PB is the main determinant of S and S(d) that both approximate the free drug fraction (1 − PB). The more the drug is bound by protein, the less it can be removed. Acidic drugs typically bind to albumin whereas basic drugs bind to acute phase reactant proteins such as alpha-1-acid glycoprotein. Critically ill patients have a high incidence of hypoalbuminemia and this will especially affect the unbound fraction of drugs with high PB such as ceftriaxone and flucloxacillin. Other factors affecting PB include temperature, pH and the presence of displacing substances such as other drugs, bilirubin or organic acids that are retained in uremia [10]. These changes in PB may at least partially explain the reported differences between a drug’s free fraction (as reported in the literature) and measured sieving coefficients [11, 12] (Table 18.1).
Table 18.1
Factors affecting sieving coefficient and dialysate saturation
S | S(d) | |
---|---|---|
Molecular weight | − | + |
Protein binding | ++++ | ++++ |
Drug–membrane interactions | + | + |
Membrane thickness | − | + |
Membrane pore size | − | + |
Dialysate/blood flow | − | + |
18.2.2 Effluent Flow Rate
The second and most important factor affecting extracorporeal drug removal during CRRT is “dialysis dose” or Q effl. Extracorporeal drug clearance (ClEC) equals the product of S(d) and Q effl [6–9].
Lower antibiotic concentrations have indeed been found in patients treated with high-volume hemofiltration [13–15]. The dose-effect also implies that (frequently occurring) differences between prescribed and delivered CRRT dose should be taken into account.
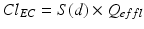
The prefilter infusion of the replacement solution in predilution hemofiltration or hemodiafiltration decreases plasma concentration in the filter and thus also extracorporeal drug removal. The above equation should therefore be adapted with a correction factor where Q b is the blood flow and
is the prefilter replacement rate [6–9].
![$$ C{l}_{EC}=S(d) \times {Q}_{effl} \times \left[{Q}_b/\left({Q}_{s_{pre}}+{Q}_b\right)\right] $$](/wp-content/uploads/2016/07/A313311_1_En_18_Chapter_Equc.gif)

![$$ C{l}_{EC}=S(d) \times {Q}_{effl} \times \left[{Q}_b/\left({Q}_{s_{pre}}+{Q}_b\right)\right] $$](/wp-content/uploads/2016/07/A313311_1_En_18_Chapter_Equc.gif)
18.2.3 Modality and Molecular Weight
Solute transport during hemofiltration is based on convection whereas hemodialysis uses diffusive transport. Convective solute removal is independent of MW up to the cut-off of the membrane (which is much higher than the MW of the majority of the drugs). Diffusion, on the other hand, is dependent on MW and this will potentially lead to a lower extracorporeal removal (compared to hemofiltration) of drugs with relatively high MW such as vancomycin (MW 1,480 Da) [16], teicoplanin (MW 1,880 Da), daptomycin (MW 1,620 Da), bivalirudin (MW 2,180 Da) or colistin (1,160 Da). This is especially the case when achieving equilibrium between blood and dialysate is hampered by the use of low-flux membranes [17], membranes with low surface area [18] and/or high dialysate flow rates [18–20]. Interaction of diffusion and convection in hemodiafiltration may also reduce the clearance of larger solutes compared to pure hemofiltration [21].
18.2.4 Membrane
Theoretically extracorporeal drug removal can also be affected by drug–membrane interactions. These interactions may be the result of drug charge and the so-called Gibbs–Donnan effect where negatively charged proteins along the membrane result in the retention of cationic drugs. Anionic drugs may experience the opposite effect explaining why drug concentrations in the effluent may be higher than the plasma concentration.
Another drug–membrane interaction is drug adsorption to the membrane that depends on drug and membrane characteristics. Drug adsorption has been described for levofloxacin, aminoglycosides and vancomycin with the polyacrylonitrile membrane [22–26]. The phenomenon rapidly reaches saturation and the clinical importance is not clear. It may influence aminoglycoside blood levels after the first dose, but, due to membrane saturation, the next dose will not be affected if the membrane has not been changed. Drug–membrane interactions provide another explanation for the reported differences between a drug’s free fraction and S(d) [11, 12].
18.2.5 Volume of Distribution
RRT only clears the plasma, which means that drugs with large V d are less efficiently cleared by extracorporeal therapy. This explains why intermittent RRT is often followed by a rebound of the plasma concentration due to re-equilibration between central and peripheral compartments. CRRT has access to the total V d due to continuous re-equilibration between the different compartments. For the same daily extracorporeal clearance, CRRT will therefore increase drug removal compared with intermittent hemodialysis. However, due to the limited plasma concentration of drugs with large V d the amount eliminated will anyway be small compared to the total amount present in the body. Here too, changes in V d induced by critical illness and its recovery should be taken into account.
18.3 Is Extracorporeal Drug Removal Clinically Important?
The clinical importance of ClEC is determined by its contribution to total body clearance, which is the sum of all regional clearances including ClEC, residual renal clearance (ClR), hepatic drug metabolism and other non-renal clearances (ClNR). The contribution of a regional clearance to the total body clearance is called a fractional clearance (FrCl) [6–9].
Any regional clearance is only clinically important if its contribution to total body clearance exceeds 25 %. Concomitant hepatic impairment, residual kidney function and the intensity of CRRT may all affect fractional extracorporeal clearance (FrClEC). In addition, the effect of AKI on drug metabolism should also be taken into account [27, 28].
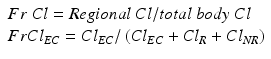
18.4 Drug Dosing Regimens
In many cases drug dosing in critically ill patients will have to compromise between the risk of insufficient efficacy and the risk of toxicity. For instance, the dosing of antibiotics should take into account the severity of the infection, whether the patient’s immune system is compromised, the minimal inhibitory concentration (MIC) of the most resistant (presumed) pathogen, the pharmacodynamics of the antibiotic, its potential toxicity and the changes in pharmacokinetics induced by critical illness.
The loading dose of a drug only depends on the volume of distribution and body weight (BW) and should thus be adapted for the changes in V d induced by critical illness, however, not for CRRT itself.
Different approaches can be used for the selection of a maintenance dose. For antibiotics, the dosing schedule should take into account the pharmacodynamic profile (time-dependent or concentration-dependent bacterial killing). For time-dependent antibiotics, such as the beta-lactams, a continuous or extended infusion may be the optimal method to deliver the maintenance dose [29, 30].

18.4.1 Literature Data
Published dosing guidelines on drug dosing during CRRT are generally based on studies involving small and heterogeneous patient populations treated with heterogeneous (modality, intensity, membrane) CRRT [7, 31, 32]. Their applicability on individual patients is therefore questionable. In addition, many clinical trials in this field poorly report CRRT settings [33, 34] or report pharmacokinetics during CRRT thus reflecting the combined effect of critical illness and CRRT [35]. Understanding the basic principles of solute removal with CRRT (besides the pharmacokinetics and pharmacodynamics of critically ill patients) is therefore of utmost importance [6, 8, 9].
18.4.2 Drugs with Measurable Clinical Effect
Medications with real-time measurable clinical effect such as vasoactive drugs or sedatives can be titrated to their effect and therefore do not represent a problem.
18.4.3 Drugs with Available Monitoring
In most clinical settings therapeutic drug monitoring (TDM) is limited to aminoglycosides, glycopeptides and some anti-epileptics that have a narrow therapeutic index. CRRT can even be used to reduce toxicity of antibiotics that require high peak levels for efficacy such as the aminoglycosides. Reducing toxicity may require an intensified CRRT [36].
Beta-lactam and quinolone antibiotics have a wide safety margin. However, the frequently reported subtherapeutic levels in patients on CRRT [3, 5] that may result in treatment failure and selection of resistant pathogens argue for an expansion of TDM to these classes of antibiotics, not just for minimizing toxicity but mainly for ensuring efficacy [37–39].
Adaptation to the required drug level can use the following formula:


18.4.4 Drug with High Protein Binding
Protein binding is the main determinant of S(d). This explains why the extracorporeal elimination of drugs with high PB can be expected to be limited. However, changes in PB due to critical illness must be taken into account and may explain why drugs with high PB in healthy volunteers such as ceftriaxone may be significantly removed with CRRT [40].
18.4.5 Drugs with Predominant Non-renal Clearance
The concept of fractional extracorporeal clearance explains why extracorporeal removal with CRRT will not be clinically important for drugs with predominant non-renal clearance such as voriconazole [41], amphotericin [42], echinocandins [43] or benzodiazepines [44]. Reduction in hepatic drug clearance induced by the patient’s condition should be accounted for and may increase the clinical importance of extracorporeal clearance. In addition, (eventually active) metabolites may be significantly cleared [45].
18.4.6 Drugs with Predominant Renal Clearance
Drugs with predominant renal clearance generally require dosage adaptation for CRRT. Whether dosage adaptation should include a change in dosing interval or maintenance dose depends on the pharmacodynamics [8, 46–48].
The simplest way to perform this dosage adaptation is to determine extracorporeal creatinine clearance (=Q effl corrected for predilution) and to administer the dose that applies for a patient with comparable renal creatinine clearance [6, 9], eventually taking into account residual renal clearance. The major drawback of this method is the assumption that renal drug clearance is only by glomerular filtration. However, many drugs undergo tubular reabsorption or tubular secretion. Since the extracorporeal system only mimics glomerular filtration and not the tubular function, this may result in underdosing of drugs that undergo tubular reabsorption and overdosing in drugs that undergo tubular secretion, as illustrated in Fig. 18.1.
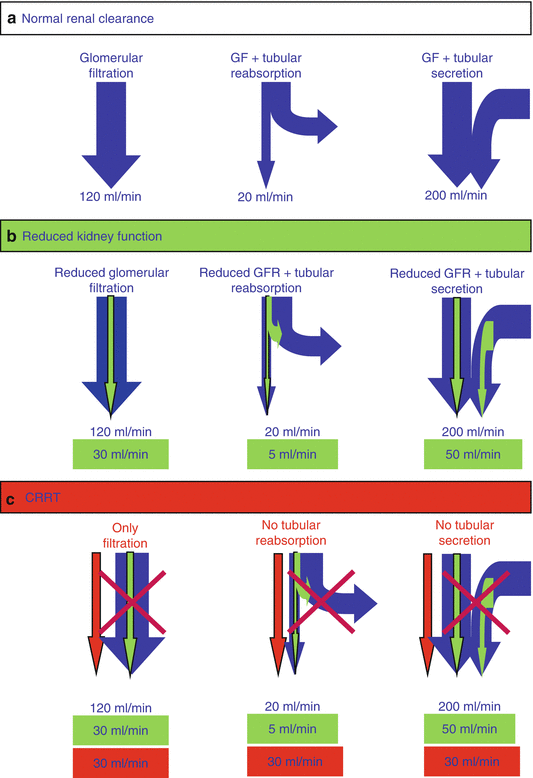
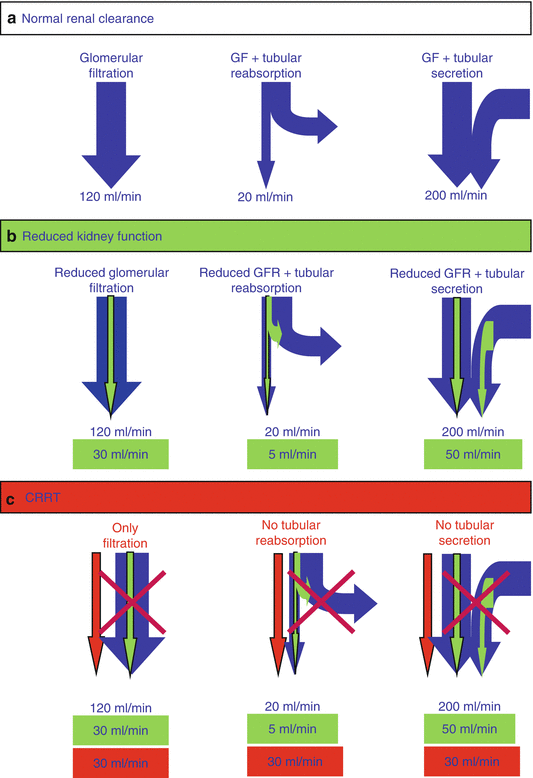
Fig. 18.1
Extracorporeal removal of drugs with tubular secretion or reabsorption. (a) Figure representing renal clearance of a drug undergoing tubular reabsorption or tubular secretion in a patient with normal GFR. (b) With reduced kidney function (and assuming a similar decrease of glomerular and tubular function) clearance of drugs with tubular secretion and reabsorption will be reduced in proportion to the reduction in GFR. (c) CRRT with an extracorporeal clearance that is similar to the renal clearance under b. Due to the absence of tubular function, the clearance of a drug with tubular reabsorption will be less reduced and may be even higher than with normal kidney function. For drugs with tubular secretion, the reduction of drug clearance will be more pronounced compared with reduced kidney function
The best example of this problem is fluconazole that has an almost exclusive renal elimination. In the normal kidney, fluconazole is substantially reabsorbed resulting in a ClR that is much lower than glomerular filtration. During CRRT, this reabsorption does not take place and the ClEC may therefore be even higher than normal ClR in healthy subjects. Supranormal doses of fluconazole will therefore be required in patients on CRRT especially with relatively high Q effl [49–52]. Another antibiotic that undergoes substantial tubular reabsorption is colistin [53].
Another method for dosage adaptation consists of reducing the dose in proportion to the reduction in total body clearance [54].
< div class='tao-gold-member'>
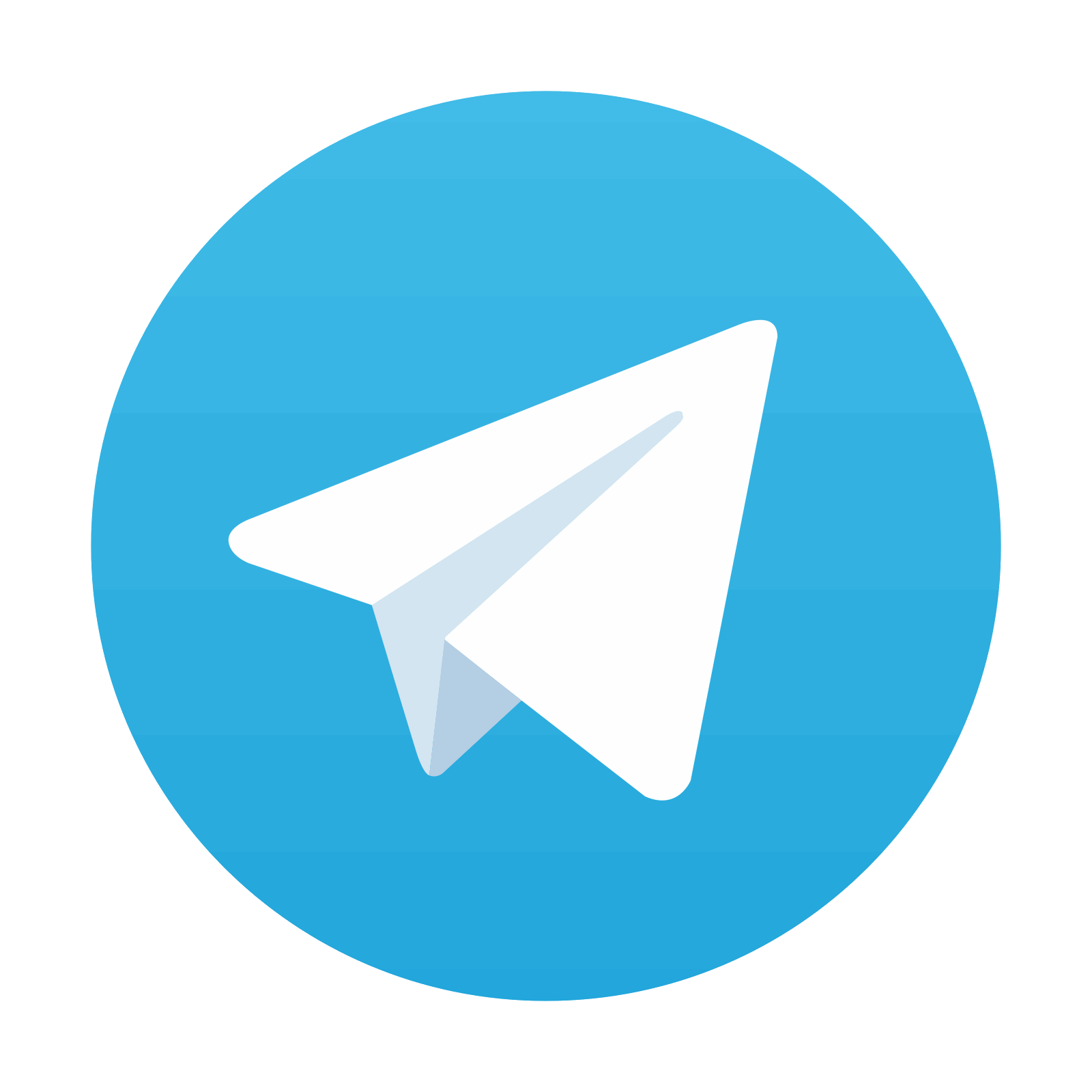

Only gold members can continue reading. Log In or Register a > to continue
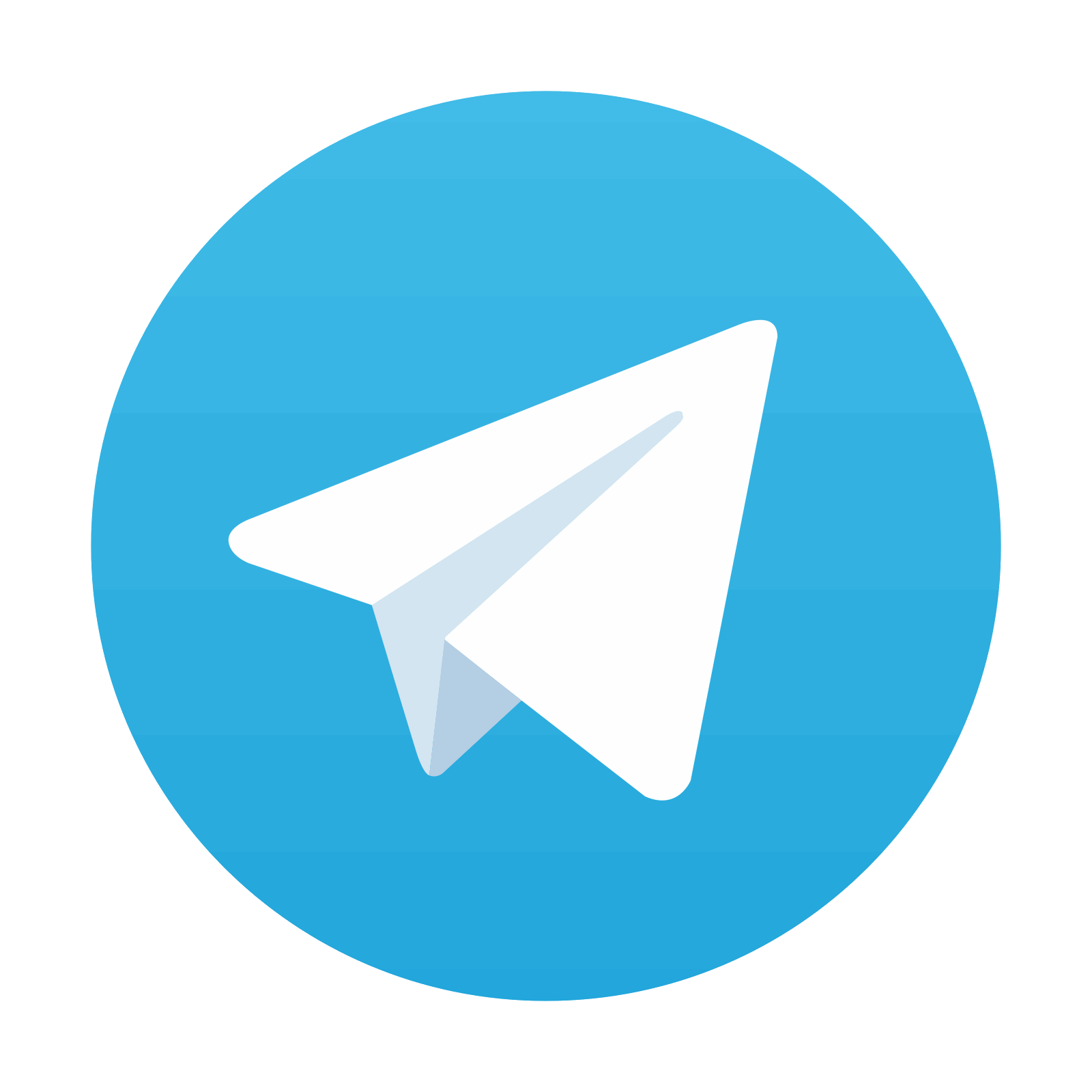
Stay updated, free articles. Join our Telegram channel

Full access? Get Clinical Tree
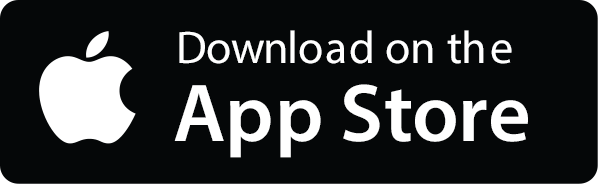
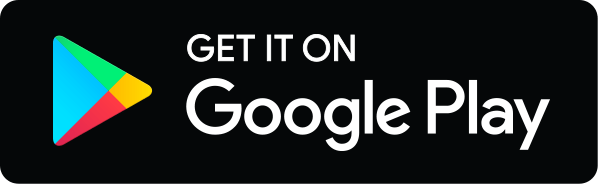
