Fig. 1.1
Molecular biology of Lynch syndrome. During replication, DNA polymerase slips on highly repetitive microsatellites. Proficient MMR repairs these slips in normal cells, but they persist in those without MMR. These alterations lead to frameshifts in coding of gene with subsequent loss of gene function. Yates D and Catto J. Distinct patterns and behavior of urothelial carcinoma with respect to anatomical location: How molecular biomarkers can augment clinico-pathological predictors in upper urinary tract tumours. World J Urol 2013; 31(1): 21–9. With kind permission of Springer Science + Business Media
Tumor Spectrum Within Lynch Syndrome
Two patterns of tumor distribution in LS may exist [15]: Type I is characterized by the early onset of right-sided colorectal cancer [16], and type II by extra-colonic tumors, especially within the endometrium and upper urinary tracts (in addition to colorectal cancer). Watson and Lynch [17] reported 23 North American families, with over 7,500 persons, annotated into low and high risk, depending upon their germ line relationship to those affected by cancer. In the high-risk group (n = 1,317), an excess of tumors within the stomach, small bowel, hepatobiliary system, UTUC, and ovary was seen (Table 1.1). In low-risk persons (n = 6,089) no significant excesses of tumors were seen. Heterogeneity was seen with respect to the number of tumors of the upper urinary tract and endometrium, and to a lesser extent ovarian cancer, whilst stomach, small bowel, and hepatobiliary cancers were distributed evenly in all 23 families. Watson and Lynch concluded that whilst endometrial, ovarian, and UTUC were seen in some LS kindred, their evidence did not support the specific existence of two different Lynch syndromes [17]. However, subsequent correlations with MMR mutations have shown differences in tumor distribution, supporting a two-LS model [18]. Currently, kindred are best classified according to the presence of extra-colonic tumors and by the presence and type of MMR mutation.
Tumor site | Observed | Expected | Poisson test p value |
---|---|---|---|
Colorectum | 287 | ||
Endometrium | 53 | ||
Stomach | 17 | 4.1 | <0.001 |
Small intestine | 10 | 0.4 | <0.001 |
Hepatobiliary | 7 | 1.4 | <0.05 |
Bladder | 5 | 4.6 | No sig diff |
Kidney | 3 | 2.8 | No sig diff |
Renal pelvis | 7 | 0.4 | <0.001 |
Ureter | 5 | 0.2 | <0.001 |
Ovary | 13 | 3.6 | <0.001 |
Lung/bronchus | 5 | 12 | <0.05* |
Pancreas | 6 | 4.1 | No sig diff |
Breast | 19 | 22 | No sig diff |
Hematological | 5 | 7.9 | No sig diff |
Skin | 2 | 2 | No sig diff |
Larynx | 1 | 2.2 | No sig diff |
Brain | 3 | 1.9 | No sig diff |
It has been long recognized that UTUC occurs within LS [19–21]. In 1990, Vasen et al. described the tumor spectrum within 24 Dutch families, including 8 UC in 4 kindred [22]. Whilst each of these reports described upper tract UC, there were also cases of bladder and renal cancer within these families. In 1998 Sijmons et al. used the Dutch LS registry to report 50 families (1,321 individuals) [23]. They observed 7 patients with upper tract UC, showing the relative risk of developing these tumors was increased 14-fold when compared to the general population (cumulative lifetime risk of an upper tract UC was 2.6 % with Lynch syndrome, versus 0.25 % (men) and 0.1 % (women) lifetime risk for the general Dutch population). The risks of bladder UC (95 % CI 0.63–3.66) and renal adenocarcinoma (95 % CI 0.85–4.89) were not increased within kindreds. Vasen et al. identified the largest increase in risk of developing kidney, renal pelvis, and ureteric cancer (combined) when they studied 210 proven LS gene mutation carriers, in 19 families [24]. They found that those families with hMSH2 mutations had a relative risk of 75.3 (95 % CI 31.3–180.9) for developing a renal or ureteric malignancy, unlike hMLH1 carriers that showed no increased risk, when compared with the general population. This corresponds to a lifetime’s cumulative risk of 12 % for 70-year-old hMSH2 mutation carriers versus 1.3 % for hMLH1 [18]. Aarnio et al. studied 50 Finnish LS families with proven MMR gene mutations [25]. They also found an increased risk of UC, with a standardized incidence rate (SIR) of 7.6 (95 % CI of 2.5–18). When converted to a cumulative lifetime risk, in agreement with Sijmons et al., this represents a 2–4 % of developing uroepithelial cancer by 70 years of age. Most recently, Skeldon et al. reported an increase risk for bladder UC (as well as upper tract UC) in Canadian LS families [26].
Overall, the risk of developing an UTUC by the age of 70 in LS is between 2 and 4 %, which is around 8- to 16-fold higher than the general population. Urothelial tumors occur in younger patients; for example, the average age at diagnosis is around 56 years old [17] and 58 years old [23], compared to the general population (peak age is between 60 and 80 years old).
Detecting Lynch Syndrome
Clinicians may care for patients with undiagnosed LS [27]. Consensus criteria (Table 1.2) have been defined to aid identification of these patients. As urologists, the typical presentation may be an upper tract UC (of which the distal ureter seems more common) in a young patient (affecting females more commonly than sporadic tumors), without a risk factor for UC (such as a nonsmoker) and with either a family or personal history of cancers within LS [14]. Pathologically, UC within LS have an inverted growth pattern [13] that may be used to suggest the syndrome, and are typically diploid rather than aneuploid.
After the exclusion of familial adenomatous polyposis |
1) At least three family members with colorectal cancer, |
two of whom are first-degree relatives. |
2) At least two generations represented. |
3) At least one individual younger than 50 years old at diagnosis. |
Clinical Implications of Loss of Mismatch Repair
MMR proteins are key to DNA repair. Tumors without MMR generate a larger number of DNA mutations than MMR-proficient cancers. As a consequence, MMR-deficient tumors lose control of many cellular processes, and the consequential tumors are more indolent than expected by their pathological appearance [32, 33]. In addition, the MMR proteins recognize DNA damage induced by certain chemotherapeutic agents, such as alkylating agents (MNNG), base analogues (6-thioguanine), adduct forming agents (cisplatin family), double strand break agents (doxorubicin (adriamycin)), and the fluoropyrimidine anti-metabolites (5-fluorouracil and 5-fluoro-2′-deoxyuridine) [34, 35]. Tumors without MMR fail to recognize DNA damage (from cisplatin) and do not respond by apoptosis. Thus these tumors do not respond to chemotherapy, as well as MMR-proficient cancers [36, 37]. In contrast, MMR-deficient cells are more sensitive to topoisomerase inhibitors, such as camptothecin and etoposide [38].
Implications for Sporadic Upper Tract Urothelial Carcinoma
In colorectal cancer, LS is estimated to account for around 5 % of all cancers. In the remaining 95 % of cancers, around 10 % share molecular features with MMR-deficient tumors, typically by losing MMR through DNA hypermethylation of hMLH1. The prevalence of LS in upper tract UC is unknown, but it is reasonable to assume a similar proportion to colorectal cancer (around 5 %). Between 10 and 15 % of sporadic upper tract UC also lose MMR expression by epigenetic means (also DNA hypermethylation of hMLH1) [13, 39, 40]. It is likely that these tumors will have a similar phenotype to LS cancers, in terms of prognosis and drug resistance.
Low Penetrance Genetic Events
Variation is necessary for the health of a population. This variation may arise at a number of molecular levels, including differences in DNA sequence. Sequence changes are extremely common and affect single DNA bases. SNPs may or may not alter the expression (if the SNP is within a promoter region) or amino acid coding (if exonic) of a protein. Changes in protein sequence may affect the structure and activity of that molecule. In UC, several important variations in gene efficacy have been identified, especially in detoxification and DNA repair pathways. More recently, technological advances allow the comparison of large numbers of SNPs between cases and controls. These experiments have yielded new knowledge into the biology of UC.
Detoxification Pathways
UC mostly arise following exposure to carcinogens, such as those from cigarette smoke or occupational tasks. Protein coding SNPs that affect the abundance and activity of detoxification proteins will prolong or reduce the duration of carcinogen exposure (Fig. 1.2). The two enzymes most implicated in UC carcinogenesis are N-acetyltransferase 2 (NAT2) and glutathione S-transferase M1 (GSTM1) [41]. Carcinogens may be detoxified by NAT2 or GSTM1 in the liver epithelium, before excretion of nonreactive (noncarcinogenic) products. Failure of this detoxification leads to CYP1A2 hydroxylation in the liver and transportation to the urine. In the urinary tract, NAT1 acetylation creates active carcinogenic metabolites. Thus, persons with slow or low NAT2 activity, reduced GSTM1 alleles, or fast/high NAT1 activity will create more urothelial carcinogens than the general population. Several authors have confirmed these effects in the bladder. For example, Garcia-Closas et al. investigated SNPs or deletions in NAT2, NAT1, GSTM1, GSTT1, GSTM3, and GSTP1 in 1,150 Caucasian Spanish patients with bladder UC and 1,149 controls. They identified elevated risks of UC for individuals with deletion of one or both copies of GSTM1 (odds ratio 1.2- and 1.9-fold, respectively) and for NAT2 slow acetylators (1.4 [1.2–1.7]). These SNPs appeared more important in cigarette smokers than never smokers (especially for NAT2), in keeping with their detoxification role.
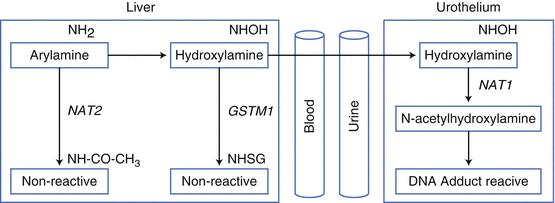
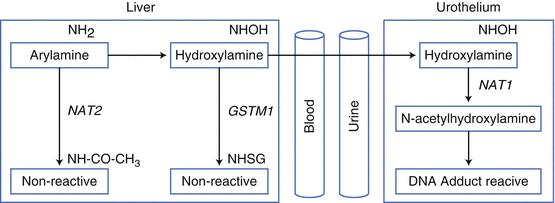
Fig. 1.2
Detoxification of arylamine and UCC carcinogenesis. Arylamines can be detoxified by acetylation (NAT2) in the liver, or hydroxylated by CYP1A2 and transported to the urinary tract. Here they undergo acetylation by NAT1, to form a highly reactive species. Genetic variants that reduce NAT2 activity and increase NAT1 activity increase UCC risk following arylamine exposure (adapted from Jung I, Messing E. Molecular Mechanisms and Pathways in Bladder Cancer Development and Progression. Cancer Control 2000: 7(4):pages 325–334)
While reports regarding bladder UC and detoxification enzyme SNPs are numerous, there are few examining UTUC. Bringuier et al. genotyped CYP1A1/NAT1 SNPs and GSTT1/GSTM1 deletions in 105 patients with renal pelvic UC, and compared to a history of analgesic ingestion or tobacco smoking [42]. Variants SNPs were detected in 6 % for CYP1A1 and 23 % for NAT1, and absent GSTT1 and GSTM1 were found in 12 % and 39 %, respectively. These proportions were similar to general population estimates, and so no association was found with these SNPs, carcinogen exposure, and renal pelvic UC. The authors concluded this may indicate that urinary mutagenicity is less important in renal pelvic than in bladder tumor development, though the sample size of this study does not allow definite conclusions. Katoh et al. examined deletion of GSTM1 and CYP1A1 polymorphisms in 83 UC patients (including 18 with upper tract UC) [43]. They identified an increased risk of UC, but were underpowered for an anatomical subgroup analysis. The same group examined GSTM1 and GSTT1 in 145 UC patients, of which 33 had upper tract disease (10 cases of renal pelvis cancer, 12 cases of ureter cancer, and 11 overlapping cases) [44]. An increased risk of UC was seen for bladder cancer (Odds ratio 1.78 [1.05–3.02]), and perhaps for ureteric cancer (2.00 [0.38–10.41]), but not renal pelvic disease (0.44 [0.07–2.66]). However, the low sample size prevented sufficiently powerful analysis to determine any true associations.
Other workers have focused on cytosolic sulfotransferases (SULT) [45], which detoxify environmental chemicals and activate mutagens through the conjugation of sulfo groups. There are two major families of sulfotransferases (phenol (SULT1A1) and hydroxysteroid (SULT2A)). SULT1A1 is expressed in liver, lung, and kidney and has a polymorphism (SULT1A1*2) in exon 7 producing a histidine substitution of an arginine residue, which decreases enzymatic activity (to alter the rate of mutagen and pro-carcinogen detoxification/bioactivation rates). Rourpet et al. genotyped 268 patients with upper tract UC and 268 healthy matched controls. The SULT1A1*2 polymorphism was significantly more common in patients than controls (37 % versus 29 %) and conferred a higher risk of UC (odds ratio = 2.18 [1.28–3.69]).
Genome-Wide SNP Analysis in UC
Most recently, technological breakthroughs have enabled massive parallel SNP analysis in single experiments. This has allowed large studies to examine millions of coding SNPS in well-powered cohorts. Collaborative consortia have analyzed 11,914 UC bladder cancer cases and 53,395 matched controls for up to 591,637 SNPs [46]. Several key regions and SNPs have been identified, including 3q28, 4p16.3, 8q24.21, 8q24.3, 22q13.1, 19q12, and 2q37.1. With regard to UC in the upper tract, Roupret et al. analyzed rs9642880 on chromosome 8q24 in 261 patients with upper urinary tract UC and 261 matched controls [47]. They identified the T/T genotype increased the risk of UC (odds ratio = 1.72 (1.1–2.8) was associated with aggressive tumors when stratified by stage and grade G2 (p = 0.04).
Acquired Exposures and Upper Tract Urothelial Carcinogenesis
Exposures Shared by Urothelial Carcinoma in the Bladder and Upper Tracts
Regardless of genetic predisposition, most UC arise following exogenous carcinogen exposure. As detailed, a gene/environmental interaction occurs between carcinogens and an individual’s ability to detoxify these chemicals. The vast majority of carcinogens for UC are thought to be derived from either tobacco smoke or occupational exposures.
Cigarette Smoking
It is estimated that cigarette smoking is the major carcinogenic exposure for around 50 % of UC [48]. Tobacco smoke contains aromatic amines, such as beta-naphthylamine and 4-aminobiphenyl, and polycyclic aromatic hydrocarbons known to cause UC [49]. Exposure to these chemicals varies with proximity (direct smokers versus passive inhalation of environmental tobacco smoke (ETS)), dose and chronicity (combined as pack years), tobacco type (dark (higher concentrations of N-nitrosamine and 2-napthylamine) versus blonde tobacco [49, 50]), inhalation into the mouth or chest [48], and current behavior (ex versus current smokers).
Cigarette smoke inhalation increases the risk of developing an UTUC by three- to sevenfold, compared to the general population [51–53]. As with bladder UC, this risk is dose adjusted, and doubles from 2.4-fold (history of <20 cigarettes per day) to 4.8-fold (>40 cigarettes per day) with increasing exposures [52]. This risk is halved with cessation of smoking for more than 10 years. Due to gender-specific smoking patterns, the same authors estimated that 7 of 10 cancers of the renal pelvis and ureter in men, and almost 4 of 10 among women were caused by smoking.
Occupational Carcinogen Exposure
It is estimated that between 5 and 15 % of UC arise following occupation carcinogen exposure and that there is a male predominance [54, 55]. Occupational carcinogens are best divided by chemical structure into aromatic amines, polycyclic aromatic hydrocarbons (PAHs), and tobacco smoke/combustion products (such as firefighters and bar staff). Exposure to aromatic amines occurs in printers, painters, rubber and dye workers, hairdresser, and the textile industry. Exposure to PAHs occurs in aluminum refining, diesel fumes (drivers, seamen, miners, etc.), metal workers, and medical staff (such as nurses).
As with other aspects of UC biology, most data exist for bladder cancer, rather than for UTUC. Wilson et al. surveyed 4.2 million Swedish persons, including 1,374 with renal pelvic and 21,567 with bladder UC for occupational history [56]. Most (80 %) has stayed in the same occupational group over the 16 years follow-up, including 16 % of those with upper tract UC were involved in metal work. When bladder and upper tract UC were compared there were many similarities. However, several occupations appeared more specific to the renal pelvices, including pharmacists, journalists, business executives, food-related workers, food process workers, and chemical workers for females, and insurance clerks, butchers, cleaners and pulp grinding, metal manufacturing, scientific/surgical instruments, insurance, and legal industries for males. McCredie et al. reported occupation risks for kidney cancer and renal pelvic UC in New South Wales [57]. When compared, an increased risk of only UC was seen in the dry cleaning (relative risk = 4.68 [1.32–16.56]) and Iron and Steel industries (2.13 [1.04–4.39]).
Acquired Exposures more Specific to UTUC
Aristolochic Acid
The Aristolochiaceae family of herbaceous plants, in particular, members of the genus Aristolochia, has been used for medicinal purposes for more than 2,500 years [58, 59]. Aristolochia species contain a family of structurally related nitrophenanthrene carboxylic acids, principally aristolochic acid I (AA-I) and aristolochic acid II (AA-II) [60] (Fig. 1.3). More than a century ago, Pohl noted the nephrotoxic effects of these compounds by administering to rabbits extracts prepared from seeds and roots of Aristolochia clematitis [61]. More recently, Mengs further documented in rodents the acute and chronic toxicities of purified AAs [62], including their carcinogenic effects [63, 64].
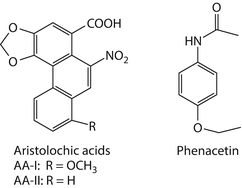
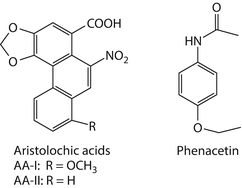
Fig. 1.3
Chemical structures of aristolochic acids (AA)-I and -II, and phenacetin, carcinogens associated with upper tract urothelial cancers
Less than 20 years have passed since exposure to AA was identified as a risk factor for UTUC in humans [65]. This association, manifested as an iatrogenic disease in Europe and Asia and as an environmental disease in the Balkans, resulted in AAs being classified formally as Group I human carcinogens [66, 67].
Chinese Herbs Nephropathy in Belgium
In the early 1990s, a direct connection was established between AA exposure and human disease, made possible by studies of a cohort of Belgian women exposed inadvertently to AA [2, 3]. This group was identified initially as a cluster of young women in Brussels with end-stage renal disease (ESRD) of unknown origin [68]. A shared etiology was suspected due to demographic features and grouping of cases. Further investigation revealed that all of these women participated in a weight-loss regimen that included the use of various drugs and Chinese herbs. Suspicions fell on Stephania tetrandra, an herb introduced into the formulation in May 1990 and used through July 1992. However, as S. tetrandra was known not to be toxic, the cause of the renal disease affecting the Belgian women remained a mystery until it was shown that this herb had been replaced with AA-containing Aristolochia fangchi. Proof of exposure to AA was subsequently validated by the (1) chemical identification of AA in the herbs imported from China [69] and (2) detection of unique AA metabolites bound covalently to DNA in tissues obtained from these patients [65, 70]. Based on prescription data and the AA content of the herb, the average dose of AA was estimated as 0.025 mg/kg body weight per day, consumed over 13 months [2]. Despite comparable dosing, nephropathy developed in only 5 % of the 1,500–2,000 Belgians who were exposed to AA, suggesting a significant role for gene variants in determining individual susceptibility to disease.
The renal disease affecting the Belgian patients was originally named Chinese herbs nephropathy (CHN) and later retitled aristolochic acid nephropathy (AAN) to more accurately reflect the etiology of this disease [71]. AAN is a chronic, progressive, and irreversible tubulointerstitial disease characterized by renal insufficiency, pronounced and early anemia, low molecular weight (tubular) proteinuria, and variable glycosuria [72, 73]. The histopathology of AAN has several defining features including (1) widespread atrophy of proximal tubules, with a gradient of injury decreasing in severity from outer to inner cortex; (2) interstitial fibrosis, mostly confined to the cortex; (3) absence of significant inflammation; and (4) relative sparing of glomeruli [72, 74].
The initial connection between AA exposure and urothelial malignancies arose from observations of three Belgian AAN patients who underwent kidney transplantation for ESRD [72]. All had used the AA-containing herbal product for 1–1.5 years and had discontinued its use 3–5 months prior to surgery. Histopathological review of the native upper urinary tract revealed the widespread presence of mild-to-moderate urothelial atypia and focal squamous metaplasia. Over the following year, one patient developed UC affecting the bladder, remnant ureter, and the contralateral ureter and renal pelvis [75].
The carcinogenic potential of AA was appreciated more fully as similar upper tract lesions were observed in other Belgian patients with AAN [65, 73, 76]. Concerns regarding the high risk of developing UTUC prompted the recommendation of prophylactic bilateral nephroureterectomy in this population [65, 76]. This strategy proved effective as renal pelvic and/or ureteral tumors were detected in over 40 % of the patients who opted for this surgery [77]. In one group of ten patients who received transplants 9–67 months after discontinuing use of the herbal remedy (mean use duration of 20 months), four had developed carcinoma in situ, present in three patients in both the ureter and renal pelvis, along with papillary upper tract UC in one case [76]. Atypia of the papillary collecting ducts and urothelium was noted in all ten patients.
In a second set of 39 patients with AAN, UC was present in 18, all confined to the upper urinary tract except for one incidence of bladder cancer; 19 of the remaining cases showed mild-to-moderate dysplasia, while no lesions were found in 2 patients [65]. Aristolactam–DNA adducts, products of AA metabolism, were detected in renal cortex and tumor tissues, providing irrefutable evidence of prior exposure to AA [65]. Retrospective analyses of exposure data for this cohort revealed a correlation between the risk of developing UTUC with cumulative dose of AA.
Although UTUC is the primary cancer caused by AA in humans, long-term follow-up studies of the Belgian cohort revealed a heightened risk of UC of the bladder, which developed in 15/38 AAN patients within 15 years following bilateral nephroureterectomy and renal transplant [77, 78]. As 80 % of these bladder cancer patients were diagnosed with UTUC at the time of surgery, these tumors may have originated from malignant cells shed from upper tract tumors and seeded in the bladder. However, 20 % of these tumors occurred in patients with no history of UTUC, suggesting that bladder malignancies may arise from a direct carcinogenic effect of AA on the bladder urothelium. Given its long-term carcinogenic potential, vigilant screening of the entire urinary tract is considered prudent practice for follow-up of patients with known or suspected exposure to AA [77].
Balkan Endemic Nephropathy
Balkan endemic nephropathy (BEN) is an acronym applied to a chronic, progressive, tubulointerstitial renal disease, limited to residents of rural villages located near tributaries of the Danube River in Bosnia and Herzogovina, Bulgaria, Croatia, Romania, and Serbia [79]. First reported in the late 1950s, BEN is estimated to affect at least 25,000 men and women in countries harboring this disease; a far larger number of individuals are believed to be at risk [80].
UTUC eventually develop in approximately half of all patients diagnosed with BEN. The prevalence of this cancer in certain endemic regions can be as much as 100-fold greater than in the country as a whole [81]. Data from a review of BEN-associated UTUC were used to explore the epidemiology of this otherwise rare cancer over the past 50 years [82, 83]. Until recently, the etiology of UTUC associated with BEN remained obscure. A variety of environmental mutagens, including mycotoxins and heavy metals, have been systematically investigated as potential causative agents of BEN/UTUC [84]. Ochratoxin A (OTA) had been the principal focus of this research. However, solid epidemiologic evidence supporting an association between exposure to OTA and the prevalence of BEN/UTUC in endemic regions is lacking [85]. In contrast, the studies summarized below support strongly the hypothesis that chronic, low-dose dietary poisoning by AA accounts for all of the important characteristics of BEN, including its geographical distribution, and the increased risk of developing UTUC.
The pathophysiology of BEN bears a striking resemblance to that observed in Belgian women with CHN/AAN; however, residents of endemic villages in Croatia deny the use of herbs containing Aristolochia [86]. An alternative route of exposure, first suggested by Ivić [87], is based on the frequent occurrence of Aristolochia clematitis as a weed growing in local wheat fields. Ivić observed that the traditional methods used for harvesting and milling of wheat permitted seeds of A. clematitis to comingle with the wheat grain used to prepare home-baked bread, a dietary staple among residents of all endemic regions. Thirty-five years later, these astute field observations were confirmed by Hranjeć et al. [88].
Molecular epidemiologic evidence that patients with BEN/UTUC had been previously exposed to aristolochic acid was provided by Grollman, Jelaković, and their colleagues [89, 90], who detected aristolactam (AL)–DNA adducts in the renal cortex of 70 % of these individuals. Their presence in renal tissue reflects active transport of AAs by the renal proximal tubule [91]. Enzymatic nitroreduction of AA yields a product that undergoes sulfonation [92], generating reactive intermediates that form covalent aristolactam (AL) adducts with dA and dG residues in DNA. Lower levels of these adducts are found in the urothelium. The steady-state level of AL-dA adducts in target tissues represents an integration of external exposure with interindividual variability in metabolism and other cellular processes, providing a link between exposure to AA and its cytotoxic and carcinogenic effects. Moreover, AL-dA adducts are highly resistant to global genomic nucleotide excision repair [93], leading to their persistence in target tissues, enhancing their usefulness as biomarkers of exposure. Thus, among Belgian women with CHN, AL-DNA adducts could be detected in the renal cortex 36–89 months following their last exposure to AA [65].
A:T-to-T:A transversions, predicted by the miscoding properties of AL-dA adducts in mammalian cells [94], represent only 5 % of somatic TP53 mutations in all types of cancers combined [95], yet they dominate the TP53 mutation spectrum in AA-induced UTUC [90, 96]. A-to-T transversions constitute 58 % of TP53 sequence changes in UTUC linked to AA exposure but less than 2 % in UTUC of patients with no apparent exposure to AA [97]. Thus, the proportion of A:T-to-T:A mutations in UTUC, coupled with their predominance at splice sites and strong bias for the non-transcribed strand [96], was established as a mutational signature for exposure to AA [97].
Taken together, these molecular epidemiologic studies document a causal relationship between AA and BEN/UTUC. In commenting on this research, DeBroe proposed that BEN/UTUC and CHN should be considered a single clinical entity, namely AAN [98].
Herbal Medicine Use in Taiwan
The earliest mention of Aristolochia in China dates to the fifth century ad; references to various species of this herb appear in virtually all major treatises of traditional Chinese medicine that have appeared since that time [99]. Remarkably, descriptions of the medicinal use of Aristolochia rarely mention intrinsic toxicity, although the doses employed are similar to those in the Belgian cohort. To address this enigma, the molecular epidemiologic approach used to explore the etiology of BEN [90] was adopted for studies in Taiwan, where the incidence of UTUC is reported to be the highest of any country in the world [100]. Moreover, the demographics of UTUC in Taiwan mimic that in the endemic regions of the Balkans.
In Taiwan, a systematic analysis of the national prescription reimbursement database between 1997 and 2003 suggested that approximately one-third of the entire country’s population had been exposed to herbs containing, or likely to contain, AA [101]. Additionally, a linear dose–response relationship has been demonstrated between the consumption of herbal remedies containing AA and the risk of developing UC [100].
Subjects of the study [102, 103], selected from among 212 consecutive Taiwanese UTUC patients who received nephroureterectomies between 1999 and 2011, included 151 patients with UTUC, and 25 cases of renal cell carcinoma (RCC) to serve as controls. Unequivocal evidence of exposure of this cohort to AA is reflected by the observation that 60 % of all cases of UTUC and 84 % of patients with A:T-to-T:A mutations in TP53 contained AL-dA adducts in their renal cortex. AL-dA adducts also were present in 60 % of patients with RCC; however, in this group, A:T-to-T:A mutations were not detected in TP53. In the Taiwanese cohort, 60 % of women and 50 % of men with TP53 mutations displayed signature A:T-to-T:A mutations. Remarkably, despite significant differences in the dose, frequency, and timing of exposure to AA, the overall distribution and positions of A:T-to-T:A transversions in the TP53 gene of patients with UTUC were almost identical to those observed in the Balkan cohort [103].
An unusually large number of splice-site mutations are observed in AA-associated UTUC. And, when TP53 mutational data derived from 97 patients with UTUC/BEN [96] are combined with data with 151 UTUC patients from Taiwan [103], all 5′-AG acceptor sites in TP53 were found to be mutated at least once, with introns 6 and 8 identified as prominent hotspots. This unusual finding is consistent with the preferential targeting of adenine residues in DNA by AA [104].
In the Taiwanese cohort, the oncogenes, FGFR3 and HRAS, appear to be activated by mutations induced by AA. As in TP53, adenine residues mutated in FGFR3 codon 373 and HRAS codon 61 are found invariably on the non-transcribed strand. The majority of this subgroup of cases had been exposed to AA.
In conclusion, AA, an intrinsic component of all Aristolochia herbal remedies, contributes significantly to the incidence of UTUC in Taiwan. As the practice of traditional Chinese herbal medicine in Taiwan mirrors that of mainland China and the carcinogenic effects of AA do not manifest themselves until 30 years after exposure, this finding, as discussed below, has implications for public health in China, India, and other countries where Aristolochia herbs have been traditionally used for the treatment and prevention of disease in recent years [105].
Global Prevalence of AA-Induced UTUC
Aristolochia plants are components of herbal remedies used in the practice of (1) Traditional Chinese Medicine (TCM) in China and other Asian countries; (2) Kampo medicine in Japan; and (3) Ayurvedic medicine in India and South Asia. These practices, along with the traditional use of Aristolochia sp. for medicinal purposes in various regions of Africa and in South and Central America, pose a significant global health threat [58, 103, 106, 107]. Furthermore, the growing popularity of alternative medicine in Western countries has led to sporadic cases of nephropathy and/or UTUC related to Aristolochia use. Although many governments, including the US, have banned the importation of Aristolochia products, while others prohibit the use of this herb for medicinal purposes, over-the-counter formulations containing AA remain available for purchase in local herb shops and via the Internet [108]. Some suppliers fail to disclose that Aristolochia is a component of their herbal product, posing a hidden danger to consumers [109, 110].
Worldwide, more than 300 cases of UTUC related to AA exposure via herbal medicines have been reported [111–113]. Many cases go unrecognized due to the long (20–40 year) latency period between AA exposure and detection of cancer, resulting in underestimation of true prevalence. Sporadic cases of UTUC linked to the medicinal use of Aristolochia plants have been reported from Hong Kong, Japan, Australia, UK, and the United States, and there is documented evidence of widespread disease in China and Taiwan (see section above), countries with long histories of Aristolochia use. In China, renal transplant or chronic hemodialysis patients with a prior history of Aristolochia use have a greater risk of developing urothelial malignancies [111, 114, 115]. AA exposure also is associated with an increased incidence of contralateral metachronous UTUC in China [116].
Phenacetin and Analgesic Nephropathy
Phenacetin (p-ethoxyacetanilide; Fig. 1.3), a pro-drug for acetaminophen, is a synthetic analgesic antipyretic agent that was first introduced into the pharmaceutical market in 1897. The original formulations, sold over-the-counter under various trade names, such as Anacin® and Bex®, typically contained phenacetin in combination with other drugs including caffeine, aspirin, or phenazone. Due to their widespread availability and efficacy, phenacetin-containing analgesic mixtures were highly popular in Europe, Australia, and North America for much of the twentieth century. However, in the 1950s, safety concerns emerged when the habitual use of phenacetin was linked to renal insufficiency and tubulointerstitial disease [117–119], a condition known as analgesic nephropathy. Renal papillary necrosis is a frequent and distinctive feature of this disease. In the 1960s, Hultengren made the etiologic connection between abuse of phenacetin-containing analgesics and the development of UTUC through a study of six Swedish patients diagnosed with both papillary necrosis and UC of the renal pelvis [120]. All but one of the patients had a history of phenacetin addiction, with reported cumulative doses ranging up to 10 kg. Reports of similar cases soon followed, so that by 1983 more than 400 patients with UTUC worldwide, particularly in Australia, Switzerland, Belgium, Denmark, and Sweden, had been linked to excessive habitual use of phenacetin [118, 119]. The geographic distribution of many cases aligned closely with known sites of phenacetin hyperconsumption and analgesic nephropathy [118, 119, 121].
An estimated 8–10 % of patients diagnosed with nephropathy due to phenacetin abuse eventually develop urothelial malignancies [122]. The upper urinary tract appears to be the preferred target tissue, as UC rates are 77 and 89 times higher in the renal pelvis and the ureter, respectively, compared to only seven times higher in the bladder, among patients who consume excessive amounts of phenacetin [118]. These tumors are often multifocal, can be bilateral, and are more common in females since this gender appears to be more likely to abuse phenacetin-containing analgesics. The majority of patients with phenacetin-linked UTUC have renal insufficiency and renal papillary necrosis at the time of clinical presentation. The incidence of UTUC is associated with an average cumulative consumption of 9 kg phenacetin, a mean exposure duration of 17 years, and a mean induction period of 22 years [118].
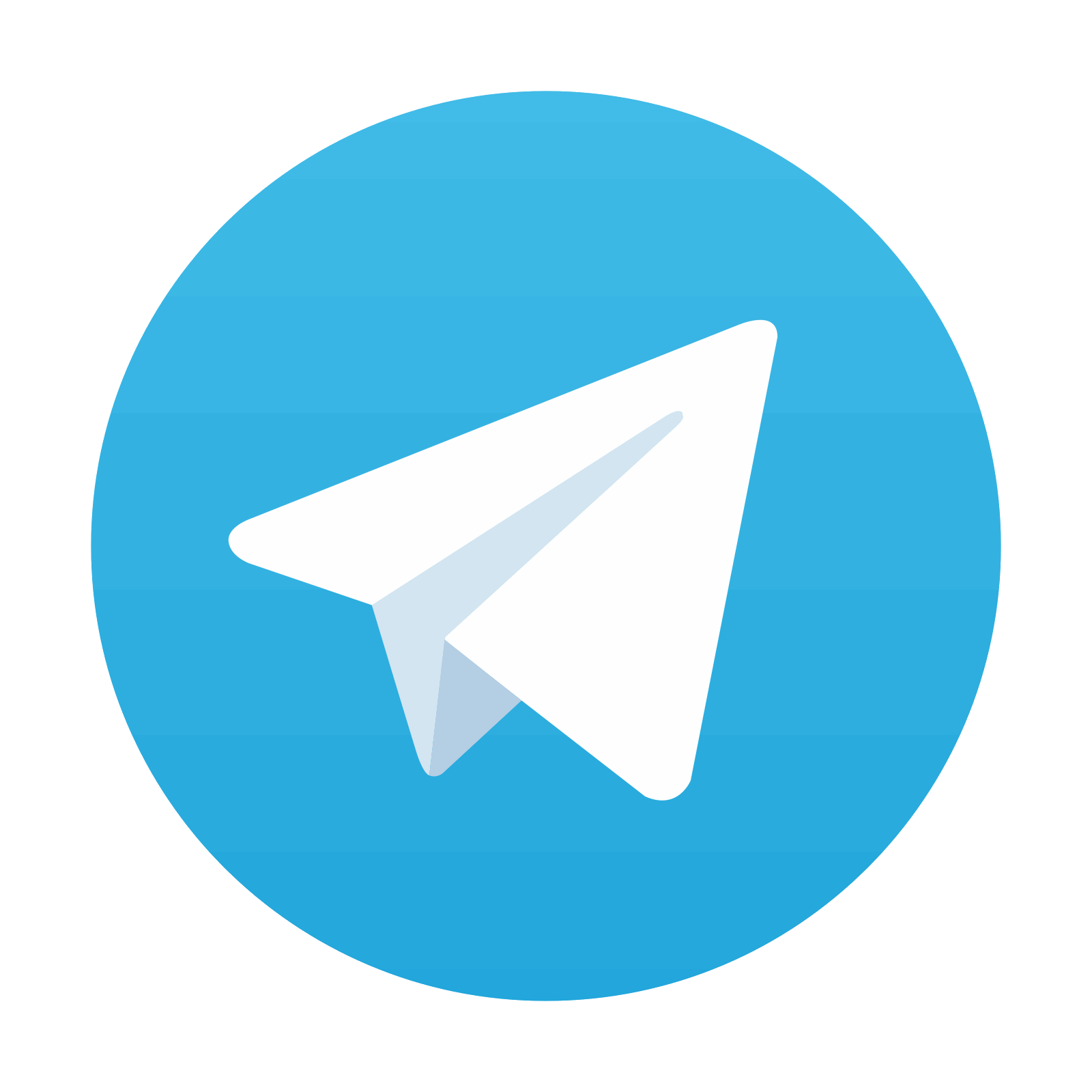
Stay updated, free articles. Join our Telegram channel

Full access? Get Clinical Tree
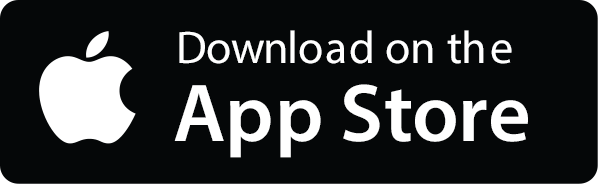
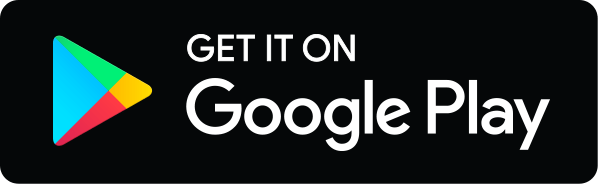
