Manometry and impedance provide only surrogate information regarding longitudinal wall function and are focused on contractile amplitude and lumen content. Ultrasound imaging provides a unique perspective of esophageal function by providing important information regarding longitudinal muscle contraction. Laser Doppler assessment of perfusion may be an important complementary tool to assess abnormal wall blood perfusion as a possible mechanism of pain.
Key points
- •
Manometry and impedance provide information regarding circular muscle function and luminal content respectively.
- •
Ultrasound imaging provides a unique perspective of esophageal function by providing important information regarding longitudinal muscle contraction, coordination between the two muscle layers of muscularis propria and esophageal distension during bolus transport.
- •
Laser Doppler assessment of perfusion may be an important complementary tool to assess blood perfusion of esophageal wall as a possible mechanism of pain.
Introduction
Endoscopy, barium swallow, high-resolution manometry (HRM), pH measurement (either catheter based or using Bravo system), and impedance monitoring of the esophagus are major esophageal function testing techniques that are available in most academic centers and centers of excellence for esophageal function testing. These recording techniques provide key information on the sensory and motor functions of the esophagus that allows one to treat patients based on the physiologic understanding of the patient’s symptoms. Another esophageal function testing technique, which even though has not gained wide popularity and acceptance, is ultrasound (US) imaging of the esophagus using either catheter-based intravascular US imaging probe or US endoscope. The latter is suited for anatomic/morphologic information of the esophageal muscle and identification of lesions that affect esophageal function by compression of the esophagus. On the other hand, catheter-based US imaging provides information on anatomy/morphology as well as functional aspects of esophageal muscle function. In order for US imaging, especially dynamic US imaging, to gain popularity it will have to be user friendly and less expensive. To date, dynamic US imaging has only been used in research laboratories. There is no question though that the static and dynamic US imaging, using either US endoscopes or catheter-based US probes, provides key information of esophageal functions that is unparallel to any other technique.
Laser Doppler blood perfusion measurement of the esophageal wall is another important modality, especially if future testing confirms that “noncardiac” chest pain and proton pump inhibition (PPI)-resistant heartburn are related to low blood perfusion or relative ischemia of the esophageal wall. The goal of this writing is to provide principles underlying these techniques and to update the status of information that these techniques have yielded.
Introduction
Endoscopy, barium swallow, high-resolution manometry (HRM), pH measurement (either catheter based or using Bravo system), and impedance monitoring of the esophagus are major esophageal function testing techniques that are available in most academic centers and centers of excellence for esophageal function testing. These recording techniques provide key information on the sensory and motor functions of the esophagus that allows one to treat patients based on the physiologic understanding of the patient’s symptoms. Another esophageal function testing technique, which even though has not gained wide popularity and acceptance, is ultrasound (US) imaging of the esophagus using either catheter-based intravascular US imaging probe or US endoscope. The latter is suited for anatomic/morphologic information of the esophageal muscle and identification of lesions that affect esophageal function by compression of the esophagus. On the other hand, catheter-based US imaging provides information on anatomy/morphology as well as functional aspects of esophageal muscle function. In order for US imaging, especially dynamic US imaging, to gain popularity it will have to be user friendly and less expensive. To date, dynamic US imaging has only been used in research laboratories. There is no question though that the static and dynamic US imaging, using either US endoscopes or catheter-based US probes, provides key information of esophageal functions that is unparallel to any other technique.
Laser Doppler blood perfusion measurement of the esophageal wall is another important modality, especially if future testing confirms that “noncardiac” chest pain and proton pump inhibition (PPI)-resistant heartburn are related to low blood perfusion or relative ischemia of the esophageal wall. The goal of this writing is to provide principles underlying these techniques and to update the status of information that these techniques have yielded.
Ultrasound imaging of esophagus
The esophagus is no exception—like the rest of the gastrointestinal tract, its outer muscular coat or muscularis propria is organized into an outer longitudinal and an inner circular muscle layer. Recent studies prove that the longitudinal muscles of the esophagus play an important role in the physiology and pathophysiology of the sensory and motor function of the esophagus and one can study longitudinal muscle function using dynamic US imaging. The esophageal longitudinal muscle starts proximally as 3 bundles: (1) a main longitudinal muscle bundle that originates from the posterior surface of the cricoid cartilage, (2) an accessory muscle bundle from the posterolateral surface of cricoid cartilage, and (3) an accessory muscle bundle from the contralateral surface of the cricopharyngeus muscle. Muscle bundles from the 2 sides quickly fan out to surround the entire circumference of the esophagus, leaving only a triangular space, the area of Laimer that is not surrounded by the longitudinal muscle at the most cranial end of the esophagus. At the caudal end, longitudinal muscle fibers continue into the stomach and some may be inserted in the circular muscle bundles of the lower esophageal sphincter (LES). Contraction of the longitudinal muscles causes axial shortening of esophagus. Circular muscles of the esophagus do not travel in a perfectly circular fashion along the length of the esophagus, instead they are oriented in the helical fashion, especially in the distal part of the esophagus. The angle of these helices increases as one goes toward the distal direction. Because of the helical morphology of circular muscle, part of the axial esophageal shortening during peristalsis is the result of circular muscle contraction. Unlike circular muscle contraction and relaxations, which can be easily recorded by intraluminal pressure probes/manometry, it is difficult to measure contraction and relaxation of the longitudinal muscle. Dynamic US imaging provides information on the local longitudinal muscle contraction and axial shortening of the esophagus.
In the animal experiments, longitudinal muscle contraction can be recorded by surgically implanted strain gauges in the long axis of the esophagus. Longitudinal muscle contraction can also be recorded by radio-opaque markers implanted in the long axis of esophagus (in humans and animals ) in combination with radiographic fluoroscopy, which records motion of the radio-opaque markers as a proxy of longitudinal muscle contraction. Clearly, these studies have provided important information; however they are of limited use for the human experimentation for several obvious reasons: (1) strain gauze implantation is not practical and (2) radiation exposure for prolonged recordings limits use of radio-opaque marker technique. More recently, a sensor that uses magnet and electromagnetic field (Hall effect) has been used to study axial motions of the squamocolumnar junction/esophagogastric junction, as an indirect marker of longitudinal muscle contraction of the esophagus. One of the limitations of all of the methods mentioned earlier is that they do not record longitudinal muscle contraction at one site (local contraction) in the esophagus, like manometry does for circular muscle.
US imaging performed using thin (1–2 mm diameter) intravascular catheters can record longitudinal muscle contraction at a focal point in the esophagus over extended time periods. US imaging also provides other important information, that is, esophageal muscle hypertrophy and luminal distension during peristalsis and gastroesophageal reflux (GER), key esophageal functions that cannot be measured by other modalities. For recording longitudinal muscle contraction, US imaging relies on the “law of mass conservation”, that is, when esophagus shortens in length (axial shortening) it causes a proportional increase in the esophageal muscle cross-sectional area and muscle thickness, which can be visualized and quantitated by US image analysis. The strength of US imaging techniques is that the recording can be done in humans for extended time periods. The major limitations of US imaging are that the equipment is expensive and data analysis is time consuming. The methodology to record US imaging and data analysis has been described in detail in an earlier publication.
Static US imaging
Muscle Hypertrophy in Esophageal Motor Disorders and Its Significance
US imaging, endoscope-based or catheter-based, provides important information of the esophageal muscle thickness in the resting or baseline condition. In normal subjects, both circular and longitudinal muscles are relatively thin (0.75 mm each). Muscle thickness is greater in the distal esophagus and it decreases in the proximal direction. Atropine decreases baseline muscle thickness, which suggests presence of baseline tone in the circular and longitudinal muscles of the esophagus and confirm early studies that used esophageal barostat to measure esophageal tone. Using echo endoscopes, several investigators reported case reports of marked muscle hypertrophy in patients with nonspecific motor disorders and nutcracker esophagus. Systematic assessment of patients with various types of esophageal motor disorders revealed interesting differences in muscle thickness between normal subjects and patients, as well as among patients with various types of motility disorders. Patients with primary or idiopathic motor disorders in general have an increase in the thickness of both circular and longitudinal muscle layers, even though the circular muscle thickness is greater than the longitudinal muscle. The increase in muscle thickness is seen primarily in the distal esophagus and it decreases gradually from distal to the proximal end. Another important finding is that the degree of muscle thickness increase or in other words muscle hypertrophy differs in different motor disorders; generally it follows (not always) the following pattern: muscle thickness in achalasia esophagus > diffuse esophageal spasm > nutcracker esophagus > nonspecific motor disorders ( Fig. 1 ). In the case of a distended esophagus, like in achalasia esophagus, muscle thickness decreases as esophagus becomes dilated; however, the cross-sectional area of muscle that represents the muscle mass is significantly larger in achalasia esophagus than other motor disorders. In a study of 98 consecutive patients referred to the motility laboratory for various symptoms, simultaneous manometry and US imaging were performed to determine the relationship between various motility disorders and muscle hypertrophy. Nearly all patients with primary motility disorders, based on manometry, were found to have an increased thickness of muscularis propria. Interestingly, 25% of patients with normal esophageal manometry also had thicker esophageal muscle than normal subject, albeit of smaller magnitude than seen in patients with typical motility disorders. Even though manometry has been normal in these patients, they had esophageal symptoms of dysphagia, chest pain, and heartburn. One interpretation of these findings is that normal manometry does not guarantee normal esophageal function, and US imaging could be a more sensitive technique than manometry to detect esophageal motor dysfunction.
What does an increase in muscle thickness signify and what is its relevance? Major cause of increase in muscle thickness/muscle hypertrophy in all muscles is overactivity or contraction of the muscle against resistance (afterload). For example, systemic hypertension and aortic stenosis cause hypertrophy of the left ventricle. Animal studies show that outflow obstruction to the esophagus induces hypertrophy/increase in esophageal muscle thickness and alteration in esophageal motor function that is not dissimilar to the one seen in association with primary/idiopathic spastic motor disorders. It may be that the fundamental abnormality in primary/idiopathic motor disorders lies in the LES function, which does not either relax or open up normally, and changes in the esophageal motor function are secondary to the LES dysfunction. The degree of LES dysfunction may determine the severity of esophageal motor dysfunction and muscle hypertrophy. For example, the LES dysfunction is most severe in achalasia esophagus and so is the esophageal body dysfunction and so on. Another pathologic process that causes muscle hypertrophy is inflammation, for example, patients with Crohn disease have hypertrophy of muscles of small intestine. Transforming growth factor-β1 and related growth factors are key players in the inflammation-induced muscle hypertrophy. It is unlikely though that inflammation plays an important role in esophageal muscle hypertrophy because types of inflammation that afflicts the esophagus, that is, reflux-induced esophagitis and eosinophilic esophagitis are not associated with an increase in the muscle thickness. To the contrary, muscle thickness is reduced in patients with reflux esophagitis and it is not altered significantly in eosinophilic esophagitis. Systemic sclerosis or scleroderma esophagus is associated with loss of muscle mass and decrease in the esophageal muscle thickness.
Dynamic US imaging
Patterns of Longitudinal Muscle Contraction in Health and Their Significance to Normal Esophageal Function
Dynamic US imaging of the esophagus is performed using catheter-based, intravascular US probes that are 1 to 2 mm in diameter. They were designed initially to study coronary arteries. These catheters can be easily placed via nose into the esophagus. Using these probes, US imaging of the esophagus can be performed continuously for hours; we have recorded US images for up to 24 hours. Images are usually recorded on a videotape, digitized, and then analyzed, even though one can record images in the digital format directly without using videotape. US images recorded by current systems are cross-sectional/tomographic/B-mode images that can be converted into M-mode US images to display changes in muscle thickness over time. These M-mode US images then can be superimposed on the manometry or other types of recordings to determine the relationship between changes in pressure or other parameters of interest and changes in the muscle thickness. Manometry studies show that during esophageal peristalsis, certain length or a segment of the esophagus, rather than a point location, contracts in a peristaltic or sequential fashion. This segment of circular muscle contraction progresses sequentially from oral to aboral direction. The length of the contracted segment increases as it moves in the caudal direction. Pressure in the contracted segment is distributed in the form of a bell-shaped curve with peak pressure in its middle. US images show that the longitudinal muscle contracts in harmony with the circular muscle; the 2 are precisely coordinated in location and timing. The peak of contraction in the circular and longitudinal layer occurs within 1 second of each other ( Figs. 2 and 3 ). Distension of a balloon in the esophagus also induces contraction and relaxation of the 2 muscle layers, cranial and caudal to the site of distension, respectively. However, that does not mean that the 2 layers cannot contract independent of each other; for example, during transient LES relaxation, a key motor event that allows retrograde transport of gastric contents into the esophagus (vomiting, belching, and reflux) pattern of contraction in the 2 muscle layers is different from peristalsis. Just before the onset of transient LES relaxation, longitudinal muscle contracts independent of circular muscle, starting at the caudal end of the esophagus and it marches in the oral direction. Longitudinal muscle contraction during transient LES relaxation is significantly greater in amplitude and longer in duration than during peristalsis. Another distinct pattern of longitudinal muscle contraction is seen in association with subthreshold stimulation of pharynx during which there is also relaxation of the LES. Longitudinal muscle contracts only at the cranial end of the esophagus, that is, just caudal to the upper esophageal sphincter with subthreshold pharyngeal stimulus. These findings prove that the 2 layers can contract together during peristalsis and independent of each other during transient LES relaxation. Recent studies also prove that the 2 muscle layers are not anchored/cemented to each other, rather they slide against each other during peristalsis and transient LES relaxation (Patel N, Bhargava V, Kim HS, et al. Differential axial shortening of circular and longitudinal muscle during peristalsis and transient lower esophageal sphincter relaxation. Submitted for publication.). Interestingly, they slide in the opposite direction during these 2 motor events, that is, there is greater axial shortening of the circular muscle than the longitudinal muscle during peristalsis and reverse is the case during transient LES relaxation.
Occlusion of the lumen caused by circular muscle contraction and its sequential aboral or oral progression (peristalsis or reverse peristalsis) causes bolus propulsion and retropulsion, respectively. Then what does longitudinal muscle layer, which comprises 50% of the muscle mass of the muscularis propria do? Longitudinal muscle contraction and relaxation results in thickening and thinning of the 2 muscle layers respectively, which provide distinct mechanical advantages. Increase in muscle thickness reduces esophageal wall stress and compliance in the contracted segment, both of which are important for esophageal lumen occlusion, which is an essential element for the bolus propulsion. On the other hand, decrease in esophageal wall thickness in the relaxed segment increases esophageal wall compliance that is also an essential requirement for the bolus propulsion. Another important function of longitudinal muscle contraction is that it causes lengthening of the esophageal segment distal to the site of contraction and axial pull on the LES in the oral direction. Recent studies prove that a pull of the LES in the oral direction induces neurally mediated relaxation of the LES. Along the same lines, it is likely that elongation of esophageal segment distal to contraction site induces its active relaxation (descending relaxation). Relaxation of esophagus and LES distal to the site of contraction allows contracted segment to propel bolus with minimal resistance (see Fig. 3 ). Relaxation of the esophagus distal to the site of the contraction and resulting increase in esophageal wall compliance allow distension of the esophagus. The degree of distension of the esophagus during peristalsis is an indirect marker of active relaxation of esophagus. US imaging allows quantitation of luminal distension. Studies show that similar to contraction, active relaxation of esophagus also occurs in a peristaltic fashion thus allowing bolus to move efficiently during peristalsis.
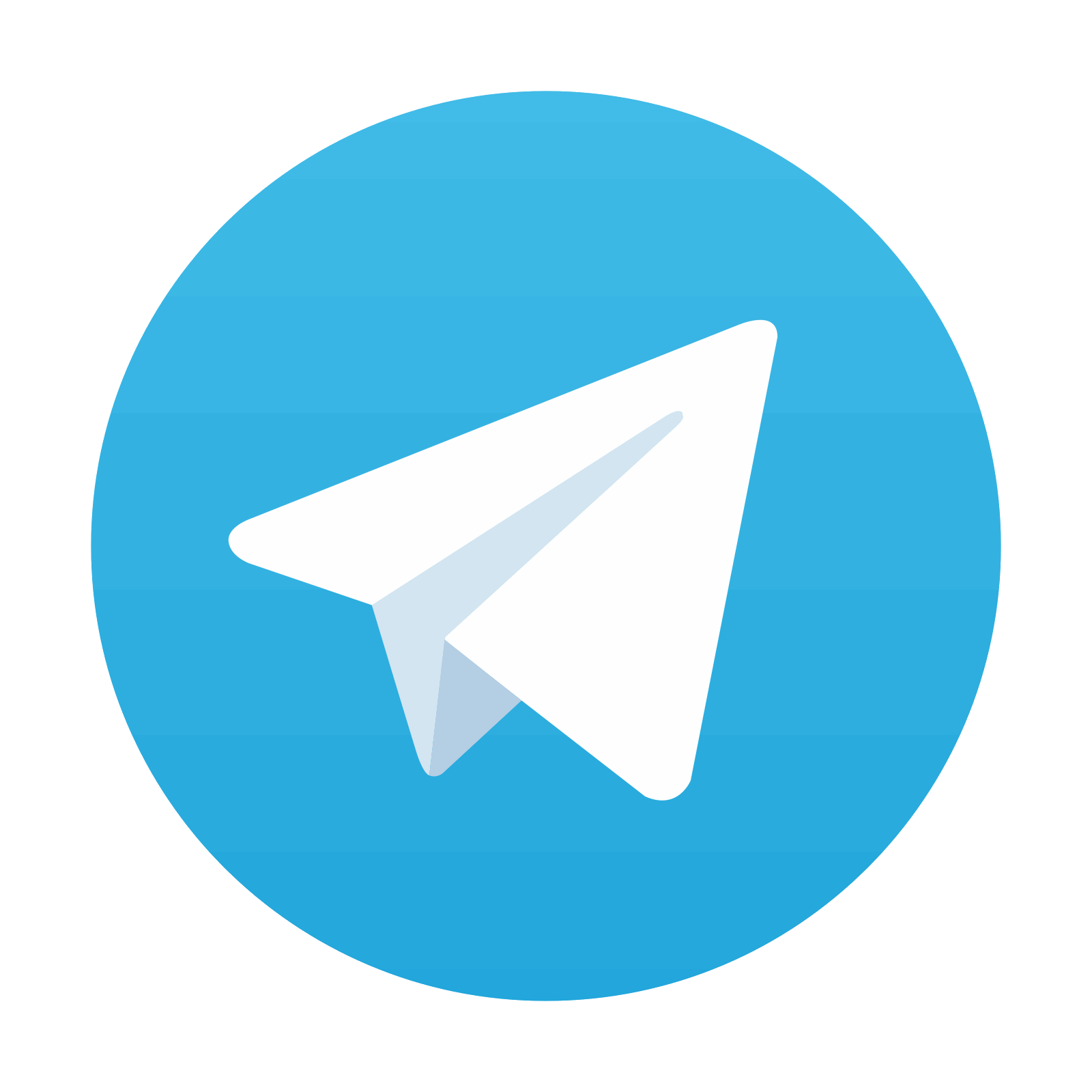
Stay updated, free articles. Join our Telegram channel

Full access? Get Clinical Tree
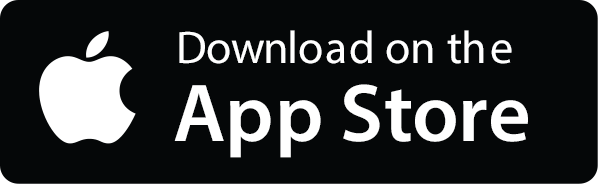
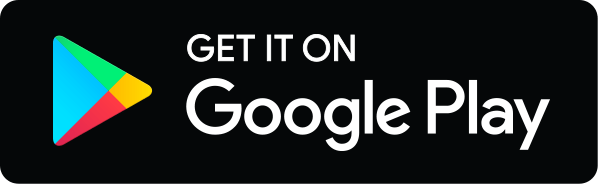