CHAPTER 17 Electron Microscopy and Other Techniques
Introduction
This chapter will focus primarily on the role of transmission electron microscopy in the assessment of liver ultrastructure and disease. It also describes, in brief, the principles and uses of other methodologies. The special conditions required for tissue processing in each of these techniques (Table 17.1) should be carefully planned for in advance of obtaining specimens. While some of these methods are not universally available in pathology departments, other departments at one’s institution or at other centres of investigation may be consulted in cases of particular diagnostic or research interest. Procedures for fixation and processing for transmission electron microscopy are available in several of the General reading references at the end of the chapter.
Table 17.1 Liver tissue processing for various techniques
Technique | Tissue preparation |
---|---|
Transmission electron microscopy | Glutaraldehyde fixation |
Scanning electron microscopy | Perfusion-fixation; critical point drying; coating with gold or platinum |
Immunoelectron microscopy | Glutaraldehyde/paraformaldehyde fixation |
Immunoperoxidase of tissue sections | Fixation in 10% neutral formalin or alternative fixative |
Immunoperoxidase and immunofluorescence of frozen sections | Snap freeze after embedding in OCT compound |
In situ hybridisation | Snap freeze after embedding in OCT compound |
Flow cytometry | Fresh tissue |
Confocal laser scanning microscopy | Snap freeze after embedding in OCT compound |
Laser capture microdissection | Conventional tissue sections for light microscopy |
Gene array analysis | Snap freeze in liquid nitrogen; store at −80oC |
OCT, optimal cutting temperature.
Electron microscopy of liver biopsies
Transmission electron microscopy (TEM) continues to provide important information about the normal cellular and extracellular constituents of the liver and their alterations in disease. Recent interest in the relationships between the various sinusoidal cells of the liver has benefited from TEM studies1,2 as has investigations of hepatic progenitor cells.3 Data from standard TEM studies can be enhanced by the application of immunohistochemical stains (see Immunoelectron microscopy), digitised three-dimensional computer reconstructions4–6 and morphometry. TEM is sometimes limited by the lack of specificity of certain ultrastructural changes and the problem of sampling error in lesions that may not be uniformly distributed. The first of these limitations is well illustrated in cholestasis; various features of cholestasis such as loss of canalicular microvilli are easily recognised under the electron microscope, but many causes produce these changes. Sampling error can sometimes be reduced by the combination of light and electron microscopy in a single instrument.7
In diagnostic work TEM should be seriously considered under five circumstances:
1. To establish the nature of an inborn error of metabolism. In a number of storage diseases the ultrastructural changes are diagnostic or give an indication of the type of disease to be considered.8,9 Specific features are seen, for example, in type II glycogenosis, in Gaucher’s disease and in Niemann–Pick disease (Fig. 17.1). Storage diseases can and should often be diagnosed by other, usually biochemical, methods, but even then electron microscopy can reduce the period of investigation by drawing attention to a likely diagnosis. Electron microscopy may show whether a liver-cell pigment is lipofuscin or the pigment of the Dubin–Johnson syndrome (Fig. 17.2), and can therefore be helpful when this syndrome is suspected but not fully proved by light microscopy.10 In some patients with Wilson’s disease characteristic changes may be seen in liver-cell mitochondria (see below).
2. To establish the presence of viral infection. Electron microscopy of liver biopsies may prove to be important when serological test results or cultures for suspected viral infection are unavailable or incomplete. Both intranuclear and intracytoplasmic virions may be identified by the appearances of their spherical or hexagonal capsids, dense core material, surface envelopes and paracrystalline and lattice-like arrays. These features can be compared with published micrographs for identification of the candidate virion.11,12 For example, some adult patients with the unusual finding of giant-cell hepatitis on routine light microscopy have been shown to have paramyxovirus-like particles in the liver as a result of electron microscopic studies of biopsy material.13,14 Electron microscopy can also be applied to cell cultures, as shown in a recent study demonstrating 50–90 nm hepatitis C virions.15 Glutaraldehyde fixation of biopsy specimens is preferred, but viral particles can also be identified in formalin-fixed tissues which are washed and then processed for electron microscopy.
3. To establish the nature of a tumour of doubtful histogenesis. The ultrastructural features of many tumours, including neuroendocrine tumours and malignant melanomas, help in making a firm diagnosis.16 The more obvious features such as neurosecretory granules in neuroendocrine tumours survive paraffin embedding; re-embedding of paraffin material for electron microscopy should therefore be considered.
4. To establish the presence of specific drug-related changes. In liver damage due to a small number of drugs, including perhexiline maleate17 and amiodarone,18–21 hepatocytes contain lysosomes filled with lamellar phospholipid material (Fig. 17.3).
5. To provide material for research. Electron microscopy offers wide potential for research into human liver disease, and it may be that future research will increase the diagnostic value of electron microscopy in this field. If liver biopsy is performed in a patient having a disease with potentially helpful or interesting ultrastructural features, small pieces of the specimen can be embedded for electron microscopy and stored indefinitely in block form. The extent to which this is done clearly depends on the resources of the particular laboratory.

Figure 17.3 Amiodarone-induced phospholipidosis.
(Illustration kindly provided by Dr S. Poucell and Professor M. J. Phillips, Toronto, Canada.)
The normal liver and examples of ultrastructural changes in disease
The following description of the liver under the transmission electron microscope is a general one. It should be noted that the quality of fixation will influence the appearance of cells and organelles. The labels in the description of normal liver refer to Figs 17.4 and 17.5.
Hepatocyte (liver cell, parenchymal cell)
Hepatocytes have similar features in different lobular regions but vary in detailed structure. For example, there are more lysosomes and mitochondria in periportal than in perivenular hepatocytes, while the converse is true for the smooth-surfaced endoplasmic reticulum. The hepatocyte is a highly polarised cell with surfaces facing the space of Disse, other hepatocytes and the bile canaliculus. The plasma membrane is specialised in these three areas. Many microvilli project into the space of Disse and into the bile canaliculus. This is a potential space formed by two or three hepatocytes in normal liver, and sometimes more in disease. The intercellular membrane of the hepatocyte is relatively smooth and forms several types of intercellular junctions.
The nucleus
The nucleus (N) is normally limited by a double membrane, the nuclear envelope, which is continuous with the rough-surfaced endoplasmic reticulum. The nuclear envelope has small pores which are thought to serve as a route of communication between the nucleoplasm and the cytoplasm. Within the nucleus there is irregularly distributed chromatin, and a nucleolus is often visible.
Structural changes
Large amounts of monoparticulate glycogen are seen in some adult hepatocyte nuclei in diabetes mellitus and in insulin resistance, and also in children. In type B hepatitis, core virus particles are seen (Fig. 17.6). Intranuclear virions are also seen in infections due to cytomegalovirus, herpesvirus, echovirus and adenovirus.
Mitochondria
Mitochondria (M) are the sites of oxidative enzyme activity, and are involved in the metabolism of amino acids, lipids and carbohydrates. There are an average of 2200 mitochondria within the hepatocyte.22 A smooth outer limiting membrane and an inner membrane with deep infoldings, the cristae, give the mitochondria a characteristic appearance. The inner membrane surrounds the mitochondrial matrix which contains many dense granules.
Structural changes
Cristae of atypical shape, crystalline inclusions and enlarged or unusually scanty granules are found in a wide variety of conditions, and sometimes also in normal liver. Giant mitochondria are seen most often in alcoholic liver disease23 but are also found in non-alcoholic fatty liver disease24 and other conditions.25 Immunohistochemistry and immunoelectron microscopy can assist in their detection.26 They are frequently found in patients with systemic sclerosis.27 In the early stages of Wilson’s disease mitochondria show variation in shape, increased electron density, widening of the spaces between membranes, vacuolation, enlargement of matrix granules and deposition of crystalline material28,29 (Fig. 17.7). Three types of Wilsonian mitochondria are described which show intrafamilial concordance.30 Abnormal, swollen and irregular mitochondria are found in hepatocytes in Reye’s syndrome31 and other microvesicular fat syndromes.32
Endoplasmic reticulum
Structural changes
Dilatation, degranulation, vesiculation and proliferation of the endoplasmic reticulum can be seen in many conditions. Some of these ‘changes’ are also influenced by the fixation procedure, making them difficult to evaluate. Their accurate quantification requires carefully controlled processing conditions and morphometric analysis. However, in α1-antitrypsin deficiency the dilatation of endoplasmic reticulum is striking, and finely granular material accumulates in the cisternae (Fig. 17.8). In chronic type B hepatitis the cisternae are also dilated and contain tubular structures representing the surface material of the hepatitis B virus (Fig. 17.6), and sometimes complete Dane particles.
Lysosomes
Lysosomes (Ly) are organelles that carry many different lytic enzymes and are involved in the breakdown of proteins, carbohydrates and lipids. Primary lysosomes are small vesicles containing enzymes, but not yet involved in catabolic processes. Secondary lysosomes are membrane-bound, often irregularly shaped electron-dense bodies in which the breakdown processes take place. When undigested residues accumulate and enzyme activity is diminished, the secondary lysosomes are called residual bodies. These are the lipofuscin granules. All types of secondary lysosomes tend to be concentrated around the bile canaliculi.
Structural changes
Lysosomes accumulate iron pigment in various forms of iron overload, including hereditary haemochromatosis.33 They can be strikingly enlarged in inborn errors of metabolism, such as Niemann–Pick disease (Fig. 17.1) and type II glycogenosis, or show characteristic changes, such as in the Dubin–Johnson syndrome (Fig. 17.2). Lamellar and reticular inclusions are seen within them in acquired, drug-related phospholipidosis.16–20
Peroxisomes
Structural changes
Peroxisomes are absent in Zellweger’s syndrome (cerebro-hepato-renal syndrome).34 In alcoholic and drug hepatitis, catalase content of peroxisomes is decreased33 and they show irregular shapes.34–36 Increased numbers of peroxisomes are seen in alcoholic and drug hepatitis35 as well as in cirrhosis.37
Cell sap (cytosol)
Structural changes
Ferritin particles accumulate in iron storage disorders.33 Fat droplets are numerous in fatty liver, but the amount of fat varies greatly with the patient’s state of nutrition. Core particles of hepatitis B virus can be identified in the cytoplasm in many cases of chronic type B hepatitis. Cytoplasmic crystalline inclusions are seen both in normal and in diseased livers. In alcoholic hepatitis, the Mallory bodies found in ballooned hepatocytes are composed of accumulations of cytokeratin and other proteins in the form of filaments (Fig. 17.9).
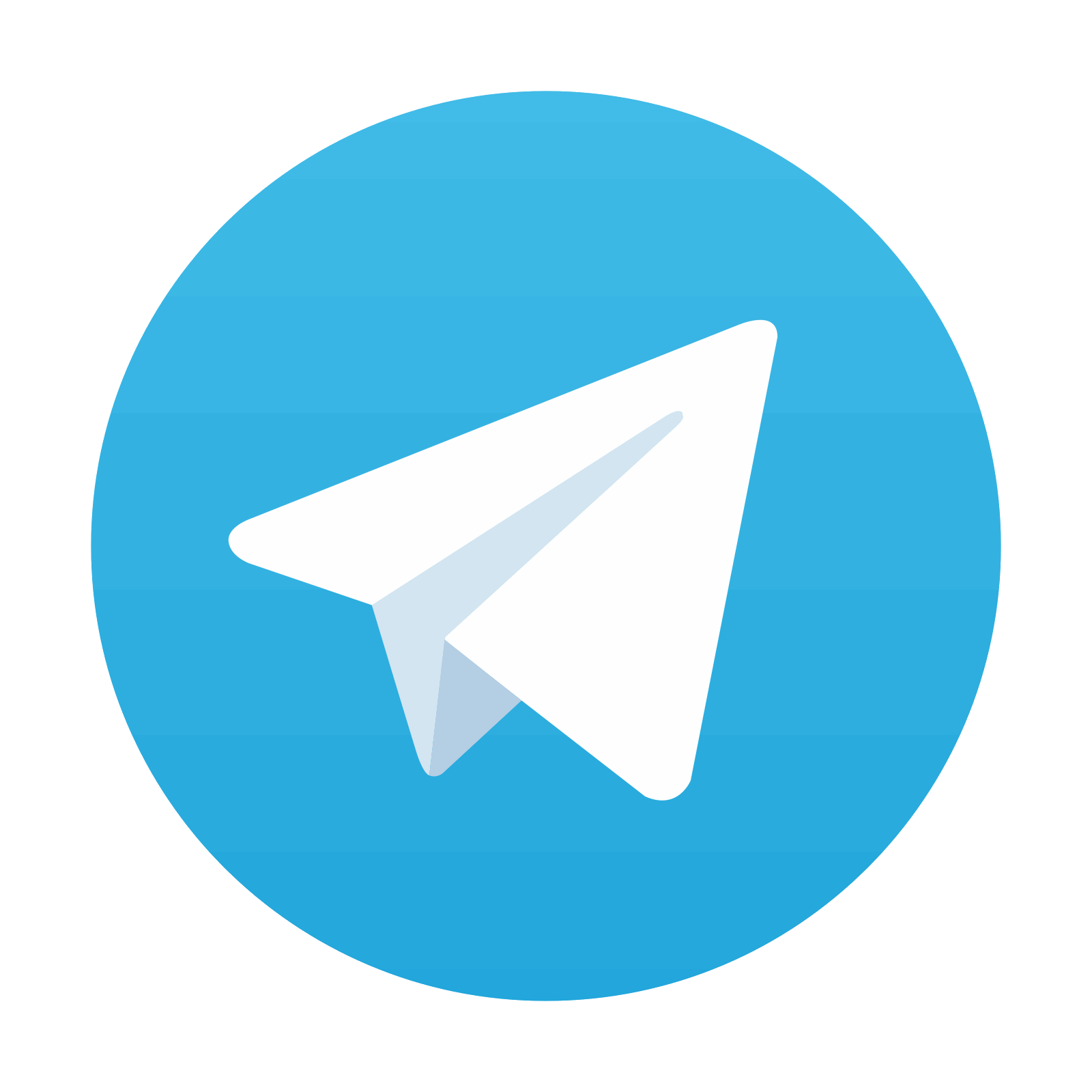
Stay updated, free articles. Join our Telegram channel

Full access? Get Clinical Tree
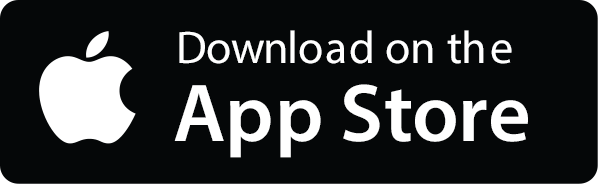
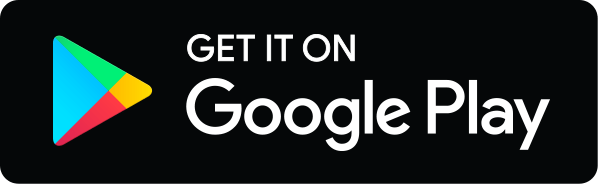
