Fig. 22.1
Radial echoendoscope
Radial echoendoscopes can be subdivided into two types: mechanical and electronic (Table 22.1). The mechanical echoendoscope is the predecessor of the electronic technology and currently Olympus is the only company to produce a mechanical model. It contains a transducer with a single piezoelectric element that physically rotates perpendicular to the long axis of the scope generating circumferential, 2D (or B-mode) ultrasonographic images of the gastrointestinal mucosa and underlying structures. Earlier models designed the motor to be part of the operating handle, which made the equipment very heavy. However, more recent models have moved the motor to the base of the cord, which makes the echoendoscope lighter and easier to use.
Table 22.1
Characteristics of current radial echoendoscopes
Radial echoendoscopes | |||||||
---|---|---|---|---|---|---|---|
Brand | Model | Type | Field of view | Scanning range | Display mode | Frequency (MHz) | Channel diameter (mm) |
Olympus | GF-UM160 | Mechanical | 100° | 360° | B-mode | 6/9/10/20 | 2.2 |
GF-UE160-AL5 | Electronic | 100° | 360° | B-mode, M-mode, D-mode, color Doppler, power Doppler | 5/6/7.5/10 | 2.2 | |
Pentax | EG-3670URK | Electronic | 140° | 360° | B-mode, color Doppler | 5/7.5/10 | 2.4 |
Fujinon | EG-530UR2 | Electronic | 140° | 360° | B-mode, M-mode, color Doppler, power Doppler | 5/7.5/10/12 | 2.2 |
The echoendoscope can operate at one of four frequencies, ranging from 5 to 20 mHz. Lower frequencies are ideal for viewing organs located further away from the scope, such as components of the pancreaticobiliary system, while higher frequencies can be used for up-close, detailed assessment of nearer structures, such as the gastrointestinal wall and its layers. Although the quality of these images is fairly good, the technology does create several disadvantages. First and foremost, the fact that the transducer is composed of multiple, mechanical parts makes the scope more prone to technical malfunctions and frequent repairs. In addition, since the transducer needs to physically rotate, it has a plastic cap filled with oil to lubricate and protect the mechanism. However, the oil and cap interfere with the ultrasound signals, creating ring-like artifacts that can impede visibility. Finally, mechanical radial echoendoscopes do not have Doppler or video capabilities, which restricts the type of information that can be obtained from the images.
To overcome these limitations, an electronic radial echoendoscope was developed. Like its mechanical counterpart, this technology utilizes transducers that create a transverse view of the gastrointestinal tract. However, instead of the rotating technology, the scope’s transducer has its crystals arranged in a fixed, circular array, which eliminates a lot of artifact and produces better quality images. In addition, the electronic echoendoscope is equipped with Doppler and tissue harmonic imaging (THI) capabilities. Power and color Doppler technology allows for accurate identification of the vasculature and evaluation of blood flow through tissue. THI is an ultrasound method that receives only second harmonic acoustic signals transmitted through the various tissues of the body, which are subsequently reconstructed into images. Multiple studies have shown that ultrasound systems utilizing the second harmonic increase the sound-to-noise ratio and reduce artifact, thus optimizing the resolution of the images created [1, 2].
Initially, the biggest disadvantage of the electronic radial echoendoscope was the ability to create only a 270° view, leaving behind a 90° “blind spot.” However, this issue was resolved within the past several years with the development of a 360° scope. As a result of these technological advances, the electronic echoendoscope was consistently found superior to the mechanical echoendoscope for visualizing structures within the gastrointestinal tract, in both prospective and retrospective studies [3–5].
Curved Linear Echoendoscopes
The curved linear echoendoscope has imaging capabilities similar to those of the electronic radial echoendoscope, with the advantage of being able to perform procedures such as FNA, cystgastrostomy , and celiac plexus neurolysis (Fig. 22.2). The ultrasound transducer is oriented at an oblique angle to the long axis of the scope, which allows direct, real-time visualization of the needle as it emerges from the instrument channel at the same angle as the transducer. However, this advantage comes at the expense of the scanning range , which is limited to 120–180° at a time, depending on the model (Table 22.2). Therefore, the scope must be manually rotated by the operator to obtain a complete circumferential view.


Fig. 22.2
Linear echoendoscope with needle emerging from the tip
Table 22.2
Characteristics of current linear array echoendoscopes
Linear array echoendoscopes | |||||||
---|---|---|---|---|---|---|---|
Brand | Model | Viewing direction | Field of view | Scanning range | Display mode | Frequency (MHz) | Channel diameter (mm) |
Olympus | GF-UC160P-OL5 | 55° forward oblique | 100° | 150° | B-mode, color Doppler, power Doppler | 7.5 | 2.8 |
GF-UCT160-OL5 | 55° forward oblique | 100° | 150° | B-mode, color Doppler, power Doppler | 7.5 | 3.7 | |
GF-UC140P-AL5 | 55° forward oblique | 100° | 180° | B-mode, M-mode, D-mode, color Doppler, power Doppler | 5/6/7.5/10 | 2.8 | |
GF-UC140T-AL5 | 55° forward oblique | 100° | 180° | B-mode, M-mode, D-mode, color Doppler, power Doppler | 5/6/7.5/10 | 3.7 | |
GF-UCT180 | 55° forward oblique | 100° | 180° | B-mode, M-mode, D-mode, color Doppler, power Doppler, flow mode | 5/6/7.5/10 | ||
Pentax | EG-3870UTK | 50° forward oblique | 120° | 120° | B-mode, color Doppler | 5/6//7.5/10 | 3.8 |
Fujinon | EG-530UT2 | 40° forward oblique | 140° | 124° | B-mode, M-mode, color Doppler, power Doppler | 5/7.5/10/12 | 3.8 |
Theoretically, this may create certain limitations for this type of echoendoscope, as it requires an operator with a more advanced level of experience and cannot generate complete, circumferential images. However, the clinical implications of the differences between the two scopes remain unclear. Several studies have compared the imaging capabilities of the linear and radial technologies in diagnosing and staging various gastrointestinal malignancies, but those have yielded mixed results, with the majority concluding that the two types of scopes were equivalent [6–10].
Although these oblique viewing echoendoscopes have proven both effective and efficient, a new class of forward viewing models is currently being developed to improve upon the original technology. The ultrasound transducer on these echoendoscopes faces straight forward and has a scanning angle of 90°. The instrument channel is positioned so that a needle advanced through it will emerge straight out, parallel to the long axis of the scope, rather than at an angle. The potential advantage of this setup is the ability to perform a targeted puncture of certain lesions, such as those in the uncinate or head of the pancreas, that may be difficult to access by a needle deployed at an angle. In addition, a needle that emerges in a straight line enables more efficient transmission of force compared to an angled needle when puncturing through tissue. And finally, a straight instrument channel without an adjacent elevator function allows for a bigger diameter, which, in turn, can accommodate larger bore needles or other devices.
However, these benefits must be weighed against the smaller scanning range of the forward viewing echoendoscope as well as the absence of the elevator function, which may decrease the accuracy during a needle puncture. To date, only several small studies with very small cohorts have evaluated the forward viewing linear echoendoscopes as the technology is still fairly new. Overall, all the studies seemed to conclude that the forward viewing and oblique viewing technologies are essentially equivalent, both in terms of imaging accuracy and success of therapeutic and diagnostic procedures [11–13]. However, more prospective, randomized controlled trials with larger cohorts are needed to assess more definitively the potential advantages of the newer forward viewing technology.
Endoscopic Ultrasound Processors
How Can We Adjust the Settings of the Echoendoscope Processors to Obtain Optimal Imaging?
As described above, echoendoscopes are equipped with multiple capabilities that can be manipulated by the available ultrasound processors. The endoscope companies have paired with ultrasound manufacturers to develop platforms for their echoendoscopes. Both Pentax and Olympus have partnered with Hitachi Aloka to generate different platforms for their own company’s scopes (Hi Vision Preirus processor for Pentax scopes and ProSound F75 for Olympus scopes, Figs. 22.3 and 22.4). Fujinon also owns its own processor (SU-8000). The main goals when performing EUS are to minimize artifacts, optimize visibility of structures, and utilize the available Doppler technology. Most of the current echoendoscope processors have several different ultrasound modalities. The B-mode generates 2D images in rapid succession to create real-time imaging of anatomic structures.



Fig. 22.3
EUS processor (Hi Vision Preirus, Hitachi Aloka Medical, Wallingford, CT)

Fig. 22.4
EUS processor (ProSound F75, Hitachi Aloka Medical, Wallingford, CT)
When utilizing the B-mode, the GAIN knob can be used to change amplification of all echoes. By turning the gain higher or lower, the brightness of an image can be increased or decreased, respectively. Increasing the gain too high in order to brighten weak echoes can inadvertently brighten artifactual echoes as well, which decreases contrast between structures and blurs the image. The ultrasound processor also contains the time-gain compensation (TGC) function, which is used to improve image uniformity. As ultrasound waves travel through various tissues, they are attenuated due to absorption, scattering, and reflection. In general, the processor compensates for this phenomenon with amplification of echoes of deeper structures under the assumption of uniform attenuation. However, if the tissue has very low density, such as cysts, or very high density, such as blood or liver, this amplification effect can over- or undercompensate for the attenuation. TGC is composed of multiple sliding knobs, each corresponding to a different tissue depth that can be moved left to right to adjust the gain of inappropriately bright or dark portions of an image, creating a more uniform and accurate picture [14].
Evaluation of a particular target can be further optimized using the depth and frequency functions. The depth/range knob can increase or decrease the depth of the viewing field, while different frequencies can be used for finding a particular structure. Most of the current echoendoscopes operate at four different frequencies, 5, 6, 7.5, and 10 mHz. The lower frequencies allow better penetration to the deeper organs at the expense of resolution, which facilitates cursory scanning through multiple structures and organs at one time. Once a specific target is identified, a higher frequency mode can be selected to obtain finer resolution and further characterize the area of interest. The zoom function can be applied when the operator needs magnification. This may be particularly useful for evaluating small structures and distances, especially if they need to be measured accurately. The focus button can be used to converge the ultrasound beam onto a particular point in the image, which can improve lateral resolution. The freeze button allows the image to be frozen and a cine function allows the endosonographer to scroll back over the previous several seconds of images.
The EUS processor also has the capability to evaluate the vasculature. The color Doppler mode allows the assessment of blood flow direction and velocity. The red color generally represents blood flow toward the transducer, while the blue color represents blood flow away from the transducer. The angle between the US wave and the direction of the flow (also known as the insonation angle) should be less than 60° to create a good signal and maximize accuracy of blood flow measurement. If that angle is higher, and especially as it approaches 90°, the perpendicular orientation precludes any blood flow from being detected at all. The angle knob allows adjustments to the angle. When assessing very small vessels, the power Doppler is available. This setting can be very useful during FNA by assisting the endoscopist to avoid blood vessels during puncture, minimizing bleeding risk. Power Doppler, however, cannot provide information about direction of flow or velocity.
Velocity can be measured using the pulsed wave spectral Doppler (PW). A gate is placed inside the blood vessel of interest and flow through the gate is detected as the rate at which the frequency changes, which is subsequently converted into velocity by the fast Fourier transform. The range of the gate can be modified with the sample volume button. Decreasing the sample volume may be helpful when measuring flow in very small vessels or a specific portion of the vessel, while increasing it may be more optimal for evaluating flow in larger arteries and veins, where accurate velocity would be the mean of all the velocities in the vessel. When using larger sample volume, it is also important to appreciate the possibility of detecting artifactual flow information from adjacent vessels. This can be addressed by the Doppler filter function, which removes low-frequency noise. A low-filter setting can be used when analyzing low-flow structures within nonmoving tissue, but it does leave behind some wall noise. High-filter settings are better equipped for high-flow systems within tissue that has an element motion, but may fail to detect lower velocities along the walls of the vessel, which would result in calculating artificially high mean velocity.
What New Technologies Are Available to Improve Endoscopic Ultrasound Imaging?
Contrast-enhanced Ultrasonography
Contrast-enhanced ultrasonography (CE-EUS) is a newer accessory tool for electronic radial and linear echoendoscopes, which allows more accurate characterization of gastrointestinal tumors based on their microvascular patterns. This technique involves injecting a peripheral vein with a contrast agent composed of gas-filled microbubbles enveloped in an albumin or phospholipid shell [15]. After injection, the Doppler or THI functions of the echoendoscope can be used to assess vascular flow in the area of interest. Color or power Doppler accurately identifies hyper- and hypovascular structures, while harmonic imaging evaluates both the extent of the vasculature as well as parenchymal perfusion [16, 17]. In pancreaticobiliary tumors, CE-EUS has been shown to be superior to standard EUS in differentiating different types of neoplasms, distinguishing malignant from benign lymph nodes , and more accurately determining the depth of tumor invasion [18–23].
D Endoscopic Ultrasound
3D ultrasonography is another relatively new tool for endoscopic imaging that reconstructs a sequence of 2D images into multiplanar structures. To ensure accuracy of the reconstruction, the angle and position of the echoendoscope during every acquired 2D image need to be known and the images must be obtained in a rapid sequence to minimize motion artifact [24]. This is easily accomplished with an electronic radial echoendoscope, because an axial view is generated without the need for manual rotation. With linear array echoendoscopes, obtaining an accurate 3D view can be more difficult, as manual rotation of the scope along its longitudinal axis can introduce artifact or error if certain areas are missed [25]. Therefore, operator experience is an integral part of generating accurate 3D representations of the anatomy. Once all the 2D images of interest are obtained, specialized software will import each digitized picture into its appropriate location within a 3D grid, ultimately creating a complete image of the area of interest. Currently, 3D imaging has been shown advantageous for characterizing rectal neoplasms, though this technology has yet to be utilized on a broad scale for evaluating other types of gastrointestinal malignancies, especially pancreatic cancer [26–28].
Endoscopic Ultrasound Elastography
Elastography is a method of determining real-time tissue stiffness, which is a property that can help distinguish malignant from benign lesions . Specialized software is used to measure stiffness by analyzing the changes in EUS images due to vascular pulsations and respiratory movements during tissue compression [29]. This information can then be translated into the degree of elasticity, either as a qualitative or quantitative measure. Qualitative elastography is based on the detection of the degree of tissue compressibility and converting that information into a color scheme overlying the B-image. In this setup, the color blue represents hard tissue, green/yellow is intermediately hard tissue, and red depicts soft tissue; harder tissue corresponds to a higher likelihood of a malignant lesion [30, 31]. Quantitative elastography is a more objective technique that involves converting the differences between tissue elasticity into a strain ratio, which may increase accuracy for differentiating benign from malignant lesions [32, 33]. To date, this software has been primarily employed in evaluating pancreatic masses and further studies are awaited to assess the value of this technique in assessing malignancies of the hepatobiliary tract.
Ultrasound Miniprobes
What Are the Advantages and Limitations of Echoendoscope Miniprobes?
As the applications of EUS continued to grow in the late 1980s, a more streamlined process along with higher resolution images was necessary [34, 35]. One important advancement in the world of EUS was the development of ultrasound miniprobes, also known as high frequency ultrasound sonography (HFUS) (Table 22.3, Fig. 22.5). Miniprobes were aimed at combining endoscopy and EUS in one continuous process, compared with what was considered “traditional” EUS, which encompassed two separate procedures with endoscopy followed by EUS. By passing the miniprobe down the accessory channel of the endoscope , it allowed the gastroenterologist to combine endoscopy and EUS into one procedure. In addition to providing this advantage, the technology behind miniprobes also focused on improving resolution in order to help discern between inflammatory and oncologic processes [36]. Miniprobes also gave gastroenterologists new access through tighter strictures and bile ducts, leading to a more detailed exploration of anatomy previously unavailable to EUS.


Fig. 22.5
Ultrasound miniprobe
Company | Probe model # | Working length (meters) | Probe diameter (mm) | Frequencies (MHz) |
---|---|---|---|---|
Fujinon | PL 1726a PL 1926a PL 2226a | 1.7, 1.9, 2.2 | 2.6 | 12, 15, 20 |
PL 2220a | 2.2 | 2.0 | 12, 15, 20 | |
PL 2226-7.5B | 2.14 | 2.5 | 7.5 | |
Olympus | UM-2R | 2.14 | 2.5 | 12 |
UM-3R | 2.14 | 2.5 | 20 | |
UM-S20-20R | 2.14 | 2.0 | 20 | |
UM-S30-20R | 2.14 | 2.0 | 30 | |
UM-S30-25R | 2.14 | 2.5 | 30 | |
UM-DP12-25Ra | 2.2 | 2.5 | 12 | |
UM-DP20-25Ra | 2.2 | 2.5 | 20 | |
UM-BS20-26R-3 | 2.14 | 2.6 | 20 | |
UM-G20-29R | 2.14 | 2.9 | 20 |
Miniprobes provide high-resolution radial images that can be used during upper endoscopy, enteroscopy, colonoscopy, and endoscopic retrograde cholangiopancreatography (ERCP). In addition to radial images, some miniprobes have dual reconstruction abilities, which provide both radial and linear images. There are two basic types of catheters: electronic and mechanical [37, 38]. Mechanical catheters contain a single ultrasound transducer that produces a 360° image perpendicular to the longitudinal axis of the miniprobe catheter. The transducer cap holds oil, which provides an acoustic interface for the miniprobe. Electronic catheters contain a probe with several fixed ultrasound transducers at the tip, however they do not have a rotating system as found with the mechanical catheters.
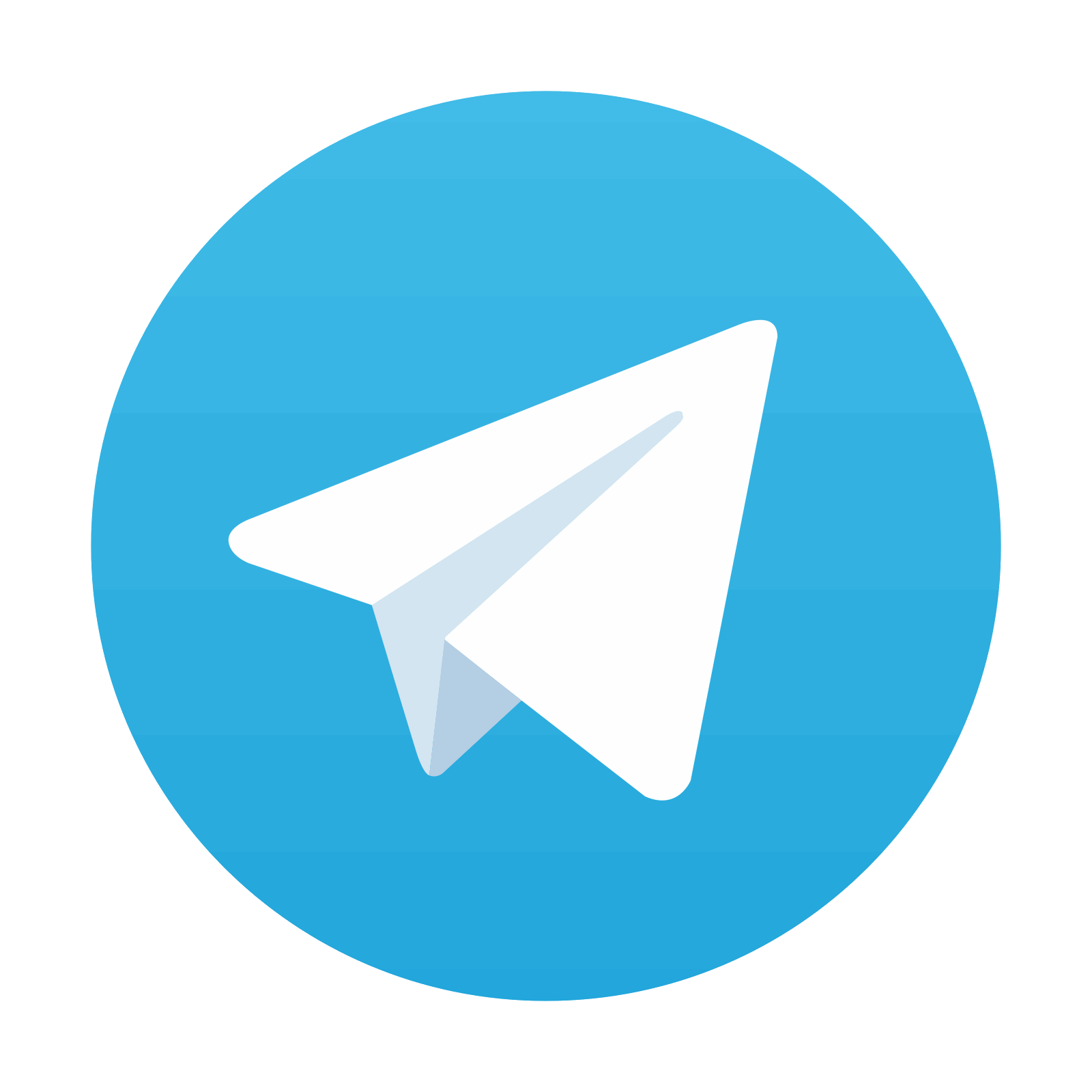
Stay updated, free articles. Join our Telegram channel

Full access? Get Clinical Tree
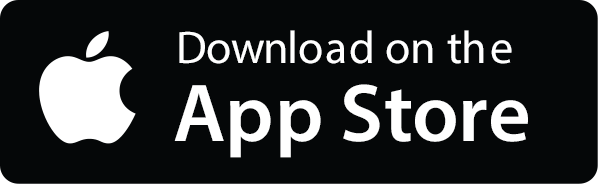
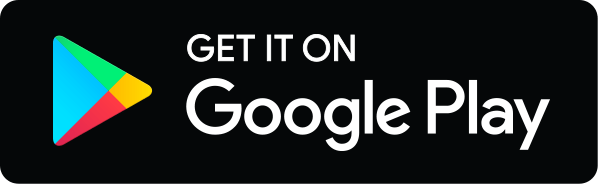