9 g; ). These physiological properties match the original in vivo reports of high-threshold afferents in the esophagus and colon. Accordingly, these vascular/serosal and mesenteric afferents display the properties of specific high-threshold mechanonociceptors. Unlike mesenteric afferents, serosal afferents are common to both the splanchnic and pelvic pathways, representing ~ 33% of all the mechanosensitive afferents in these respective pathways. As discussed in detail below, these vascular/serosal high-threshold afferents are polymodal and respond to a wide variety of inflammatory and immune mediators and are sensitized in inflammatory and chronic visceral hypersensitivity (CVH) states.
17.8
Mechanically Insensitive or Silent Afferents
There are numerous reports of afferents that were previously insensitive to mechanical stimuli that become responsive to mechanical stimuli following exposure to chemical and inflammatory mediators. Recent studies suggest that ~ 25% of all splanchnic and pelvic afferents innervating the distal colon and rectum are mechanically insensitive in naïve preparations from mice. Subsequent zymosan treatment leads to a decrease in the proportion of “silent” afferents at short- and long-term time points, and a corresponding increase in the proportion of mechanically sensitive high-threshold vascular/serosal afferents. There is also evidence for MIA that respond to bradykinin, ATP, or capsaicin in the mouse splanchnic nerve, but do not subsequently become mechanosensitive. Their role is unclear, but they may serve as specific chemoreceptor afferents. These observations suggest that mechanosensory proteins may exist in vascular/serosal afferent endings that can become functional after inflammatory insult, inducing mechanosensitivity. The increase in the proportion of vascular/serosal afferents following insult may contribute to an increased afferent barrage from the periphery in response to distension and contraction, resulting in persistent pain states as discussed below.
17.9
Clinical Relevance of the Extrinsic Sensory Afferent Pathways Innervating the Gastrointestinal Tract
Inflammation of the gut, either through gut infection or abnormal immune responses, has been regularly demonstrated to cause abnormal neuronal function. These profound effects result in dysregulated neuronal signaling, abnormal secretion, motility and sensory signaling, resulting in the development of diarrhea, constipation, discomfort and pain. The importance of such changes is highlighted in a number of highly prevalent organic and functional gastrointestinal disorders. In organic disorders such as inflammatory bowel disease (IBD), which includes Crohn’s disease and ulcerative colitis, chronic uncontrolled inflammation of the intestinal mucosa is recognized as the pathogenesis of neuronal dysfunction and correspondingly the presentation of symptoms. However, for functional bowel disorders, such as functional dyspepsia and irritable bowel syndrome (IBS), where macroscopic mucosal damage is not evident, but symptoms of persistent abdominal pain, discomfort, and abnormal bowel function are evident the pathogenesis is less clear. IBS is characterized by chronic abdominal pain and is subclassified on the basis of altered bowel habit. This classification consists of IBS with constipation (IBS-C), IBS with diarrhea (IBS-D), IBS with alternating or mixed motility (IBS-A/M), and postinfectious IBS (PI-IBS). While the etiology of IBS is multifactorial and additional risk factors may also be required for development, there is a strong correlation between a prior exposure of the patient to gut infection and symptom occurrence. Numerous clinical studies have attributed IBS symptom development to a preceding bout of gastroenteritis induced by pathogens such as Campylobacter, Escherichia coli , Giardia lamblia, and Salmonella . Importantly, it is the duration and severity of the initial illness that is one of the strongest associated risk factors for the development of IBS symptoms. Such bouts of acute gastroenteritis can trigger IBS symptoms in patients that persist for greater than 8 years after the initial infection. The potential reasons for these persistent symptoms are becoming clearer from samples from IBS patients and from mechanistic preclinical studies.
There is increasing evidence demonstrating that subgroups of IBS patient display altered immunological function and persistent low-grade inflammation within the gut wall. For example, colonic biopsy samples collected from IBS patients display greater mast cell infiltration and an increased release of key mediators, particularly histamine and tryptase. This increase in mast cell numbers correlates with the severity and frequency of abdominal pain and discomfort, due to these activated mast cells being in closer proximity to afferent nerve fibers. Correspondingly, supernatants from IBS patient biopsies, but not healthy subjects, cause activation of afferent nerve endings and their cell bodies. Additional studies demonstrate that mucosal biopsy samples from IBS patients also display increased levels of the proinflammatory cytokine interleukin (IL)-1β and increased number of intraepithelial lymphocytes. Additional changes are also evident in the peripheral blood mononuclear cells (PBMCs) from IBS patients. In particular, the proinflammatory cytokines, tumor necrosis factor-α (TNF-α), IL-1β, and IL-6, are all increased in PBMC supernatants from IBS-D patients ( Fig. 17.5 ). These increases also correlate with symptoms of abdominal pain intensity and frequency. Importantly, when these PBMC supernatants from IBS-D patients, or the individual cytokines TNF-α, IL-1β, and IL-6, were tested on mouse colonic afferents, they evoked pronounced mechanical hypersensitivity in both low- and high-threshold afferent subtypes. This hypersensitivity is dependent upon TRPA1, but not TRPV1-dependent mechanisms ( Fig. 17.5 ). This is important as TRPA1 and TRPV1 are key nociceptive channels in the gastrointestinal tract, as discussed below.

17.10
Animal Models Used to Investigate Sensory Afferent Dysfunction Associated With Prevalent Gastrointestinal Disorders
Short-term inflammation over a period of a few days is a protective process that facilitates wound healing. Such inflammation induces neuronal hypersensitivity of the afferents innervating the gastrointestinal tract. However, it appears that in patients with CVP neuronal hypersensitivity fails to reset back to normal long after healing of the intestinal tissue. In order to investigate potential mechanisms, a myriad of gut infection and inflammatory models have been used. This includes the use of nematodes (e.g., Trichinella spiralis) , bacteria (e.g., Citrobacter rodentium, E. coli ), inflammation with administration of dextran sodium sulfate (DSS), chemical irritants such as mustard oil and acetic acid, and haptens such as trinitrobenzene sulfonic acid (TNBS). However, the time course and nature of the resultant inflammation are different between these models and are defined by the different categories of immune cells involved in the response. For example, DSS is dependent upon innate immunity and is restricted to the mucosa, while TNBS combines with endogenous proteins and antigens to evoke a transmural Th1-mediated inflammation. In contrast, zymosan causes a brief monocyte-based inflammation, but unlike DSS or TNBS, does not induce an increase in myeloperoxidase (MPO) activity at any time after intracolonic treatment. Despite such differences, these models can induce hypersensitivity of extrinsic sensory afferent neurons, their peripheral and central projections and their resultant communication with the CNS.
17.11
Alterations in Extrinsic Sensory Afferent Pathways Innervating the Gut During and After Infection/Inflammation
It is clear that experimentally induced inflammation or infection causes afferent hypersensitivity, neuronal hyperexcitability, and correspondingly hyperalgesia and allodynia in whole animal models. Consistent findings of such changes have been most apparent when studying isolated neuronal cell bodies across different regions of gut and across different experimental models. Most studies utilizing inflammatory (TNBS), nematode ( T. spiralis, Nippostrongylus brasiliensis ) or bacterial models ( C. rodentium ) show that neurons innervating the stomach, small intestine, and the colon display pronounced hyperexcitability after the initial insult. This hyperexcitability is characterized by a decreased threshold for activation, and increased firing rate.
Hypersensitivity of peripheral sensory afferent endings is also evident across a range of different experimental models; however, different afferent subtypes, different neuronal pathways, and time courses are involved in this process. For example, one of the most consistent and long-term displays of visceral hypersensitivity occurs following neonatal insult. In such cases, gastric irritation in neonates results in chronic gastric hypersensitivity and gastric motor dysfunction in adults, in the absence of detectable gastric pathology. Alternatively, neonatal animals receive either mechanical or chemical colonic irritation between postnatal days 8 and 21 and are then tested when they are adults, where they display CVH, allodynia, and hyperalgesia, associated with central neuronal sensitization, in the absence of identifiable peripheral abnormalities.
T. spiralis infection results in both low-threshold and high-threshold jejunal afferents displaying significant reductions in mechanosensitivity at 14 days postinfection. However, at 28 and 56 days postinfection pronounced mechanical hypersensitivity is evident. The development of this longer-term mechanical hypersensitivity is dependent upon innate inflammatory process. By contrast, TNBS-induced colitis causes high-threshold nociceptors to become mechanically sensitized, have reduced activation thresholds, and display hypersensitive responses in inflammatory and post-inflammatory states. This hypersensitivity is particularly apparent in splanchnic afferents with high mechanical activation thresholds and is greater following recovery from overt tissue damage (28 days post-TNBS) ( Fig. 17.6 ). This hypersensitivity translates to an increased density and sprouting of colonic afferent central terminals in the thoracolumbar spinal cord and an increased number of activated dorsal horn neurons in the spinal cord in response to noxious colorectal distension ( Fig. 17.7 ). In contrast, TNBS-induced mechanical hypersensitivity is not evident during inflammation in afferents with low-thresholds (mucosal, muscular, and muscular/mucosal). However, pelvic high-threshold and mucosal afferents only become hypersensitive postinflammation.


Other studies have shown transient, absent, or inconsistent effects of TNBS-induced inflammation on low-threshold distension-sensitive afferents and transient hypersensitivity during in vivo colorectal distension studies. The apparent discrepancy of these findings with TNBS may relate to the severity of mucosal inflammation, which is a predictor for alterations of visceral sensory function in rodents and in humans. Acute zymosan treatment, which recruits a different immune response to TNBS, does lead to low-threshold sensitive afferents displaying short and long-term hypersensitivity. Inflammatory mediators, TNBS, and zymosan treatment can also activate or sensitize two different types of mechanically insensitive silent afferents, the proportion of MIA is increased in a number of inflammatory and postinflammatory states. Another model utilizing intracolonic administration of deoxycholic acid, an unconjugated secondary bile acid, induces a mild, transient colonic inflammation within 3 days, which resolves within 3 weeks. This causes exaggerated visceromotor responses (VMRs) to colorectal distension, referred pain to mechanical stimulation, and increased dorsal horn neuron activity, which persists for at least 4 weeks. Various stress models have been shown to increase visceral pain sensitivity. However, stress, combined with prior acute colitis induced by C. rodentium , results in exaggerated peripheral nociceptive signaling of colonic afferents, their cell bodies and correspondingly VMR thresholds. However, such an interaction does not occur with stress and DSS treatment, which again may suggest specific neuroimmune interactions in the development of neuroplasticity and chronic colonic hyperalgesia. Given this complexity and that understanding of many of these interactions remain in their infancy and are expertly reviewed elsewhere ; the remainder of this chapter will focus on the resultant effects of gut inflammation on extrinsic sensory afferents innervating the gastrointestinal tract and highlight the key ion channels, receptors, and mediators involved mediating nociceptive and antinociceptive sensory signaling.
17.12
Nociceptive and Algesic Mechanisms Contributing to Acute and Chronic Visceral Hypersensitivty
17.12.1
Protease-Activated Receptors
Protease-activated receptors (PARs) are a family of four (PAR 1–4 ) G-protein coupled (GPCR) receptors that typically are activated by proteolytic cleavage of their N-terminal extracellular domain. This releases a new motif on the N-terminal tail that binds to the receptor in an agonist-like fashion. However, many proteases, including trypsins and catepsins, can cleave PARs at divergent sites resulting in biased agonism that can lead to unique pathophysiological outcomes. Colon-innervating DRG neurons express PAR 2 , which when activated affects visceral sensory signaling. Elevated luminal serine protease activity, as well as increased tryptase release, occurs in colonic samples from IBS patients. When administered into the colon of healthy mice, these proteases induced visceral hyperalgesia (increased pain) and allodynia (pain at innocuous stimulus intensities) via a PAR 2 -dependent mechanism. This hypersensitivity can be prevented by a PAR 2 antagonist, was absent in PAR 2 knock-out ( −/− ) mice, and can be replicated by intracolonic PAR 2 agonist administration. Such PAR 2 -mediated effects are further corroborated by recent findings demonstrating that supernatants derived from IBS-D, and IBS-C biopsies increased the excitability of mouse colonic sensory DRG neurons from PAR 2 wild-type ( +/+ ) mice, but not from PAR 2 −/− mice. More recently, in IBS patients, it has been shown that the intestinal epithelium produces and releases the active protease trypsin-3, which is able to signal to human submucosal enteric neurons and mouse sensory neurons, and to induce visceral hypersensitivity in vivo, all by a PAR 2 -dependent mechanism. In animal models of IBD, there is evidence for increased luminal levels of PAR 2 -agonists, particularly cathepsin-S, resulting in increased PAR 2 -mediated activation of spinal nociceptors and visceral hypersensitivity.
17.12.2
Histamine Receptors
Histamine, the predominant mediator released from mast cells, acts via four related G-protein coupled receptors (H 1 , H 2 , H 3 , and H 4 ). Histamine is a powerful modulator of sensory afferent activity at the level of the gut wall and contributes to visceral hypersensitivity. In vivo, selective histamine H 1 receptor (H 1 R) antagonists reduce visceral hypersensitivity in animal models of IBS and IBD. Correspondingly, IBS supernatant-induced excitation of rat jejunal afferents and DRG neurons is reduced by a H 1 R antagonist. This indicates that the pro-nociceptive effect of histamine is, at least in part, mediated by H 1 R expressed on sensory afferents. H 1 R has been demonstrated to couple with both TRPV1 and TRPV4 on gut afferents, which enhances afferent sensory signaling to the spinal cord. Activation of H 1 R is clinically relevant in IBS, as the selective H 1 R antagonist, ebastine, reduced abdominal pain, and improved quality of life in IBS patients in a randomized, double-blind, placebo-controlled, and single-center clinical trial. In addition to H 1 R, there is evidence for histamine H 4 receptors (H 4 R) modulation of visceral sensation. The H 4 R agonist 4-methylhistamine excites human submucous plexus neurons, an effect that is inhibited by the selective H 4 R antagonist JNJ7777120. In a postinflammatory rat model of IBS, this H 4 R antagonist dose-dependently reduced visceral hypersensitivity. Interestingly, there appears to be a functional interaction between H 1 R and H 4 R receptor subtypes, as their simultaneous administration in vivo potentiates their antinociceptive actions.
17.12.3
Serotonin
The majority of 5-HT in the body is found in the gastrointestinal tract, primarily contained within enterochromaffin cells and is released by meals, toxins, and chemotherapeutic agents. In the upper gut, 5-HT release activates vagal mucosal afferent endings, which underlies chemotherapy-induced nausea and vomiting. More recently, it has been demonstrated that microbial metabolites, as well as norepinephrine, result in the release of 5-HT from enterochromaffin cells in the intestine and colon that activates afferents innervating the mucosa via a 5-HT 3 receptor mechanism ( Fig. 17.8 ). These findings demonstrate that enterochromaffin cells are gut chemosensors that couple to sensory neural pathways.

Mucosal barrier function is crucial for the overall function of the gastrointestinal tract; and is disturbed during inflammation associated with IBD, while increased epithelial permeability is evident in the small intestine and colon of IBS patients. These changes allow bacteria to access the interstitial compartment of the gut, resulting in luminally applied LPS from Salmonella typhimurium activating jejunal afferents. These afferents also display an increased sensitivity to 5-HT 3 receptor agonists.
In the rat, 56% of high-threshold splanchnic colonic afferents respond to 5-HT, via both 5-HT 3 and non-5-HT 3 receptors, which correlates with the percentage of thoracolumbar DRG cell bodies retrogradely labeled from the colon that display 5-HT 3 receptors. In the rat, 5-HT 1 , 5-HT 2 , and 5-HT 3 receptor subtypes have been demonstrated to modulate responses to noxious colorectal distension, and serotonergic activation of visceral sensory neurons may increase their sensitivity to other sensory modalities. Following intestinal inflammation, the contribution of 5-HT 3 receptors is decreased, yet the responsiveness to 5-HT is increased, indicating that other 5-HT receptor subtypes play an increased role in disease states. Correspondingly, later studies demonstrated that peripherally administered 5-HT 4 receptor agonists have antinociceptive actions, while also having prokinetic actions and reducing colonic inflammation. In terms of translation to humans, metabolism of 5-HT appears disrupted in both IBS and IBD patients. The involvement of 5-HT in IBS patient symptomatology is implicated by increased number of enterochromaffin cells, increased mast cell populations, increased postprandial 5-HT release, and a decrease in symptoms using 5-HT 3 receptor or 5-HT 4 receptor antagonists.
17.12.4
Voltage-Gated Sodium (Na V ) Channels
Na V channels are large complex structures composed of a primary α subunit as the pore-forming region along with one or more auxiliary β subunits. Na V channels are essential for action potential generation, propagation and the overall control of membrane excitability. Nine different mammalian Na V channels, Na V 1.1-Na V 1.9, have been identified and can be divided into two groups based on their sensitivity to tetrodotoxin (sensitive: Na V 1.1, Na V 1.2, Na V 1.3, Na V 1.4, Na V 1.6, Na V 1.7; resistant: Na V 1.5, Na V 1.8, and Na V 1.9). From a translational perspective, intrarectal administration of lidocaine (a pan-Na V channel blocker) in IBS patients reduces rectal sensitivity and abdominal pain. These findings suggest that Na V channels and the activation of peripheral afferent endings in the colon play key roles in the CVP experienced by IBS patients. It also shows that treatments applied in the periphery can reduce abdominal pain in IBS patients, independent of CNS actions.
Recent studies show diverse roles for specific Na V channels in sensation from the gastrointestinal tract. Functional studies of colon innervating afferents reveal that Na V 1.1 plays a crucial role in signaling mechanical pain from the colon. Application of the highly selective Na V 1.1-agonist, δ-theraphotoxin-Hm1a (Hm1a), enhances mechanically evoked firing in a subpopulation of high-threshold colonic nociceptors, which is blocked by incubation with the Na V 1.1/Na V 1.3 antagonist ICA-121431 ( Fig. 17.9 ). Furthermore, Hm1a also induces hyperexcitability of isolated colon-innervating DRG neurons from healthy control mice. Importantly, colon-innervating DRG neurons isolated from mice with CVH show significantly enhanced responsiveness to Hm1a compared to healthy control mice, suggesting that Na V 1.1 is essential for the development and maintenance of chronic visceral pain conditions ( Fig. 17.9 ). Accordingly, antagonism of Na V 1.1 may be a future target for the treatment of disorders accompanied by chronic visceral pain originating from the colon.

Na V 1.5 in the circular smooth muscle contributes to normal intestinal motility through the modulation of slow-wave activity and muscle contractility. Ranolazine, a treatment for chronic angina, is able to inhibit Na V 1.5 currents in human colonic smooth muscle cells, which is likely to be responsible for the constipation seen during long-term ranolazine treatment. Several loss-of-function mutations in SCN5A , the gene encoding Na V 1.5 channels, have been reported in IBS patients, which may be associated with their altered motility and abdominal pain.
Biopsies from patients with rectal hypersensitivity show increased expression of Na V 1.7 channels in rectal sensory fibers. However, deletion or pharmacological blockade of Na V 1.7 in mice does not affect colonic afferents in healthy states. There is evidence for increased Na V 1.8 expression in the DRG of mice in models of colitis, while Na V 1.8 plays a key role in ciguatoxin (a pan-Na V channel activator)-induced activation of colonic nociceptors and visceral pain. Na V 1.9 is required for responses to intense mechanical stimulation, contributes to inflammatory mechanical hypersensitivity, and is essential for activation by noxious inflammatory mediators, such as ATP, PGE2, bradykinin, ATP, histamine, and 5-HT.
17.12.5
Acid Sensing Ion Channels
Acid sensing ion channels (ASICs) are members of the DEG/ENaC family of ion channels, which contribute to the detection of pH and mechanical stimuli via entry mainly of sodium ions through four related channels, ASIC1, ASIC2, ASIC3, and ASIC4. These ASICs form heteromultimers, and the composition of these heteromultimers differs depending on the tissue studied. In terms of the gut, ASIC1, 2, and 3 mRNA expression has been detected in vagal and spinal pathways but their abundance varies greatly between gut innervating afferents. ASIC1 has a greater expression in gastroesophageal innervating nodose ganglion neurons compared with colon innervating neurons, similar levels of ASIC2 are in both sets of neurons, while colonic neurons contain more ASIC3 than gastroesophageal neurons. As little as 30% of colonic neurons express ASIC1, while 47% express ASIC2 and 73% express ASIC3. Correspondingly, studies utilizing ASIC −/− mice have demonstrated widespread differences in the mechanosensory mechanisms between different pathways. Deletion of ASIC1a increases the mechanical sensitivity of splanchnic colonic and vagal gastroesophageal afferents ( Fig. 17.10 ).

Disruption of ASIC2 has varied effects: increasing mechanosensitivity in gastroesophageal mucosal endings, decreasing gastroesophageal tension receptors, increasing colonic serosal endings, and causing no change in colonic mesenteric endings ( Fig. 17.10 ). In ASIC3 −/− mice, gastroesophageal tension afferents have markedly reduced mechanosensitivity, while mucosal afferents are unchanged. In the colon, splanchnic serosal and mesenteric afferents display mechanosensory deficits, and in the pelvic nerve muscular/mucosal afferents have significantly reduced mechanosensory responses ( Fig. 17.10 ). Low-threshold pelvic mucosal and muscular afferents remained unchanged. Although the deficits in mechanosensitivity observed in the respective ASIC −/− mice are fairly modest, particularly compared with those documented for TRPV4 and TRPA1 ( Fig. 17.10 ), the effects on afferent mechanosensitivity translate to changes in the whole animal. Observations of altered gastric emptying and fecal output are found in ASIC1a −/− and ASIC2 −/− mice, respectively, while reductions in colonic mechanosensory function in ASIC3 −/− mice translate to significantly reduced VMR to colorectal balloon distension (CRD). In humans, increased ASIC3 expression is observed in Crohn’s disease intestine. Differences between the upper gut and lower gut afferents are also apparent in the sensitivity to the nonselective DEG/ENaC blocker benzamil. Splanchnic colonic afferent mechanosensitivity is virtually abolished by benzamil application, whereas gastroesophageal afferents are only marginally inhibited. Deletion of ASIC2 or ASIC3 significantly reduces the ability of benzamil to reduce splanchnic serosal afferent mechanosensitivity, while deletion of ASIC1a has no effect on benzamil sensitivity. ASIC3 also contributes to visceral peripheral sensitization as demonstrated by an intracolonic zymosan induced increase in VMR in control mice, which is lost in ASIC3 −/− mice. Deletion of ASIC3 also prevents gastritis-induced acid hyper-responsiveness of the stomach-brainstem axis. In terms of potential therapeutic options, although potent ASIC3 blockers are available, they need to be extremely selective to avoid serious side effects given that ASIC1a and ASIC2 are involved in CNS and baroreceptor function.
17.12.6
TRPV1
TRP channels constitute a major superfamily of nonselective ion channels that can be characterized as ankyrin repeat (TRPA), canonical (TRPC), melastatin related (TRPM), mucolipin (TRPML), polycystin (TRPP), and vanilloid binding (TRPV). TRPV1 is probably the best-known member of the TRP channel family as it is the archetypal somatic pain sensor and is expressed on small diameter primary afferent neurons (C-fibers) in DRG. Transient receptor potential channel vanilloid 1 (TRPV1) acts as an immediate sensory alarm in response to low pH, heat, and noxious agents such as capsaicin. Therefore, TRPV1 has been strongly implicated in somatosensory nociception and pain, including thermal nociception and inflammatory hyperalgesia and allodynia, and neuropathic pain. Because of this link to pain, TRPV1 is one of the most heavily studied channels in the gastrointestinal tract. However, compared with cutaneous afferents, the role of TRPV1 in the viscera is not as straightforward. This is because its expression is not necessarily indicative of sensory neuron nociceptor function. First, in mouse, TRPV1 expression is greater in colon innervating neurons than those innervating the skin, with 82% of thoracolumbar and 50%–60% of lumbosacral colonic DRG neurons displaying TRPV1-LI. Correspondingly, all classes of low-threshold and high-threshold afferents in vagal and spinal pathways respond in varying degrees to capsaicin, with a high-functional expression of TRPV1 in all colonic afferent subtypes. Capsaicin also causes mechanical desensitization in vitro in gastroesophageal, jejunal, and splanchnic colonic preparations. Finally, TRPV1 is also expressed in nonneuronal gut tissues, such as the rat esophageal epithelium where there is a TRPV1 splice variant, which is significantly different (60 vs 95 kDa) to the sensory DRG form of TRPV1.
Corresponding with these differences in expression, stark contrasts also exist between somatic and visceral afferents when investigating mechanosensory function in TRPV1 −/− mice. Deletion of TRPV1 has no effect on somatic mechanosensory function ; however, deficits are apparent in intestinal afferents, specifically in certain types of low-threshold distension sensitive gastroesophageal, jejunal, and pelvic colonic afferents. By contrast, high-threshold gut afferents are unaffected by TRPV1 deletion. Nevertheless, TRPV1 has been strongly implicated in contributing to visceral pain. The ability of TRPV1 to respond to low pH places it in a prime position to sense esophageal acid reflux, giving rise to the sensation of heartburn, while intraesophageal capsaicin instillation induces symptoms of epigastric burning. In animal models, intracolonic capsaicin administration causes pronounced visceral pain, while TRPV1 −/− mice also display decreased VMRs to CRD, suggesting an involvement of TRPV1 in signaling behavioral aspects of colonic pain. A key interaction in this process appears to be via TRPV1 and the G protein-coupled receptor kinase 6 (GRK6). The proinflammatory cytokine IL-1β sensitizes TRPV1, which can be prevented by overexpressing GRK6. Following colitis, TRPV1-induced behavioral pain responses are more pronounced in GRK6 −/− mice than in GRK6 +/+ mice, suggesting that GRK6 can regulate inflammation-induced sensitization hyperalgesia.
An often-overlooked function of TRPV1 activation is its ability to cause neurogenic inflammation via the local release of substance P and CGRP from sensory afferent endings. Mice lacking TRPV1 develop significantly less esophagitis after acid exposure compared with TRPV1 +/+ mice, while administration of a TRPV1 antagonist before or after intracolonic TNBS administration significantly reduces colitis severity. Similarly, in rats and mice, TRPV1 antagonists attenuate disease severity in DSS-induced colitis. However, diametrically opposed to these observations there are also reports of TRPV1 having a protective role in inflammatory models, with TRPV1 activation improving DSS-induced colitis in mice. Furthermore, a dinitrobenzene sulfonic acid model causes more severe colitis in TRPV1 −/− mice than TRPV1 +/+ mice. In terms of translation to humans, expression of TRPV1 is increased in colonic nerve fibers of IBD patients, and in patients with rectal hypersensitivity.
As discussed above, numerous inflammatory mediators can interact with TRPV1 to modulate their functional properties. In colon innervating DRG neurons, 5-HT can sensitize TRPV1 function. Specifically, 5-HT incubation increases capsaicin-induced currents and decreases the activation threshold temperature of TRPV1 from 42°C to 38°C. These effects can be mimicked by 5-HT 2 and 5-HT 4 receptor agonists, while this sensitization of TRPV1 can be reduced by selective 5-HT 2 and 5-HT 4 receptor antagonists, but not by a 5-HT 3 receptor antagonist.
17.12.7
TRPV4
TRP channel vanilloid 4 (TRPV4) is the mammalian homolog of the Caenorhabditis elegans gene Osm-9, which is critical in osmotic and mechanical avoidance, suggesting that it functions to detect noxious osmotic and mechanical stimuli. In gut innervating sensory DRG neurons, TRPV4 expression is more restricted than that observed for TRPV1. TRPV4 is localized in 38% of gastroesophageal vagal neurons, 65%–76% of splanchnic colonic DRG neurons, and 58% of pelvic colonic DRG neurons. Although TRPV4 expression is more restricted, it is actually enriched within colonic DRG neurons, with its expression 20-fold greater in splanchnic colonic DRG neurons than in whole DRG. In the periphery, TRPV4 protein colocalizes with CGRP in colonic nerve fibers in the serosal and mesenteric attachment. By contrast, TRPV4 expression is scarce in intramuscular or mucosal nerve fibers.
Consistent with the lack of TRPV4 expression in gastroesophageal vagal neurons deletion of TRPV4 has absolutely no effect on vagal afferent function. However, in colonic afferents, where TRPV4 is enriched, mechanosensory responses are dramatically reduced in TRPV4 −/− mice. This is the case for both splanchnic serosal and mesenteric afferents. TRPV4 deletion also increases the mechanosensory thresholds of these afferents, suggesting that TRPV4, like TRPA1 (discussed below), contributes to setting mechanical activation thresholds. Furthermore, pelvic serosal afferents display similar deficits in mechanosensory responses and thresholds to those seen in the splanchnic pathway ( Fig. 17.10 ). Consistent with the case in the vagal pathway, low-threshold pelvic afferents are completely unaffected by TRPV4 deletion. Taken together, these data indicate that TRPV4 makes a specific and major contribution to high-threshold colonic afferent mechanosensory function. The extent of these deficits in mechanosensitivity are some of the largest reported in any afferent population, somatic or visceral. These changes in colonic neuron function also translate to decreased VMR to CRD in TRPV4 −/− mice or in mice with downregulated TRPV4 expression (via siRNA intrathecal injection), the extent of which is particularly apparent at higher distension pressures.
The endogenous TRPV4 agonist 5,6-EET (an arachidonic acid metabolite) increased the mechanosensory responses of high-threshold colonic afferents in TRPV4 +/+ mice, but not in TRPV4 −/− mice. Another TRPV4 agonist, 4α-PDD caused significant TRPV4-mediated calcium influx in isolated colonic DRG neurons. Intracolonic administration of 4α-PDD in TRPV4 +/+ mice increased neuronal c-fos expression in the lumbosacral spinal cord and caused dose-dependent visceral hypersensitivity. Therefore, TRPV4’s role is not only linked to visceral mechanosensation but also to visceral hypersensitivity.
TRPV4 can be sensitized by a series of inflammatory mediators both in vivo and in vitro. Preexposure of colonic DRG neurons to histamine or 5-HT increased Ca 2 + responses induced by 4αPDD via various second messenger pathways. 5-HT or histamine also enhances TRPV4 expression at the plasma membrane. Downregulation of TRPV4 via siRNA intrathecal injection significantly reduces the hypersensitivity induced by 5-HT or histamine. Although PAR 2 interacts with TRPV1 and TRPA1 (see above), there is evidence for a strong interaction with TRPV4, which underlies visceral hypersensitivity. TRPV4 and PAR 2 are anatomically and functionally coexpressed in a large percentage of colon innervating neurons. In isolated colonic DRG neurons, the PAR 2 -activating peptide, PAR 2 -AP, sensitizes TRPV4-mediated currents, while activating splanchnic serosal colonic afferent fibers by a TRPV4-dependent mechanism. Intracolonic administration of PAR 2 -AP also causes enhanced VMR to CRD at higher distending pressures (> 40 mmHg) measured 6 h and 24 h after administration. This is not observed in TRPV4 −/− mice at either time point, suggesting that TRPV4 mediates both the acute and delayed hyperalgesia induced by PAR 2 activation. Taken together, these data suggest that TRPV4 is a common mechanism to several known mediators of visceral hypersensitivity, including histamine, 5-HT, and PAR 2 .
At face value, TRPV4 appears to be an ideal target for the treatment of visceral pain. TRPV4 has a high specificity for high-threshold spinal colonic afferents with one of the largest deficits in mechanosensory function documented in TRPV4 −/− mice. There appears to be little to no role in low-threshold spinal afferents or in vagal afferent fibers. TRPV4 is concentrated in the outer layers of the colon and is association with blood vessels, in particular during active inflammation of the colon associated with Crohn’s disease and ulcerative colitis. Serine protease levels, 5-HT signaling, and histamine are all known to be increased in IBS patients and TRPV4 interacts with these mediators. Finally, IBS patient biopsy samples have been shown to contain increased levels of specific polyunsaturated fatty acid metabolites, which stimulate extrinsic sensory neurons from mice and generate visceral hypersensitivity via a TRPV4-dependent mechanism.
17.12.8
TRPA1
TRP channel ankyrin 1 (TRPA1) has been implicated as a mechanosensor and a major mediator of inflammatory pain. In gut innervating sensory neurons, TRPA1 expression is more restricted than that observed for TRPV1. In these neurons, TRPA1 is localized in both vagal and spinal afferents, with 55% of vagal gastroesophageal afferent neurons, 54% of splanchnic colonic, and 58% of pelvic colon innervating DRG neurons expressing TRPA1. In peripheral tissue, TRPA1 is located in mucosal, serosal, and mesenteric nerve fibers, indicating that TRPA1 is well placed to participate in the function of these afferent subtypes. Correspondingly, TRPA1 agonists (mustard oil or cinnamaldehyde) universally evoke mechanical sensitization of serosal and mesenteric high-threshold colonic afferents and pelvic mucosal afferents. These compounds also activate colon innervating DRG neurons in isolation. The use of TRPA1 −/− mice has allowed specific determination of the afferent fiber types that utilize TRPA1 to detect mechanical stimuli. These studies show striking deficits occurring in high-threshold colonic afferents, specifically splanchnic mesenteric afferents and splanchnic and pelvic serosal afferents ( Fig. 17.10 ). Furthermore, TRPA1 makes a modest contribution to low-threshold mucosal afferent mechanosensitivity in both vagal and pelvic pathways innervating the esophagus/stomach and colon, respectively. In contrast, TRPA1 does not contribute to the mechanosensitivity of vagal tension receptors or pelvic muscular and muscular/mucosal afferents. Interestingly, these are the classes of afferent that are affected by TRPV1 deletion. Another important finding is the observation that TRPA1 deletion increases the activation thresholds of serosal and mesenteric afferents to mechanical stimuli, suggesting that TRPA1 is important in setting mechanical activation thresholds. The predominant mechanosensory role for TRPA1 in high-threshold serosal and mesenteric afferents combined with the ability of TRPA1 agonists to increase the mechanical responsiveness of these afferents is suggestive of an involvement in pain. Supporting this assertion, TRPA1 −/− mice also display decreased VMR to high intensity (80 mmHg) CRD, but not lower distension pressures (15–60 mmHg). The observation that intracolonic administration of TRPA1 agonists enhances VMR to the higher distending pressures (45 and 60 mmHg) within 2 h of administration suggests that TRPA1 can modulate visceral mechanical hyperalgesia (discussed below). Further inference can also be deduced from the observation that the classes of TRPA1 −/− afferent displaying deficits are exactly the same classes of TRPA1 +/+ afferent displaying mechanical hypersensitivity after TNBS-induced colitis, namely, splanchnic mesenteric and serosal afferents and pelvic serosal and mucosal afferents.
Mustard oil has long been used as a visceral inflammatory model to provoke tissue damage and sensitize nociceptors. We now know that mustard oil (allyl-isothiocynate) is a TRPA1 agonist, and recent findings provide us with a more complete understanding of how this compound causes these effects in the viscera. TRPA1 agonists induce neurogenic inflammation via the release of substance P and CGRP, with increases in cytokines associated with macrophage and neutrophil activation and recruitment. However, the contribution of TRPA1 to different types of inflammation may not be as prevalent as that of TRPV1, with recent reports suggesting that the severity of colitis induced by TNBS is unaffected by TRPA1 deletion.
TRPA1 is also directly activated by irritants such as the endogenous aldehyde, 4-hydroxynonenal (4-HNE). Intracolonic administration of mustard oil or 4-HNE increases the VMR to CRD at the highest distending pressures (45 and 60 mmHg, respectively) within 2–3 h. Correspondingly, these agonists also cause neuronal activation, as indicated by induced c-fos expression, in the superficial laminae (I and II) of thoracolumbar (splanchnic) and lumbosacral (pelvic) spinal cord in TRPA1 +/+ but not TRPA1 −/− mice. Thus, intracolonic administration of TRPA1 agonists activates spinal nociceptors and causes visceral hyperalgesia. TRPA1 is also indirectly activated by inflammatory mediators such as bradykinin and PARs. TRPA1 mediates bradykinin-induced mechanical hypersensitivity in the guinea pig esophagus and the bradykinin-induced mechanical hypersensitivity observed in splanchnic serosal afferents. TRPA1 is also required for PAR 2 -induced visceral hyperalgesia as the delayed visceral mechanical hypersensitivity to CRD caused by intracolonic administration of the PAR 2 -activating peptide in TRPA1 +/+ mice is completely absent in TRPA1 −/− mice.
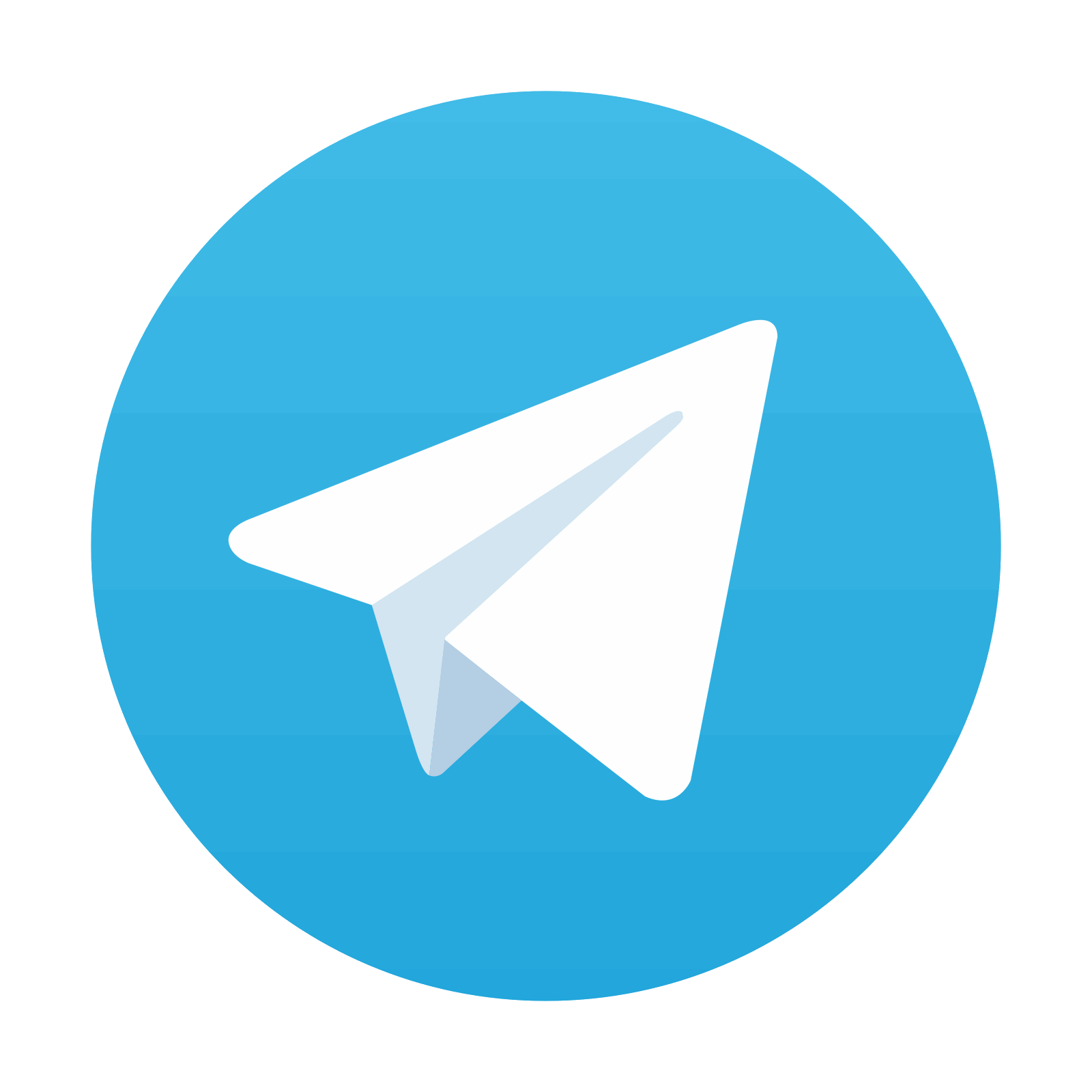
Stay updated, free articles. Join our Telegram channel

Full access? Get Clinical Tree
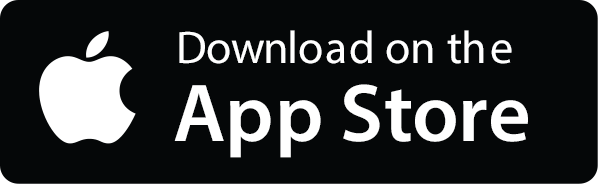
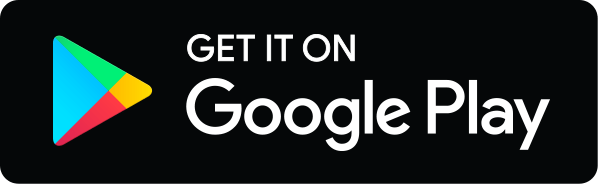