Fig. 2.1
Charles Darwin
Technologic advancement has lent itself to the development of innovative surgical technique and has changed the surgical standard of care in several areas. Laparoscopic cholecystectomy is a technique that has demonstrated clear-cut advantages over its open surgery predecessor. From decreased postoperative pain to shorter length of stay, the superiority of this approach from a short-term outcome standpoint catapulted it to the standard of care for surgical treatment of routine gallbladder disease [1–4]. Although not extinct, open cholecystectomy represents less than 10 % of gallbladder resections performed annually. Similarly, routine tubal ligation is almost exclusively performed in a laparoscopic manner, avoiding the unnecessary morbidity of a laparotomy.
While shown to be oncologically sound and to significantly reduce postoperative pain and length of stay [5–8], laparoscopic colectomy (LC) has experienced a flatter trajectory in its evolution with less than a 50 % implementation for colorectal resections. Attributed to the technical challenges of the approach and significant learning curve [9, 10], the lack of significant adoption of the laparoscopic approach may signify the establishment of an intermediate surgical technique. To clarify, the outcome advantages and technical feasibility may not demonstrate enough robustness to supplant the standard open approach [11]. Some of the technical obstacles of laparoscopic colectomy include inadequate optics with poor resolution of 2D images, incongruous eye–hand coordination, decreased dexterity of laparoscopic instruments, and reduced sensory information with a loss of accurate haptic feedback.
Evolution is a concept that relies upon constant, random mutation. Although not random, but rather the result of conscious innovation, the introduction of robotic technology to the field of surgery can be viewed as one such mutation. From rudimentary instruments such as the PUMA 560, designed to perform more precise biopsying of the brain and prostate, to the more sophisticated platforms of the ZEUS and da Vinci® systems which enable surgeons to perform complex minimally invasive resections, robotic technology has emerged as a variant of MIS aimed at addressing the technical limitations of laparoscopy and expanding the benefits to patients. With its origin in cardiothoracic surgery, robotic surgery has metastasized to such specialties as urology, gynecology, ENT, and colorectal surgery. Within the field of colorectal surgery, surgeons have reported on the expansion of their capabilities and technique in regards to the optics, dexterity of instruments, and stability of the current robotic platform. The goal of this chapter is to address the evolution of robotic technology and robotic surgical technique.
2.1 The Dawn of Laparoscopic Surgery
Interest in evaluating the occult spaces of the human body using tube instruments and rudimentary light sources was first noted in artifacts from ancient Mesopotamian and Greek civilizations. However, the development of modern endoscopic technology truly found its beginnings in the early nineteenth century when several European physicians contrived the first cystoscope which was used to look into the vagina, urethra, and bladder. The term “celiacoscopy” was coined by the German surgeon Georg Kelling (1866–1945) in 1901 after inserting a cystoscope into the abdomen of a laboratory dog followed by cadaveric and live humans [12]. Kelling had experimented with high-pressure insufflation of air into the abdominal cavity (Lufftamponade) as a means to halt intra-abdominal bleeding. To evaluate the effect of the air tamponade on the intra-abdominal contents, he began inserting a cystoscope.
While Kelling never published his results, Hans Christian Jacobaeus (1879–1937), a Swedish internist, did report on the first use of “laparothoracoscopy” in 1910 during his investigation of pneumoperitoneum as a treatment for tubercular peritonitis. He chronicled the use of this novel endoscopic technique in 97 patients, using the large volume of ascites created in the condition as a means of inflating the abdomen and protecting from intestinal injury.
Slow progress ensued over the next 50 years in the field of laparoscopy, but culminated in a publication by American surgeon, Raoul Palmer, in the early 1950s describing the first diagnostic laparoscopy. German gynecologist, Kurt Semm, led the charge for pelviscopic and laparoscopic surgery in the mid-1950s, developing techniques such as minimally invasive adnexectomy, hysterectomy, and appendectomy. As an instrument maker, he invented many sophisticated laparoscopic instruments such as a CO2-pneumatic insufflator, a thermocoagulator, and several intracorporeal knot-tying devices. Unfortunately, Semm was harshly ridiculed by his gynecologic colleagues and many international societies, with opponents demanding that he undergo a “brain scan” for “only a person with brain damage would perform laparoscopic surgery” [13]. With passion and perseverance, Semm continued his work and began to influence open-minded surgeons in both Europe and the USA.
The late twentieth century ushered in an era of laparoscopic implementation and diversification. The first descriptions of laparoscopic cholecystectomy were reported by Erich Mühe (Germany, 1985), Philippe Mouret (France, 1987), Francois Dubois (France, 1988), and McKernan/Saye (USA, 1988). After presenting a paper describing the first laparoscopic cholecystectomy at the German Surgical Society (GSS) meeting in 1986, his paper was rejected, much like the work of Semm some 30 years before. Mühe was later given the highest honor by the GSS in 1992, recognizing the substantial contribution he had made toward the development of laparoscopic surgery. The use of trocars, specialized instruments such as graspers and scissors, and vessel clips was introduced in these early procedures. Interestingly, Mühe had developed his own “galloscope” through which he performed the first laparoscopic cholecystectomies (Fig. 2.2). The galloscope consisted of side-viewing optics, a light source, a duct for insufflation, and instrumentation channels with valves. He adopted early on a single-incision approach, described as an “open tube laparoscopic cholecystectomy,” which necessitated a single 2.5 cm incision created directly above the gallbladder for his galloscope and required no insufflation [14, 15]. This technique was not adopted universally, as the multi-port approach with insufflation demonstrated the advantages of enhanced visualization and range of motion for instruments.
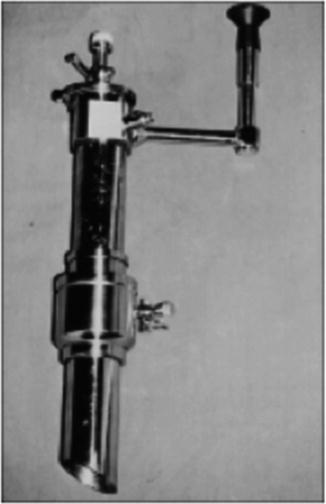
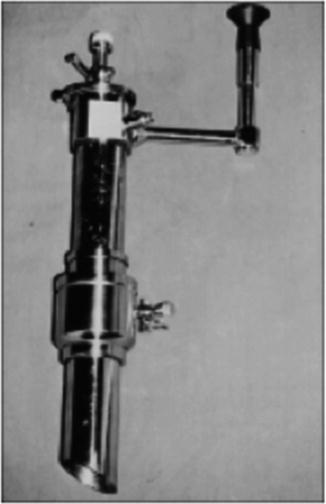
Fig. 2.2
The Mühe “galloscope” through which was used to perform the first laparoscopic cholecystectomy
As laparoscopic cholecystectomy gained momentum, surgeons began to expand the use of laparoscopic technology to include such procedures as appendectomy, adrenalectomy, gastrectomy, and colectomy. Large series and randomized controlled trials emerged in the literature demonstrating the feasibility, safety, and oncologic soundness of laparoscopic colectomy for both benign and malignant colorectal disease [16–18].
2.2 Robotic Technology
While early surgical robotic technology was originally created to enhance precision in biopsying, the development of more complex robotic surgery platforms was first driven by the National Aeronautics and Space Administration (NASA) and the US military in hopes of achieving telepresence surgery. Telepresence surgery refers to the concept of performing surgery from a remote location outside of the operating room, such as the battlefield or space station. When physicians became exposed to this new research, they recognized a very imminent application of robotic technology in the current operating room. The collaboration between surgeons and roboticists culminated in a variety of telepresence devices such as AESOP, PROBOT, and ROBODOC which provided very specific, but limited surgical activities (i.e., voice-activated, “third-arm” camera assistance, more precise prostate biopsying, and femur manipulation in hip replacements) [19]. AESOP’s voice-activated technology was incorporated into the ZEUS Robotic Surgical System (ZRSS) by Computer Motion, an early master–slave platform that consisted of three robotic arms controlled by the surgeon sitting at a remote console. ZRSS was cleared by the FDA in 2001, but was discontinued when a merger between Computer Motion and Intuitive Surgical occurred in 2003.
In 2000, Intuitive Surgical launched their first FDA-approved robotic surgery platform, the da Vinci® Surgical System, and has been the most widely used surgical platform to date. The da Vinci® Surgical System is comprised of a patient-side cart with four interactive arms controlled by the surgeon at the console using his forefingers and thumbs to manipulate the master controls.
2.3 Optics
From the primitive light-sensitive “eyespot” of simple unicellular organisms to the more complex optical systems of vertebrates, the evolution of modern optics has relied upon 540 million years of gradual mutation. Luckily, surgical optics have developed over a much shorter period of time. Building upon the early developments of such visionaries as Austrian Philipp Bozzini who created the Lichtleiter (Fig. 2.3) in 1805, the first illuminated scope which consisted of a rudimentary viewing tube, a series of mirrors, and a candle as a light source, and German dentist Julius Bruck who first linked a medical scope to an electric light source (1867), physicists have been pushing the boundaries of medical optics with the hope of enhancing surgical capabilities. The development of fiber-optic technology in the 1950s allowed for improved illumination but exposed the deficits of lens design. A series of small lenses were required to propagate the light through the shaft of the early scopes, but high-quality lenses were exceptionally difficult to manufacture. British physicist Harold Hopkins unveiled his “rod lens” design, which eliminated the need for lenses by using glass rods to transduce the light and allowed for the development of smaller scope diameter.
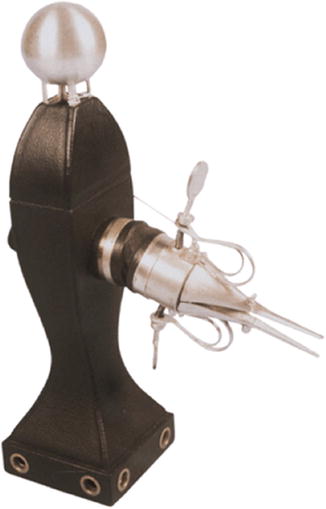
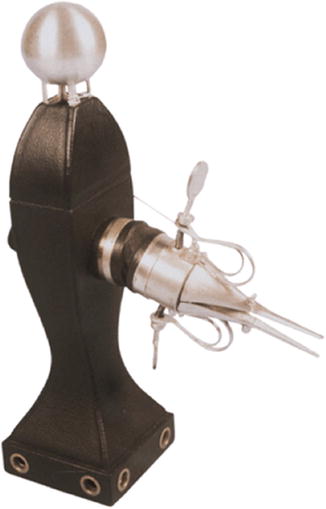
Fig. 2.3
The Lichtleiter created by Austrian Philipp Bozzini in 1805, the first illuminated scope which consisted of a rudimentary viewing tube, a series of mirrors, and a candle as a light source
Until analog medical cameras were made available in the 1970s to attach to the scopes, the surgeon was required to utilize the eyepiece for visualization of the surgical field. The development of medical cameras utilizing silicon chip technology or charge-coupled devices (CCD) in 1982 was considered a groundbreaking technologic advancement in that it allowed for the storage of a digital image of the surgical scene that could be transmitted more efficiently and with greater resolution than its analog predecessor. These higher-quality images could be projected onto monitors that could be viewed by the entire surgical team. Standard laparoscopic images are 2-D and result in the loss of binocular cues, limited depth perception, and increased motion parallax [20].
Robotic surgery optical technology has revolutionized MIS optics in that it has allowed for 3-D visualization of the surgical field at the remote console. 3-D technology is centered around the concept of stereopsis or creation of depth perception. The robotic system creates depth of field using two cameras and two different lenses, projecting images that are slightly different in each eye. A 3-D image is the result of the ocular disparity registered by the brain. Enhanced depth perception has been associated with a shorter learning curve in particular MIS skills such as intracorporeal knot tying and suturing [21, 22].
2.4 The Surgical Hand
The acquisition of human stereoscopic vision accompanied the evolution of the prehensile hand. With the densest concentration of nerve endings of the entire body centered at its fingertips, the hand is the organ that is most intimately associated with the sense of touch. Haptic feedback generated by the hand is responsible for spatial recognition and interpretation of density and texture of objects, cognitive skills essential to surgical performance. As MIS became a prominent surgical technique, a reduction of sensory information resulted as direct contact of the hand with the surgical field was replaced with crude feedback from rigid laparoscopic instruments. Decreased dexterity and range of motion are often cited as drawbacks of laparoscopy and contribute to the steep learning curve of complex MIS procedures. The robotic “hands” of the current system attempt to regain some of these lost functions by providing wristed instruments with 90 degrees of articulation and 7 degrees of freedom. While little to no haptic feedback exists with this platform, the concept of surgical synesthesia has been suggested, referring to the development of a sense of “touch” from the visual cues gained as an object is touched [20, 23, 24].
In addition to the specialized hands, the stability of the robotic platform allows for more precise fine motor activities, such as dissection, tissue manipulation, and suturing. The significant tremor reduction of the robotic platform is considered an advantage over both laparoscopic and open surgery.
2.5 Ergonomics of the Robotic Surgeon
Chronic musculoskeletal and vertebral disk injuries have been associated with the prolonged ergonomic stress of traditional laparoscopy [25–27]. Many of the chronic back, neck, and shoulder injuries described are attributed to the incongruous technical maneuvers required with straight-stick laparoscopic instruments, awkward body positioning, and non-ergonomic positioning of the operating table and video console [28]. Surgeon laparoscopic case volume and workload over time have been specifically linked to chronic musculoskeletal pain and discomfort [29]. It has been suggested that less surgeon fatigue occurs during robotic surgery as the operator sits in a more comfortable position at the console, manipulating lightweight master controls.
In a quantitative comparison study evaluating muscle activation during traditional laparoscopic surgery (TLS) versus robotic-assisted surgery (RAS), surface electromyography measurements were recorded from the surgeon’s biceps, triceps, deltoid, and trapezius muscle groups [30]. Significantly elevated muscle activation was noted in the biceps, triceps, and deltoid muscles during TLS, compared to RAS. Similar levels of muscle activation were noted in the trapezius muscles in both TLS and RAS. These findings suggest that the more ergonomic design of the robotic surgery platform may minimize surgeon muscular fatigue over time and perhaps improve overall productivity and longevity of practice.
2.6 Evolution of Robotic Surgical Technique
Robotic surgical technique for numerous procedures has developed after many painstaking hours in the laboratory and operating room. From the number of trocars utilized to the positioning of instruments, current surgical instrument configuration has largely been established through a trial-and-error process. Some of the first robotic surgical experiences describe techniques used for cardiac and gynecologic procedures. In 2000, Kappert et al. [31] reported on their “three-point stab incision” trocar arrangement for closed-chest coronary artery bypass while Lapietra et al. [32] described a three-port approach for the thoracoscope and two needle holders, with the use of a fourth “service entrance” incision in the seventh interspace for suture passing, retractor blade placement, and introduction of the valve prosthesis for mitral valve surgery. In the first reported series of hysterectomy with bilateral salpingo-oophorectomy, a four- to five-port approach was detailed by Diaz-Arrastia et al., utilizing a camera port, two robotic arm ports, and 1–2 laparoscopic assistant ports [33].
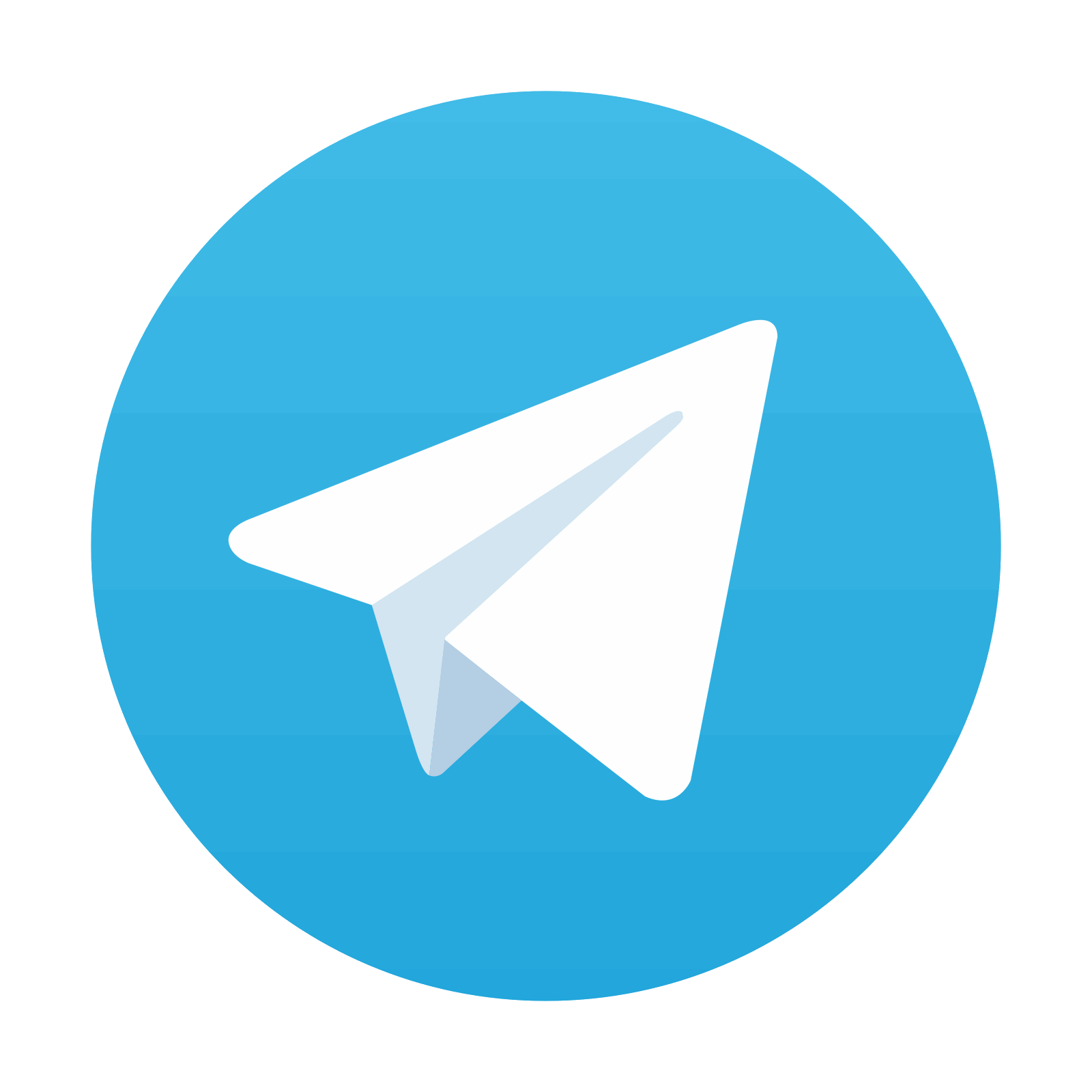
Stay updated, free articles. Join our Telegram channel

Full access? Get Clinical Tree
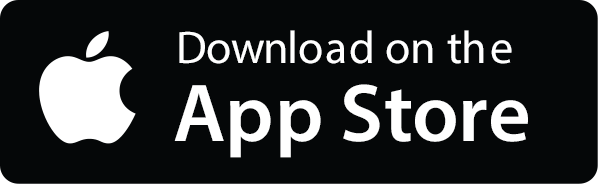
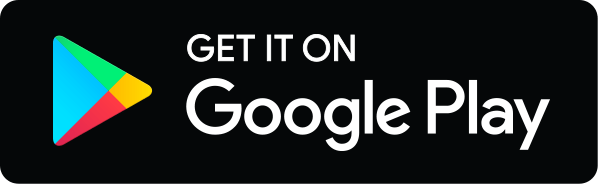