Abstract
The gut microbial community acts as a vector for mediating effects of diet on host physiology. Recent advances in the next-generation sequencing and mass spectrometry along with translatable animal models have paved the way for dissecting the effects of gut microbiota in mediating the effect of diet on host physiology. The major macromolecular components of our diets, which includes fats, carbohydrates (with varying levels of host/microbe accessibility), and proteins can elicit microbial community remodeling, and serve as substrates for the generation of molecules by microbes that affect host physiology. These molecules can be either beneficial or undesirable for the host and play an important role in health as well as causing disease. In addition to composition of diet, the timing of consumption can have significant effects on microbiome structure and function and may predispose individuals to microbiota mediated diseases. Further work analyzing these complex interactions will spawn new, individual specific therapies, which target microbes in the gut for a variety of host conditions.
Keywords
Diet, Microbiota, Fat, Protein, Carbohydrates, Short-chain fatty acid, Bile acid, Obesity, GI motility
Abbreviations
DIO
diet-induced obesity
FFA
free fatty acid
FOS
fructo-oligosaccharides
GOS
galacto-oligosaccharides
HFD
high fat diet
HMO
human milk oligosaccharides
LPS
lipopolysaccharide
PBA
primary bile acid
RYGB
Roux-en-Y gastric bypass
SBA
secondary bile acid
SCFA
short-chain fatty acid
WD
western diet
33.1
Introduction
Diet has long been implicated in a suite of specific physiological outcomes in the host, ranging from obesity, to cancer, to risk of infectious disease. The advent of readily accessible next-generation sequencing (NGS) platforms in the last 15 years has revealed that diet can affect the composition and functionality of microbes in the gut. Targeted mechanistic studies of these changes have revealed that many of the well-described effects of diet on host physiology have substantial microbial underpinning, suggesting that the gut microbiota—the collection of microbes in the gut—is a vector for diet to exert physiological changes in the host.
Diet is considered as one of the three major effectors of host microbial structure, which along side host genetics, and early life exposures, are responsible for the assembly and subsequent composition of our gut microbiota. Factors like our method of delivery, whether we are breast fed or formula fed, and when we were first exposed to antibiotics interact with host-genetic pressures to set the foundation for what will ultimately become the homeostatic microbial associations that we maintain for the duration of our lives. However, diet has the capability to modulate that foundation and has been shown to trump host genetics with respect to phenotypic outcomes. The diet plays a critical role in regulating the relative abundance and activity of the bevy of microbes that live in and on us. Diet-induced microbiome changes occur rapidly, in as few as 4 days, and the effects are readily reversible in the short term. However, long-term effects of diet on microbial community structure have also been noted. Cultural dietary norms tune baseline microbial composition to fit the dietary preferences of populations and given sufficient generational exposure, diet can lead to irreversible microbial extinction events. Extinctions are not only difficult to rectify, but the reduction in microbial diversity due to long-term dietary pressure can have negative long-term outcomes for the host. As such, we must pay careful attention to our diets and their resulting effect on microbes in the gut. To begin to understand the effect of diet on the gut microbes and host physiology we must first explore the tools that are available to investigate these systems.
33.2
Techniques to Assess Effects of Diet on Microbial Composition and Functionality
33.2.1
Compositional Tools—Culture Based
Early studies on dissecting the microbiota were compositional or survey experiments. The so-called “who’s there” studies. Forays into this field were once technologically limited to culture-based techniques, which relied on an array of specialized growth mediums in varying conditions to identify the specific microbes. Expensive and time consuming, this paradigm is also restricted to a small subset of organisms for which established culture conditions have been described. This limited our insight to < 20% of what is present in most environments. As a result, locations with limited diversity and those enriched in difficult or impossible to culture resident organisms were considered sterile. The urinary tract is a prime example. Once considered sterile outside of infection, we now appreciate the urinary tract has a complex and dynamic microbial association. Nearly all locations in the body now have described resident microbes, and there is great diversity in composition and function based on location. This revelation is the direct result of the development of culture-independent sequence-based identification methodologies, which greatly enhance the extent by which we can probe composition.
33.2.2
Compositional Tools—Sequence Based
Initial sequenced-based compositional analyses of gut microbiota took advantage of the variable conservation of DNA sequence in the 16S ribosomal RNA subunit found in all microbes. Regions of conserved sequence flanking highly variable segments are used as targets for PCR-based amplification of sections of 16S subunit from pools of DNA extracted from the entire microbial community. In early investigations, the overall composition of the resulting pool of DNA was examined using denaturing gradient gel electrophoresis to separate different sized bands. Different bands represented distinct taxonomic groups and assessments about diversity within a given population—known as α diversity—could be made by evaluating the number and diversity of bands. Major advances were made with the incorporation of massive parallel sequencing on the PCR products using NGS technologies (e.g., Illumina, 454, Ion Torrent, SOLiD, etc.). These tools generate gigabases of sequence information and downstream bioinformatic pipelines can identify individual 16S sequences from the overall pool by comparing the sequence data to well established and curated databases. We can now assess intrasample (α) and intersample (β) diversity with speed and accuracy.
The extent to which we can probe the entirety of the community, referred to as depth, is limited by the amount and quality of sequence information that can be generated from a given sample. Recent advances in sequencing technology with increased depth and read length have created an alternative approach known as whole metagenomic shotgun (WMS) sequencing. In WMS, all the available genomic DNA in a sample is sequenced using massive parallel techniques and then identified using established microbial genomic databases. The additional information provided by the broad targeting allows for more precise identification and enumeration of specific members in a population. It is particularly valuable for differentiating microbes that have near-identical 16S sequences and can provide insight into the functional genetic capacity of its members, a clear advantage over strictly marker gene (16S)-based approaches. However, the complete genomic databases are not as well established as those for 16S and it is generally more time consuming and expensive than other techniques. In addition, new bioinformatic tools such as PICRUSt have been developed that create in silico predictions of community functionality based on 16S compositional information, leveling the playing field in terms of capabilities across platforms. Both technologies are subject to sequencing biases, so a researcher’s choice of techniques is often determined by specific experimental goals, availability, and cost.
Cutting-edge third-generation sequencing technologies that are beginning to emerge will define the next shift in microbiome tools for assessing community structure. Termed single-molecule real-time (SMRT) sequencing, these technologies have the potential to generate read lengths (continuous sequence from a single piece of DNA) of 10 kilobases and beyond—more than 100 times that is possible with the next-generation platforms used for 16S or WMS sequencing. The increased read length solves many of the limitations of NGS including the challenges of resolving heterologous DNA regions from closely related organisms.
The data generated using NGS is readily distilled using a variety of open source bioinformatic pipelines. These tools allow researchers to assess community composition and diversity within or between samples at varying levels of stringency. Once highly specialized, these bioinformatic pipelines are now commonplace in the research community, frequently as institutional core facilities. Microbial community analysis is more accessible than ever to researchers and is being rapidly integrated into broad areas of biomedical research. However, pure compositional data is limited in its ability to provide insight into host-microbe interactions. Moving beyond “who’s there” to “what are they doing” using functional analyses is the next advancement in the field.
33.2.3
Functional Tools
The rapid advances in compositional analysis tools has generated large data sets attempting to identify specific microbes that are associated with pathological presentations, particularly in the GI tract. However, the gut microbiome is highly variable, even in healthy populations. This makes it difficult, if not impossible to identify specific microbes as diagnostic or causal of observed pathologies based solely on compositional association. A potential explanation for the heterogeneity in human populations is the concept of functional redundancy. Absolute community composition may be irrelevant from the perspective of the host, provided that a core functionality is satisfied. In layman’s terms, this is the “more than one way to skin a cat” theory and could account for the vast diversity of gut microbiome structures in human populations. It suggests that community functionality may be what is most stringently conserved between individuals. Compositional data struggles to provide insight into functional capacity, so additional omics tools have been developed to assess the community functionality at the DNA, RNA, protein, and metabolic levels.
The WMS sequencing as described previously, illustrates one such approach where broad coding potential of a community can be assessed. However, DNA-based surveys merely predict potential functionality, not specific to activity under a given set of conditions. Transcriptomic approaches like RNAseq go a step beyond, and provide a snap shot of gene expression profiles of microbial communities under a given condition. These approaches use massive parallel sequencing on microbial mRNA isolated from samples to answer the “what are they doing” question. Outputs from RNAseq can be compared with established databases to reveal gene expression profiles for individual organisms and also serve as inputs for pathway analysis tools like HuMANN2 to show differential expression in broad metabolic pathways.
After defining “who’s there?” and “what are they doing?” the last level to probe is “what is the result?” Proteomic and metabolomic survey techniques define the suite of functional machinery and their resulting effects on the overall metabolic state of complex communities. They describe the metabolic equilibrium in a sample at the time of assessment. Most proteomic and metabolomic approaches use nuclear magnetic resonance (NMR) or chromatography (liquid or gas) paired with mass spectrometry to identify the proteins or metabolites in communities. Both targeted (searching for specific molecules) or untargeted (all detectable molecules) approaches have been developed and can provide insight into the metabolic milieu. Typically, a combination of all the three approaches (compositional, transcriptomic, and metabolomic) is most effective to generate a complete perspective of a microbial community and its potential effects on host pathology. However, while the omic techniques can help generate hypothesis, to take full advantage of the power of the omic technology, appropriate in vivo models are needed.
33.2.4
Modeling Dietary Effects in Animal Models
Obviously, the most relevant system to study the dietary effects on host pathology is humans. However, like all human studies, there are notable limitations on what can be done from an observational/interventional perspective. With respect to the microbiota, an additional complication is the broad heterogeneity in both healthy and diseased populations. It is difficult if not impossible to assign discrete microbial criteria that are associated with specific physiological outcomes in human studies simply due the shear variability from a genetic, environmental, and dietary perspective. As a result, animal models have gained traction as robust systems with reduced variability and greater control to begin to dissect diet-related effects.
Initial studies of host-microbiome interactions focused on the normal microbial communities associated with rodent hosts. However, the rodent microbiome is compositionally distinct from human microbiome and frequently requires significant perturbation/modification to mimic human phenotypes. While these models provide conceptual knowledge, they are limited in their translatability to human populations. The need for more readily translatable models has driven the incorporation of germ-free (GF) and gnotobiotic (previously GF mice with defined microbial associations) animal models into dietary studies. The GF animals are born, reared, and subsequently maintained in sterile environments. These animals are completely devoid of any microbial association until the investigator initiates a deliberate exposure. To maintain their sterility, they are housed in sealed isolators, fed sterile food and water, and supplied filtered air. By stringently adhering to these specialized husbandry techniques, sterility can be readily maintained for the lifetime of the animal. The GF mouse is essentially a blank slate for modeling any microbial association the investigator chooses, from individual microbes (mono-associations) to complete complex communities from mice or other species. In the perspective of translatability, the most relevant community to model is humans, and mice colonized with microbes of human origin are often referred to as “humanized.” Humanized mice faithfully recapitulate the structure and function of human communities and represent a readily translatable preclinical model for studying the effects of diet on host physiology that are mediated by the microbiome.
An additional advantage of the mouse model is the precision by which dietary inputs can be controlled. Minimizing confounding variables is challenging when designing diets and careful consideration should be taken with respect to their composition. At a minimum comparable diet formulations should be isolcaloric. The source of nutrients in diets should also be carefully controlled. Standard laboratory rodent chows comprise complex carbohydrate, protein, and fat sources for which precise molecular compositions are not always known. When studying the effects of specific dietary substrates on host outcomes, it is critical that the precise composition of diets be understood. Defined diets, like defined microbiological media, are composed of specific known components—carbohydrates, amino acids, fats, vitamin, and minerals—and should be employed whenever examining effects of specific dietary inputs. Generally, defined diets pose logistical challenges in GF/gnotobiotic applications as autoclave procedures used to sterilize the diets can lead to nutrient degradation. Alternative sterilization techniques are available, but come with increased contamination risks. Despite the technical complications, the level of precision and control provided by defined diets warrants the additional steps needed for their use in GF and gnotobiotic applications. The combination of advanced omics techniques, representative model systems, and custom diets define the tools that can be implemented to dissect diet-microbe-host interactions in the gut.
33.3
Dietary Inputs and Host/Microbiome Effects
Using the compositional and functional tools in the relevant in vivo systems described above, investigators have begun to dissect the role of microbes in mediating diet-induced effects on host pathology. Most studies have focused on particular dietary metabolites, with specific emphasis on major macromolecular groups: fats, carbohydrates—both host accessible (simple sugars) and inaccessible (resistant starch/dietary fiber, etc.), and proteins ( Fig. 33.1 ). These groups are described in detail below as we highlight the impact of these different groups on our physiology.

33.3.1
Fat and Host Accessible Carbohydrates
The obesity epidemic occurring in the western world has garnered intense research attention. The high levels of fat and simple sugar in western diets (WDs) are a major purported cause of diet-induced obesity (DIO). However, the energy imbalance from increased caloric density in WDs cannot solely explain the increased rates of DIO. In the search for additional mediators, microbiome constituents have been implicated as a potential factor. Investigations into the effects of high-fat diet (HFD) and WD (high fat and high host accessible simple sugars) on murine gut microbiome have shown substantial shifts in community structure as a result of dietary intervention. Specifically, increased abundance of Firmicutes and Proteobacteria with a commensurate decrease in the Bacteriodes has been observed and similar trends have been noted in children from European (WD) and African (traditional high fiber, low-fat diet) locations. These changes are rapid, with community remodeling effects of WD being observed within hours to days.
The resulting dysbiosis or alteration in community structure to a state distinct from healthy controls is predicted to be a major cause of DIO. Investigations into the underlying mechanisms have begun to show the specific roles that microbes have on energy balance in the host. Conventional mice (mice with a normal murine microbiome) and humanized mice (previously GF mice that were give a human microbiome) are susceptible to DIO. However, strictly GF mice are resistant to developing obesity when fed WD or HFD. The HFD fed GF animals show elevated levels of AMP activated protein kinase (AMPK)—an upstream regulator of fatty-acid metabolism, and fasting-induced adipose factor (FIAF). Upregulation of these genes leads to increased metabolism of fatty acids and decreased storage of liver derived triglycerides. Interestingly, after being exposed to microbes, previously GF animals display reduced AMPK and FIAF levels and subsequently go on to develop DIO. Further implicating the role of microbes in DIO is the observation that the obesity phenotype is transferrable by transplanting obesity-associated microbiome. When microbial communities from either genetically obese or DIO animals are transferred to naïve GF mice, the recipients become obese despite eating normal caloric loads. Together these data suggests that microbes play a significant role in regulating host energy metabolism/balance however the specific microbial mechanisms that drive this host response have yet to be determined.
In addition to obesity, other physiological effects have been noted as a result of WD/HFD. A recent study by Reichardt and others observed that conventional C57BL/6 mice fed a WD for 6–12 weeks developed dysbiotic gut microbiome and display elevated levels of plasma free fatty acids (FFA) and fecal lipopolysaccharides (LPS). The FAA and LPS are readily produced by the Firmicutes and Proteobacteria that are enriched in WD-induced dysbiotic states. The FAAs and LPS synergistically drive induction of TLR4 mediated degeneration of nitrergic myenteric neurons in the proximal colon leading to delayed colonic transit and constipation. Similar effects to community structure and delay in colonic transit have been described in mice fed HFD alone and obese mice also show increased intestinal permeability and decreased chloride secretion that are linked to PPAR-γ and GLP-2 specific host pathways. It is predicted that the increase in intestinal permeability allows for microbial generated LPS/FAA to translocate across the epithelium and drives stimulation of pro-inflammatory and neurodegenerative pathways. The HFD has also been implicated in a TLR4 mediated increase in visceral hypersensitivity, and studies in IBS models have demonstrated a microbial link in visceral pain perception and gut microbiome. Although a definitive link in HFD specific microbiome alterations and visceral hypersensitivity remains to be made, it stands to reason that the two are connected.
The dramatic rise in obesity rates has lead to a commensurate increase in surgical interventions for obese individuals who fail to lose weight using traditional dietary and exercise-based approaches. The most common bariatric weight loss procedure is the Roux-en-Y gastric bypass (RYGB), which is considered the gold standard surgical intervention. The procedure results in a decrease in food intake, alterations in food preferences, and most patients experience weight loss of up to 65% of their starting weight. While the resulting changes in eating habits frequently result in decreased caloric intake, much like the onset of DIO, caloric balance alone cannot account for the rate or maintenance of weight loss. Unsurprisingly, microbial mediators have been implicated that may account for this discrepancy. In recent work from Liou et al., it was demonstrated that RYGB performed on mice elicits a microbiome remodeling, which enriches for the Gammaproteobacteria and Verrucomicrobia in a weight change and caloric restriction independent fashion. Furthermore, delivery of the RYGB remodeled microbial communities into naïve GF mice caused the recipient mice to lose weight. Together these data further emphasize the importance of microbial community composition with respect to energy balance and show that the effects can be bidirectionally manipulated.
Another consequence of WD/HFD mediated alterations in the microbiome is the enrichment of bacterial species that can readily generate carcinogenic compounds. The WD/HFD enriches for Clostridia spp., which are capable of dehydroxylating primary bile acids (PBAs) to secondary bile acids (SBAs). The SBAs are produced in high levels under these conditions, as the host also increases production of PBAs to facilitate digestion of the high-fat content in the diet. The resulting microbe produced SBAs are implicated in physiological functions such as facilitating nutrient absorption, regulating metabolism, and providing protection from opportunistic gastrointestinal pathogens like Clostridium difficile. However, high concentrations of SBAs in the colon and hepatic circulation have potential carcinogenic effects and the dietary microbiome remodeling that results from HFD/WD has been implicated as a key risk factor for colon and liver cancer.
33.3.2
Host Inaccessible Carbohydrates
The carbohydrates are a major component of human diets but not all carbohydrates are created equal. It is important to note the distinction in availability for carbohydrates from the perspective of the host and microbes. Mono- and disaccharides, like those described above as major components of WD, are readily absorbed in the small intestine. Some of these molecules make it to the colon where the majority of gut bacteria reside. Host-inaccessible carbohydrates, also referred to as resistant, or nondigestible, cannot be metabolized by the host in the absence of further metabolic breakdown from microbes and thus serve as major energetic inputs for the bacteria in the gut.
Resistant starches, which include plant-based starches like cellulose and hemicellulose, are one such example. Humans lack the enzymatic machinery to break down these molecules, making them prime targets for microbial fermentation. Resistant starches are primarily broken down by the Bacteroides , Ruminococcus , Roseburia , and Prevotella into short-chain fatty acids (SCFAs) including butyrate, propionate, and acetate. Commensurately, the consumption of resistant starches enriches for the presence of these microbes. The SCFAs are generally beneficial for the host via a number of described pathways. A major role is as metabolic inputs in locations throughout the body. Butyrate is readily metabolized by colonic epithelial cells, propionate functions in liver gluconeogenesis, and acetate is oxidized in the heart and brain. The SCFAs have also been demonstrated to regulate GI motility and drive host serotonin (5-HT) release, regulate innate immune cell function/migration, increase expression of epithelial tight junction proteins, and have antiinflammatory properties. The broad reaching effects of SCFA make them an attractive candidate for interventions designed to increase abundance of resistant starch fermenting bacteria to treat a number of different host conditions. However, as the effects of SCFAs are not specific and are dose dependent, off target effects should be considered in any therapy designed to increase the abundance and activity of SCFA producing microbes.
Oligosaccharides originating from a variety of sources are also indigestible by the host and can have broad effects on microbiome composition and host physiology. Fructo-oligosaccharide (FOS) is a plant carbohydrate storage molecule composed of 1–7 d -fructose subunits linked to a terminal d -glucose molecule. It is found in high abundance in bananas, onions, garlic, asparagus, and artichokes. The FOS is a commonly used prebiotic (a selectively fermentable molecule that changes the composition or function of a microbial community) for its ability to enrich for Bifidobacteria , in some communities. Bifidobacteria is considered to be beneficial to the host for its ability to lower pH via production of SCFAs, improve epithelial barrier functionality, regulate both innate and adaptive immune response pathways, competitively exclude pathogens, and produce antimicrobial peptides. However, the effects of FOS on microbial community structure and resulting metabolite production have been shown to be variable on an individual community basis and many of the purported beneficial effects of FOS have yet to be scientifically confirmed. This may account for the mixed response to FOS as a prebiotic for some individuals. Oligosaccharides derived from human milk (human milk oligosaccharides—HMOs) have also been evaluated as prebiotics. However, difficulties in manufacturing HMOs in scale have driven focus to the functionally related and easily produced galacto- oligosaccharides (GOS). The HMO/GOS enriches for Bifidobacteria as well as Lactobacillus in children and individuals fed formula supplemented with GOS show microbial communities that are reminiscent of breast-fed children. Breast-fed individuals have reduced rates of diarrhea, necrotizing enterocolitis, and allergic and autoimmune diseases, which are thought to have microbial underpinnings. This suggests that GOS prebiotic supplementation in infants may be an effective strategy in improving long-term health outcomes.
Oligosaccharides like FOS/GOS are structurally similar to another class of carbohydrate molecules called inulins. Despite the terms being used interchangeably in the literature, these are distinct molecules with the primary difference being polymer length. Inulins comprise 10–60 subunits compared to the 2–10 found in the oligosaccharides. Inulins are also non-digestible by the host, primarily being broken down by microbes in the colon. Supplementation with dietary inulins also has a Bifidobacteria enriching effect on microbial communities and inulins have also been shown to enrich for Faecalibacterium prausnitzii. Human studies suggest that inulin supplementation can increase stool frequency and bulk which point to its potential use as a prebiotic to treat some forms of constipation. Further supporting the role of inulins as relevant prebiotics, Liu et al. examined the effects of inulin on microbiome and GI physiology in HFD-induced obese mice. The HFD animals that were transitioned onto custom HFDs supplemented with inulin showed shifts in microbial community structure. They have increased abundances of the mucin degrading bacteria Akkermansia and showed improved epithelial layer architecture and integrity. The microbial mediated mechanisms underlying the physiological changes associated with FOS, GOS, and inulin supplementation remain to be defined despite their association with beneficial host outcomes. As we move to the next phase of investigations, the focus will be on microbial community functionality and its effect on host physiology rather than an attempt to enrich individual members within a community.
33.3.3
Protein
Proteins are a major component of WDs, nearly as abundant as carbohydrates. Host degradation of proteins is an enzymatic process mediated primarily by gastric enzymes and occurs in the stomach and small intestine. Not all of the resulting short peptides and free amino acids are absorbed in these locations and the excess fractions become available to microbes in the colon. In addition to protein from dietary sources, shed epithelial cells, mucus, and pancreatic secretions contain substantial host-derived proteins, which are also microbe accessible. The Bacteroides and Clostridia are particularly well suited to fermenting proteins and free amino acids using both cell surface associated and secreted proteases and protein in the diet provides a selective advantage for these protein-fermenting specialists. The resulting microbial mediated breakdown of amino acids in the gut generates a variety of secondary metabolites with a range of host effects. Metabolism of tryptophan by microbes generates indole derivatives including indole acetic acid (IAA) and indole-3-propionic acid (IPA). These molecules act as ligands for the aryl hydrocarbon receptor, are protective against colitis, and involved in reducing inflammation in the central nervous system. Microbes are also capable of decarboxylating dietary tryptophan to tryptamine, a β-arylamine neurotransmitter, which induces ion secretion in gut epithelial cells. Host serotonin (5-HT) is also generated from dietary tryptophan so competition for tryptophan by microbes in the gut may alter overall serotonin dynamics in the host. Both ionic secretion and serotonin production in the gut affect gastrointestinal transit time.
A variety of toxic metabolites including ammonia, amines, N -nitroso compounds, phenolic compounds, and sulfides are also produced as a result of protein fermentation. One example is para -cresol ( p -cresol), a phenolic compound generated from tyrosine, which is a uremic toxin uniquely generated by the microbiome. Members of the Bacteroidaceae, Clostridiaceae, Enterobacteriaceae, and Lactobacillaceae are known to produce p -cresol. Urinary levels of p -cresol are positively correlated with dietary protein levels and commensurately decrease upon transition to diets high in oligosaccharides indicating the use of dietary substrates in its production. The resulting microbe generated p -cresol can affect a variety of host tissues once it enters systemic circulation. p -Cresol reduces spontaneous beats of cardiomyocytes by affecting gap junctions, it drives reactive oxygen species production in osteoblasts, induces insulin resistance, elicits DNA damage in colonic epithelial cells, and has been linked to colon cancer and kidney failure.
An additional secondary metabolite of protein fermentation is hydrogen sulfide (H 2 S). H 2 S has been shown to regulate a variety of physiological processes in the gut including inflammation, motility, genomic DNA damage, and oxidative stress. There are two primary pathways for microbial generation of H 2 S. The first stems from enzymatic breakdown of sulfur containing amino acids like methionine, cysteine, and taurine. Members of the Prevotella , Fusobacterium , Bilophia , Desulfovibrio , and others have been described to perform this chemistry. Only methionine and cysteine are found in dietary protein sources but taurine is frequently added to modern energy drinks due to its proposed but unconfirmed stimulatory effects and thus may be a significant dietary contributor to H 2 S production for some individuals. The second pathway of H 2 S production from microbes stems from the sulfate-reducing bacteria (SRBs, e.g., Desulfovibrio , Desulfobacter ) which generate H 2 S using free hydrogen as the input rather than amino acids.
H 2 S produced from microbial sources can have a variety of effects on host physiology. Increased H 2 S production from probiotic administration of Desulfovibrio elicits a decrease in whole gut transit time, which can be reversed by co-treatment with bismuth—an H 2 S chelator. H 2 S also blocks the oxidation of butyrate in colonic epithelial cells by inhibiting the activity of acyl-CoA-dehydrogenase. The lack of butyrate as an energy source affects multiple epithelial cell functions including ion absorption, mucin synthesis, and membrane lipid synthesis. These collectively contribute to epithelial barrier dysfunction thus it was predicted that H 2 S might play a role in pathogenesis of ulcerative colitis (UC). Interestingly, patients with UC show reduced butyrate oxidation, increased intestinal permeability, are enriched for H 2 S producing bacteria, and have increased fecal levels of H 2 S.
Robust links also exist between protein (particularly from animal sources) and development of colorectal cancer (CRC), and microbes have been implicated in this process. H 2 S produced by microbial protein fermentation can elicit genomic DNA damage in colonic epithelial cells and is predicted to be a contributing factor for CRC, potentially by exploiting polymorphisms in DNA repair systems. It has been demonstrated that patients with previous CRC have elevated H 2 S levels and Fusobacterium, a H 2 S producer, has been shown to be associated with CRC tumors. A definitive mechanistic link between Fusobacterium H 2 S mediated genotoxicity and cancer has yet to be described, but Escherichia coli , another H 2 S generating bacteria, has also been associated with CRC development suggesting that a mechanistic link does exist.
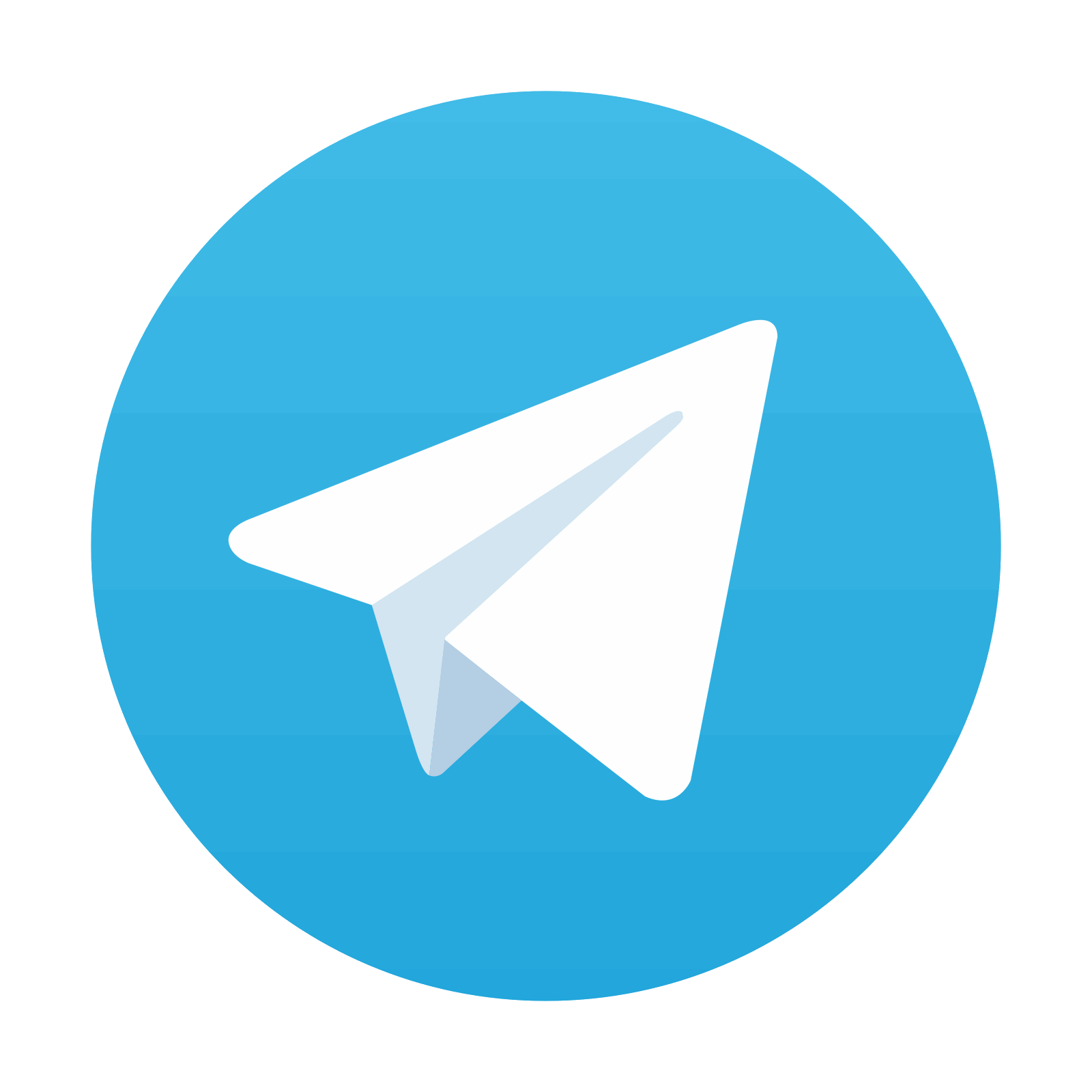
Stay updated, free articles. Join our Telegram channel

Full access? Get Clinical Tree
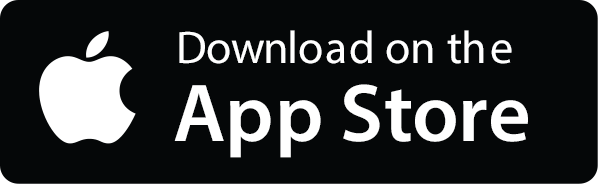
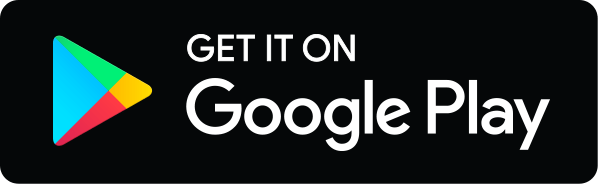