Fig. 4.1
Illustration of how foods move through the gastrointestinal tract (Figure from OpenStax CNX™ available at: https://courses.lumenlearning.com/ap2/chapter/digestive-system-processes-and-regulation/)
Intestinal Microbiota
The digestive tract is the most heavily colonized organ in humans with the colon alone estimated to contain more than 70 % of all human microbes [9]. The gut microbiome (microbiota, microflora, or flora) coexists with the host and influences the host’s own metabolism, nutritional status, and immune function (Fig. 4.2). Four bacterial phyla are commonly found in humans: Firmicutes (e.g., Streptococcus, Lactobacillus, Clostridia), Bacteroidetes (e.g., Prevotella, Bacteroides), Proteobacteria (e.g., Haemophilus, Escherichia, Salmonella, Oxalobacter), and Actinobacteria (e.g., Propionibacterium, Bifidobacteriales) [11]. For more than 100 years, the existence of gastrointestinal microbes and their beneficial effect on health have been known [7]. The use of kefir by people in the Bulgarian area was attributed to their notably longer life expectancy at the turn of the twentieth century than that of other Europeans [12]. Indeed, a species of lactic acid bacteria – Lactobacillus bulgaricus – owes its name to this observation. The gastrointestinal tract normally contains more than 1,000 known different species of bacteria [11], many of which are pathogenic if allowed to overgrow but many others of which are beneficial and thus known as probiotics. The definition of a probiotic has changed and continues to change over time but is most commonly and basically defined as a live microorganism that confers a health benefit to the host [13, 14] (Table 4.1).


Fig. 4.2
The interactions of nutritional factors and the intestinal microbiome on gut-associated metabolic activities, barrier function and the immune system (Published in: Thomas and Ockhuizen [10])
Table 4.1
Definitions for probiotics and prebiotics
Definition | Examples | Food sources | Examples of proposed effects |
---|---|---|---|
Probiotics | |||
Live, nonpathogenic microorganisms that inhabit the gastrointestinal tract and confer a health benefit on the host when consumed and/or administered in sufficient amounts or when colonized in the gastrointestinal tract in sufficient concentrations and within conditions that optimize their activity | Certain strains of: | Yogurts, kefir and other fermented dairy products, breast milk, cheeses, fermented foods (e.g., soy, vegetables), over-the-counter supplements, supplemented infant formulas, fortified foods | Effects are direct and include: |
Lactobacillus | |||
Prevention/reduction of duration and severity of certain virus- or antibiotic-induced diarrhea | |||
Bifidobacterium | Attenuation of effects of lactose intolerance | ||
Escherichia | Decreased expression and concentration of putrefactive bacterial metabolites | ||
Enterococcus | Alleviation or prevention of irritable or disordered bowel activity | ||
Streptococcus | Prevention of overgrowth of pathogenic bacteria | ||
Bacillus | Prevention of certain infections | ||
Certain yeasts | Treatment of some urogenital infections | ||
Prebiotics | |||
Nondigestible but fermentable dietary components that allow for specific changes in the gastrointestinal microflora that confers benefits upon the host | Inulin | Fruits, vegetables, whole grains, honey, extraction of chicory root, over-the-counter supplements, fortified foods | Effects are indirect, i.e., mediated by intestinal microflora, by way of providing substrate for the colonization of probiotics (see above definition) that produce beneficial metabolites or exert other effects (see above) |
Some oligosaccharides (e.g., fructo-oligosaccharide, galacto-oligosaccharide) | |||
Lactulose | |||
Lactitol | |||
Resveratrol | |||
Gum arabic | |||
Fructan | |||
Some pyrodextrins | |||
Resistant starches |
As bacteria ferment undigested food material passing into the colon, metabolites are created, many of which are increasingly recognized as beneficial to the host [9]. There are two main types of bacterial fermentation in the gut, saccharolytic and proteolytic. Saccharolytic fermentation is carbohydrate based and gives rise to beneficial metabolites such as the short chain fatty acids propionate and acetate [19]. Short chain fatty acids are integral to the health of the colonic epithelium and have a myriad of other benefits, including anti-inflammatory properties [7, 8, 11]. Proteolytic bacterial fermentation is protein based and is a source of a number of toxic metabolites, particularly uremic toxins (e.g., indoxyl sulfate and p-cresyl sulfate) [8, 20].
Bacterial populations differ depending on location in the digestive tract. Bacteria in the mouth are dominated by Streptococcus, which exist in biofilms on the surface of teeth [9]. The stomach, despite its low pH, also has a rich microbiota under normal physiologic conditions. But the highest density of gut microorganisms appears in the lower intestinal tract and is dominated by two bacterial phyla, Bacteroidetes and Firmicutes [11]. The primary gut microbial profile is thought to be fairly stable throughout a person’s life once established, but external factors can dramatically affect it [19], including the intake of substrate for bacteria [17]. Many studies have documented the effect of antibiotics on gut bacteria and their ability to induce dysbiosis [21]. In one study, antibiotic exposure was associated with an enrichment and stabilization of resistant Bacteroides strains [22]. Other studies have documented the role of antibiotics in the overgrowth of pathogenic gut bacteria such as Clostridium difficile [23]. Aging may also affect gut microbiota. The composition of the gut microbiome in people >65 years of age is distinct from that of younger adults [24].
Diet patterns and dietary changes also seem to influence the types of bacteria in the microbiome [19]. In one study, elderly adults living independently in the community had a reduction in the diversity of their gut flora after moving to assisted living, and these differences were associated with dietary changes incurred while in assisted living [25]. Differences in gut microbiome profiles have been observed in lean vs. obese individuals . Mouse studies have shown that obesity is associated with changes in the relative abundance of two dominant bacterial divisions, Bacteroidetes and Firmicutes [26]. Overweight and obese humans have been shown to have a higher relative Firmicutes abundance than lean individuals [27]. High-fat vs. low-fat diets have been shown to associate with distinct differences in gut microbial profiles [28]. High-protein diets are associated with increased levels of uremic toxins, metabolites produced by proteolytic bacteria, such as trimethylamine-N-oxide (TMO) [29], a microbial-generated metabolite of choline and phosphatidylcholine. High TMO blood levels have been linked to increased cardiovascular risk [29, 30]. In a study comparing the gut microbiome of people eating an entirely animal-based diet (high protein) to one that was completely plant based (lower in protein and higher in fiber), those eating the animal-based diet had higher levels of Proteobacteria and fewer Firmicutes than those eating the plant-based diet. Interestingly, the investigators discovered that 1 day on either diet was sufficient to shift the gut microbiome profile [19]. Higher- vs. lower-fiber diets in general are associated with changes in gut microbiota. Studies tend to show a higher proportion of Firmicutes with higher-fiber diets in addition to lower numbers of Lactobacillus [14].
In addition to macronutrients, dietary micronutrients and other plant-derived compounds have been found to influence the microbiome, and these effects are currently an area of heightened interest, if not debate, among biomedical researchers. For example, while a gluten-free diet in patients with celiac disease promoted the growth of Bifidobacterium populations in one study [31], it was associated with lower levels in another [32]. Bifidobacteria are thought to reduce intestinal inflammation, so understanding of their modulation by gluten and/or other plant-derived components is important for many patients with intestinal disorders not limited to celiac. In patients with generalized irritable and/or inflammatory bowel problems, a dietary strategy used with increasing clinical prevalence is a diet low for fermentable oligosaccharides, disaccharides, monosaccharides, and polyols (FODMAPs), touted for its purported ability to reduce symptoms associated with these bowel disorders (e.g., bloating, abdominal pain, diarrhea, constipation) [33]. By default, this diet is frequently low in fiber. But its effect on healthy gut bacteria, such as Bifidobacterium and other species, is controversial. Inulin and fructo-oligosaccharides, which are typically limited in the low FODMAP diet, have been shown to increase Bifidobacterium populations; their restriction may reduce it [34]. But other evidence suggests that long-term restrictive diets, especially those lower for carbohydrates such as low FODMAPs, detrimentally alters gut microbiota and contributes to dysbiosis [35], which could actually worsen symptoms related to irritating bowel symptoms.
Certain gut microbiota is being investigated as a source of systemic inflammation in various disease states, including chronic kidney disease [36]. Patients with kidney disease frequently have a predominance of bacteria that possess urease-, uricase-, indole-, and p-cresol-forming enzymes. The presence of these bacteria can cause increased bacterial metabolism of urease to ammonia [37], which erodes the epithelial barrier. In patients with kidney stones, much attention has been placed on Oxalobacter formigenes (O. formigenes) [38], an obligate oxalate user that resides in the colon. Studies have shown that many people who form kidney stones do not have high levels of this bacterium in their digestive tract [39, 40]. Whether this is diet related has not yet been clarified, but antibiotics appear to have a major effect on O. formigenes [41, 42]. Indeed, reduced recolonization rates after antibiotic therapy are noted [43]. A few other species of intestinal bacteria, including strains of Lactobacillus and Bifidobacterium [44, 45], are also capable of consuming oxalate and have recently been shown to carry the same oxalate-degrading genes as O. formigenes [46]. Dietary patterns that enhance these bacterial species may thus be beneficial for patients who form calcium oxalate stones; studies are needed to confirm this.
Disorders of Digestion and Absorption Relevant to Kidney Stones
Irritable Bowel Syndrome and Inflammatory Bowel Disease
Irritable bowel syndrome (IBS) and inflammatory bowel disease (IBD) are common gastrointestinal tract disorders with symptoms including abdominal discomfort, distension, bloating, diarrhea, and/or constipation [47]. IBS is by definition a diagnosis of exclusion [48]. It is characterized by pain and altered bowel function in the absence of any organic or physiological cause. While the exact cause of IBS is not clear, it is thought to be multifactorial and possibly related, at least in part, to a dysregulation of the brain-gut axis resulting in spasms or inappropriate muscle activity [49]. Dysbiosis is also thought to play a role [50], and dietary triggers that promote dysbiosis are described. IBD is largely an idiopathic disease characterized by chronic, T-cell-mediated inflammation of the digestive tract [51, 52], though a genetic basis has been proposed [53]. Other theories suggest that one contributing factor may be an altered or inappropriate immune response to microflora in the digestive tract [54]. Two common types of IBD are ulcerative colitis, which mainly affects the colon and rectum [55], and Crohn’s disease [56], which may occur anywhere in the digestive tract.
IBS is associated with bacterial overgrowth [57], bile salt malabsorption, fat malabsorption, calcium malabsorption, and lactose malabsorption. Bowel surgery can induce IBS symptoms [58, 59]. Dietary treatments for IBS have typically been diets that exclude or limit fat, sugar (simple carbohydrates), and individual “trigger” foods [51]. New evidence implicates certain types of carbohydrates, specifically fermentable oligo-, di-, and monosaccharides and polyols (FODMAPs). FODMAPs draw water into the digestive tract, which could promote bloating in people prone to IBS. But studies of patients who restrict dietary FODMAPs are inconclusive in showing efficacy; individual variations and responses to treatment appear common [35, 60].
Patients with IBS have a higher risk for urinary stone disease [61, 62]. The fat malabsorption that often accompanies IBS and conditions causing IBS-like symptoms (e.g., celiac sprue, cystic fibrosis) is associated with an increased risk for calcium oxalate stones due to the binding of calcium with fats [63], rendering calcium less available to bind with oxalate and to lower its absorption. Additionally, patients with IBS have been found to have lower levels of Bifidobacterium [64]. As some species of Bifidobacterium are known to degrade oxalate, therapeutic interventions to enhance their presence in the gut are being considered [65].
IBD is associated with increased intestinal permeability which triggers inflammatory responses [47, 52]. With this chronic inflammation, normal gut tissue may be replaced with scar tissue and ultimately cease to function. Bowel resections are not uncommon in patients with IBD [58, 59]. The prevalence of urolithiasis is higher in patients with IBD [63, 66], and this is potentiated as patients undergo multiple bowel resections. Risk factors for renal stone development include malabsorption and dysbiosis, both of which can lead to excessive oxalate absorption and urinary excretion [61]. Additionally, patients with IBD characterized by frequent or chronic diarrhea often experience bicarbonate wasting, and this can lead to lower urinary citrate excretion and higher risk for calcium stone formation and also to uric acid stones if urine pH is low [61, 63].
Short Bowel Syndrome (SBS)
Small intestine resections involving the loss of more than 100 cm can lead to compromised nutritional status due to macronutrient malabsorption (fat, carbohydrate, and protein) and micronutrient malabsorption (vitamins and trace elements) [58, 59]. Problems with diarrhea are common in patients with SBS due to increased gastric motility, unabsorbed bile salts entering the colon, and excess water losses in stool [67]. Treatment for SBS is aimed at controlling diarrhea and fluid losses and repletion of minerals and vitamins [51, 67]. Late complications of SBS include cholelithiasis due to the fact that bile losses exceed the liver’s capacity to synthesize the bile. Small bowel bacterial overgrowth (SBBO) and d-lactic acidosis are also complications with SBS [57]. SBBO results in inflammatory changes in the intestinal mucosa causing fluid losses, fat malabsorption, and subsequent fat-soluble vitamin deficiency. With d-lactic acidosis, there is a malabsorption of carbohydrates (especially refined sugars) [67].
Patients with SBS have an increased risk for calcium oxalate kidney stones due to increased absorption of oxalate from the colon [58, 61]. In the setting of fat malabsorption (steatorrhea), increased intraluminal free fatty acids sequester and bind to calcium, resulting in increased oxalate absorption. Other factors contributing to increased stone risk include higher fecal fluid losses and reduced urine output, lower urinary magnesium (due to lower magnesium absorption) causing reduced capacity to keep oxalate soluble in urine, and bicarbonate wasting from diarrhea causing higher renal citrate reabsorption and lower urine citrate [63]. Acidic urine has also been observed in patients with SBS, and this may promote the precipitation of uric acid in urine.
Gastric Bypass Surgery
Bariatric surgery for obesity is increasing. Malabsorption of macronutrients, essential vitamins, and minerals occurs along with substantial weight loss. An increased incidence of kidney stones related to bariatric surgery has been observed similar to risks listed above for SBS [68–70]. Increased incidence of oxalate nephropathy has also been observed [71, 72]. In patients with bariatric surgery and nephrolithiasis, urinary oxalate was found to be directly related to fat malabsorption and the concomitant reduced availability of free calcium to bind oxalate in the gut [73]. When patients were placed on a low-fat diet after bypass, urinary oxalate levels were lower [74]. Vitamin deficiencies due to malabsorption may include pyridoxine (vitamin B6), a cofactor in hepatic metabolism of glyoxylate [75, 76]. Impaired function of the enzyme participating in this reaction leads to increased oxalate biosynthesis and urinary excretion. Whether and how bariatric surgery affects the gut microbiome are not fully characterized [77]. However, decreased intestinal colonization with oxalate-degrading bacteria in patients after gastric bypass has been described [78].
Diarrhea
Patients with gastrointestinal tract conditions characterized by diarrhea, which may be idiopathic, antibiotic induced, or associated with any of the digestive conditions described above, must compensate for extrarenal fluid losses in order to promote suitably ample urine and maintain lower supersaturation. Low urine volume increases the concentration of urine and thus raises the risk of all types of stone formation. Diarrhea also promotes malabsorption, which increases the risk for bicarbonate wasting [63]. The rapid transit of gut materials associated with diarrhea reduces the time required for bacterial oxalate fermentation and potentially to altered microbial populations that are overall less effective in degrading oxalate. Strategies to control diarrhea are therefore helpful in mitigating the effects of low urine volume, malabsorption, dysbiosis, and bicarbonate wasting [61].
Oxalate Absorption and Transport
Patients with any type of digestive tract disorder are potentially at risk for dysbiosis which may lead to higher oxalate absorption. Patients with gastrointestinal conditions may avoid certain foods, such as fiber or fructo-oligosaccharides. As these food-derived compounds provide substrate for many bacteria, dietary changes that eliminate or reduce these could affect the health of the gut microbiome. For example, a restriction of dietary oxalate may reduce the presence of O. formigenes as its only food source is limited [79]. In other digestive tract disorders, non-functioning or necrotic gut tissue could alter oxalate absorption and/or transport. For example, some patients with IBD and/or other bowel disorders have increased colonic mucosal permeability due to inflammation and potentially also to autoimmune activities [73]. This is thought to lead to excess free oxalate absorption as some oxalate is absorbed before binding to calcium or magnesium and prior to bacterial degradation. Patients with delayed stomach emptying, such as in gastroparesis, may also be at risk for enteric hyperoxaluria. The stomach is a site of oxalate uptake [80]; transport is presumed to be via a transcellular mechanism. Increased oxalate absorption is observed when transit time through the stomach is slow.
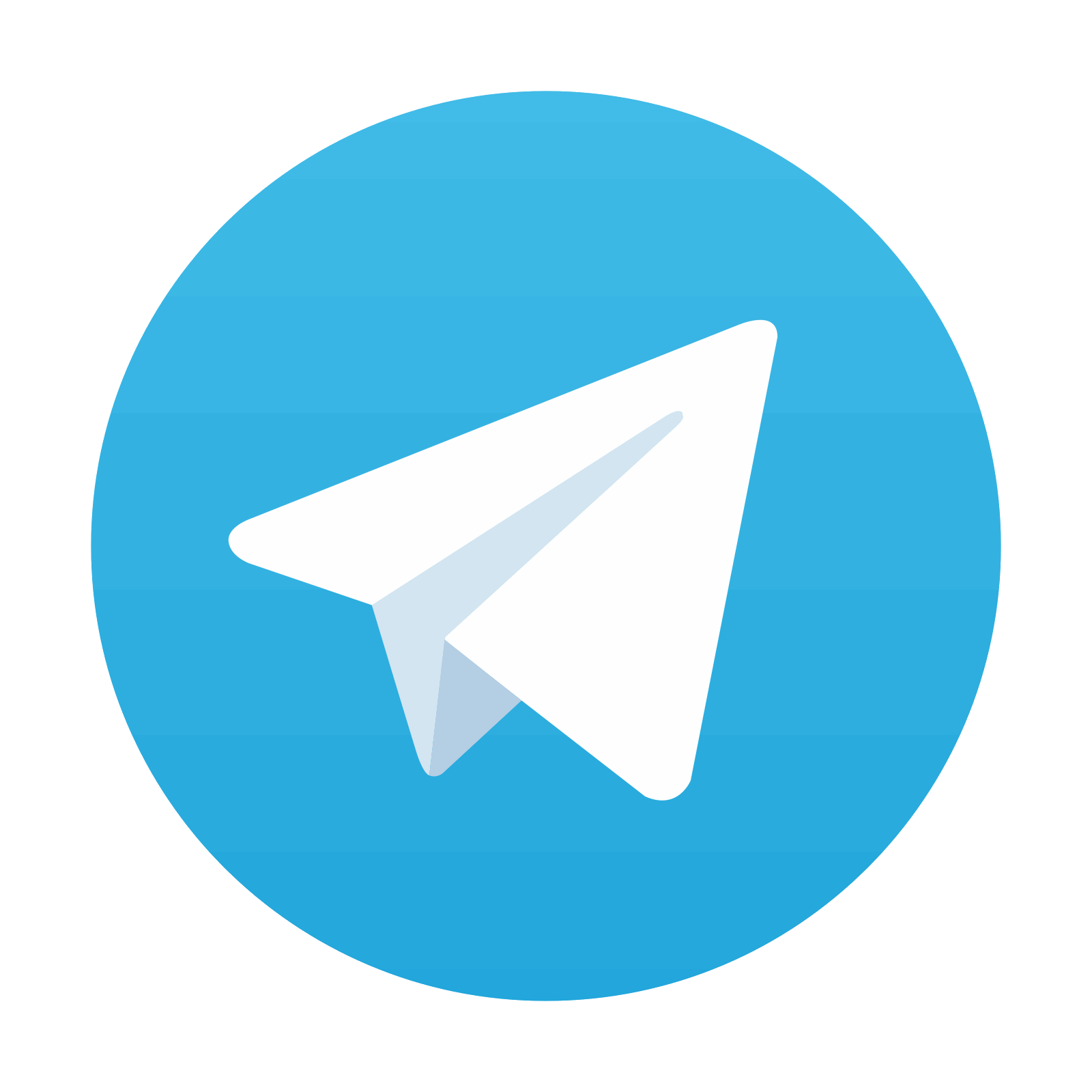
Stay updated, free articles. Join our Telegram channel

Full access? Get Clinical Tree
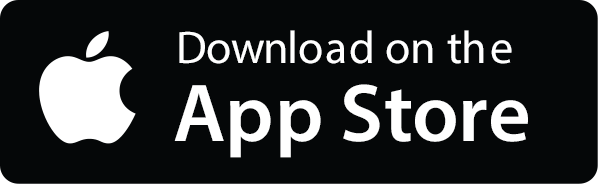
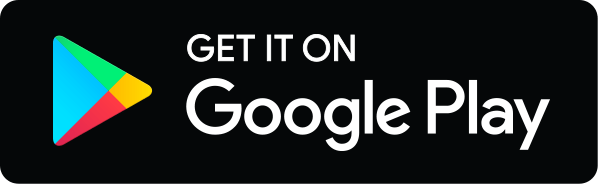