The gut microbiota provides a range of ecologic, metabolic, and immunomodulatory functions relevant to health and well-being. The gut microbiota not only responds quickly to changes in diet, but this dynamic equilibrium may be managed to prevent and/or treat acute and chronic diseases. This article provides a working definition of the term “microbiome” and uses two examples of dietary interventions for the treatment of large bowel conditions to emphasize the links between diet and microbiome. There remains a need to develop a better functional understanding of the microbiota, if its management for clinical utility is to be fully realized.
Key points
- •
Diet has a significant impact on the structure-function activities of the gut microbiota.
- •
Advances have been made in showing how host phenotype is shaped by the gut microbiota, and how diet may provide the selective pressure, in a positive or negative way, to sustain this relationship.
- •
Diet modification offers the opportunity in a clinical setting to reshape the gut microbiota for the relief of symptoms associated with functional disorders, and the therapeutic treatment of some gastrointestinal and extraintestinal diseases.
- •
However, studies need to continue to advance from microbiota profiling to function-based approaches and analyses, and more rigorous study designs also need to be used, to better differentiate between the cause and effect relationships of diet-microbiome interactions, and to translate microbiome research to medicine.
Introduction: what is the microbiome?
There are several extant definitions of the term “microbiome,” a field of research that has become principally associated with the technological advances in DNA/RNA sequencing and computational biology. As such, the microbiome is still commonly defined as the collective genomic content of all microbes recovered from a habitat or ecosystem (eg, saliva and stool samples, skin swabs). However, although such a definition captures the functional potential inherent to the microbiota (micro-), there is a need to place this knowledge in context with the interactions and processes contingent on the physicochemical attributes of their surrounding environment (-biome). This more holistic definition of the microbiome is applied throughout this article, in recognition of diet as a major influence on microbiome dynamics. By doing so, the concept of nutritional ecology is introduced: how the nutrient and its variations across temporal and spatial scales affect the gut microbiota. We contend that nutritional ecology will provide the mechanistic bases for understanding “diet and the microbiome,” which will translate into improved diagnoses and treatments for functional and organic diseases.
Introduction: what is the microbiome?
There are several extant definitions of the term “microbiome,” a field of research that has become principally associated with the technological advances in DNA/RNA sequencing and computational biology. As such, the microbiome is still commonly defined as the collective genomic content of all microbes recovered from a habitat or ecosystem (eg, saliva and stool samples, skin swabs). However, although such a definition captures the functional potential inherent to the microbiota (micro-), there is a need to place this knowledge in context with the interactions and processes contingent on the physicochemical attributes of their surrounding environment (-biome). This more holistic definition of the microbiome is applied throughout this article, in recognition of diet as a major influence on microbiome dynamics. By doing so, the concept of nutritional ecology is introduced: how the nutrient and its variations across temporal and spatial scales affect the gut microbiota. We contend that nutritional ecology will provide the mechanistic bases for understanding “diet and the microbiome,” which will translate into improved diagnoses and treatments for functional and organic diseases.
General concepts and approaches of microbiome studies
For the interested reader, Morgan and Huttenhower present a well-structured and illustrated general overview of the techniques and approaches underpinning microbiome studies. Over the last two decades, microbiome studies have emphasized the use of polymerase chain reaction techniques targeting regions within the gene encoding 16S ribosomal RNA in prokaryotes (ie, bacteria principally, and archaea). When combined with the rapid advances in DNA sequencing technologies and a combination of ecologic, biostatistical, and computational methods, these 16S rRNA profiling methods have resulted in a taxonomy-based assessment of gut microbial communities resident in different regions of the gastrointestinal tract. Importantly, these approaches have afforded the differentiation of the microbiota to reveal specific microbes and microbial consortia indicative of alterations to gut homeostasis, which are generically referred to as “dysbiosis.” During the same period the National Institutes of Health Human Microbiome Project has augmented these studies by producing the “reference genomes” of individual microbial species, which has supported the inference of the functional attributes inherent to the 16S rRNA profiles by such methods as PICRUSt. However, and because of the continued advances in sequencing technologies, the time and cost constraints to “shotgun metagenome sequencing” are being relaxed, which affords a scale and depth of sequence coverage that provides an actual (rather than inferred) representation of the functional attributes inherent to the microbiota.
These studies have also substantiated that the microbial communities of the gut are readily differentiated according to their microbial (and gene) density, diversity, and distribution; as affected by anatomic structure, host secretions, and digesta residence times at different sites. Although the esophageal, stomach, and small intestinal microbiota have now been characterised, most studies that have advanced the mechanistic understanding of diet-microbiome interactions have been undertaken using stool/fecal samples and/or tissue samples collected from the large bowel. As such, the term “gut microbiome” has come to define this (terminal) region of the gastrointestinal tract. During the last 5 years in particular, shotgun metagenome sequencing of stool microbiota and the associated metagenome-wide association studies has revealed that the form and function of the stool microbiota is altered in patient cohorts with type-2 diabetes, cirrhosis, and colorectal cancer. These differences have not only provided insights of how microbial metabolism contributes to disease, but the identification of candidate gene and organismal biomarkers of health and disease.
Critically, these methods have also shown the gut microbiota of humans (and other animals) is rapidly altered by changes in habitual or available diet, leading to the perception that diet may exert a stronger selective pressure on the gut microbiota than host genetics. A particular focus in the last 10 years has related to obesity research, with Turnbaugh and colleagues reporting an enrichment of microbial genes involved in carbohydrate, lipid, and amino acid metabolism in the obese adult gut. More recent studies have revealed that non-obese and obese individuals are characterized by variations in gene richness: subjects with a low gene count are characterized by increased adiposity, insulin resistance, and inflammation. The difference in gene richness has been suggested to be predictive of previous weight gain and in mice, could be partially reversed following a dietary intervention for weight loss. The links between diet and microbiome have also been further substantiated via fecal transplant studies in animal models. For instance, Turnbaugh and colleagues were among the first to show how the transfer of an obese (or lean) phenotype to a naive host (ie, germ-free mice) can be effectively recapitulated using diet to exert the necessary selective pressure to sustain the microbiome. This type of an approach is being increasingly used to establish how either specific microbes or microbial consortia contribute to the onset of non-communicable metabolic and immune-mediated diseases. Additionally, the benefits of existing and candidate next-generation probiotic strains, in terms of their capacity to attenuate inflammation and/or positively affect barrier function and host metabolism (eg, Bifidobacterium spp, Faecalibacterium prausnitzii and Akkermansia muciniphila ), are being examined by their introduction to either germ-free or conventionally reared mice.
Table 1 provides a summary of some recent advances in the understanding of how diet and the gut microbiome, from ecologic, metabolic and immunomodulatory contexts, can affect gut function and health. In addition to the other contributions provided in this issue, there are numerous books and reviews of the topic, especially as it pertains to diet-microbiome (diet × microbiome) interactions during pregnancy and early life, obesity and metabolic diseases, and immune development and immune-mediated diseases. Indeed, these interrelationships are now being defined from conception to grave: from their influences on fetal and infant developmental biology and homeostatic processes, to triggering a plethora of acute and chronic (extra) intestinal diseases, through to defining rules for microbiome restoration to better treat diseases and prevent relapse. Here, as a primer for gastroenterologists, we provide two examples of how diet has been used as a primary intervention that affects the microbiome: exclusive enteral nutrition (EEN) and diets with reduced fermentable oligo-, di-, monosaccharides and polyols (FODMAP) as induction therapies for Crohn’s disease (CD) and irritable bowel syndrome (IBS), respectively. These two examples provide insights into how the microbiota is affected by these conditions, how diet can be managed to produce positive health outcomes, the consequences of these interventions on the microbiome, and why improved knowledge of diet × microbiome interactions is needed.
Diet | Ecological Effect | Microbiota Effect |
---|---|---|
Breastmilk | Provides human milk oligosaccharides | Promotes growth of Bifidobacteria and Lactobacillus |
Low FODMAP diet | Mitigates IBS symptoms | Alterations in bacterial taxa |
Exclusive enteral nutrition | Induces remission and promotes healing in CD | Alters structure-function activity of the microbiota |
Grains, resistant starches, “fibers” | Promotes SCFA production and reduced pH | Suppresses growth of Gram-negative Enterobacteria |
Anti-inflammatory effects | Promotes growth of acetate and butyrate producers Stimulates Bifidobacteria and F. prausnitzii |
Dietary Metabolites | Metabolic and/or Immunomodulatory Effects | Microbiota Effect |
---|---|---|
SCFA | Metabolic effects via specific GPCR receptors Effects of butyrate on Treg cells | Variations in proportions and total SCFA produced Loss of butyrate producing commensals with inflammation (eg, F. prausntizii ) |
Trimethylamines | Risk factor for cardiovascular and renal disease | Microbial by-products of choline and creatinine |
Branched-chain amino acids | Promotes insulin resistance and diabetes | Enrichment of these pathways in microbiome of T2D |
Primary bile acid metabolites | Variations in host signaling via NR and GPCR | Gram-positive and sulfidogenic bacteria, Bacteroides |
Riboflavin precursors | Activation of MAIT cells | Produced mainly by Gram-negative enteric bacteria |
Anti-inflammatory peptides | Anti-inflammatory effects | Loss of F. prausnitzii associated with inflammation |
Polyphenols | Improve barrier function and proglucagon levels | Stimulation of A. muciniphila populations |
The microbiome and inflammatory bowel disease
The cause of inflammatory bowel disease (IBD) remains elusive because it is a multifactorial disease influenced by the complex interplay between host genetic susceptibilities, environmental, and lifestyle choices (eg, habitual diet, smoking). With the global incidence and prevalence of IBD increasing, and reaching across to historically low-risk ethnic groups and countries, the microbiome is widely perceived to represent the functional interface among these three components precipitating disease. Indeed, Sartor proposed variations in the balance of the gut microbiota as a trigger for disease, and a large number of case-control and observational studies of the stool and mucosa-associated microbiota from patients with new-onset and chronic IBD now show an overall decrease in bacterial diversity of adult and pediatric patients with IBD during active disease, when compared with healthy control subjects. Reductions in bacterial lineages associated with the Gram-positive Clostridium leptum and Clostridium coccoides clusters are consistently reported in patients with IBD. Members of these lineages are recognized to produce short chain fatty acids including butyrate during active growth in the large bowel, and at least some are implicated in driving the expansion of regulatory T-cell populations in the gut that suppress the inflammatory response. Furthermore, reductions in the abundance of F. prausnitzii , which in healthy subjects may comprise approximately 5% of the total population, are frequently reported for patients with CD and ulcerative colitis (UC). Longitudinal studies have also revealed that these changes are coincident with the onset of disease and that the restoration of these bacterial taxa is prognostic of better health outcomes. Conversely, an increase in the relative abundance of pro-inflammatory Gram-negative bacteria principally affiliated with the Proteobacteria has been consistently identified in IBD, and some Bacteroides spp have also been implicated in the onset of IBD and flares. Much less is known about the diversity of the non bacterial members of the healthy gut microbiota. Our own studies revealed a strong signal for methane-producing archaea associated with colonic tissue, although reductions in the absolute counts and taxonomic composition of archaea and fungi have been reported for patients with IBD compared with healthy subjects. These findings strongly suggest that although the anti-inflammatory factors produced by such bacteria as F. prausnitzii are sustained and robust in healthy subjects, the growth, persistence and metabolic activity of these bacteria can be challenged by not-yet-identified factors that change within the gut milieu during active disease episodes. At the same time, pro-inflammatory bacteria seem to thrive in these same conditions but whether inflammation is the cause, or the consequence of these interrelationships still needs to be established.
Starving the “Hungry Microbiome” as an Induction Therapy for Inflammatory Bowel Disease?
In some respects, the understanding of the contributions of diet to the cause and treatment of IBD has been historically marginalized, limited mainly to anecdotal observations, surveys, and reports rather than robust experimental designs. However, and perhaps because of the rapid emergence of study of the gut microbiome, and the recognition of the dynamic relationships between diet and the microbiota, a more systematic assessment of these interrelationships has been forthcoming, offering new opportunities with real clinical impact. Currently, the most notable of these is the use of enteral feeding of a nutritionally replete liquid formula (EEN) in pediatric patients with CD, which can induce clinical remission and mucosal healing rates approaching 80% of newly diagnosed subjects. Various types of EEN formulae exist and are classified as elemental (free amino acids), semi-elemental (peptides), or polymeric (proteins), with the polymeric formulae considered to be more palatable. The formulae do not vary in their ability to induce or maintain remission and moreover, they are superior to corticosteroids in their ability to induce mucosal healing in patients with CD, which is the greatest predictor of long-term health outcomes. Although EEN is predominately used with pediatric patients it has also been shown to result in positive outcomes with adults, although it suffers from lower compliance.
Although the exact mechanisms of action remain unknown, EEN has been proposed to have positive impacts on the host and microbiota. In general terms, studies of the stool microbiota have shown that EEN results in a further reduction in bacterial diversity, and in particular, reductions in those bacterial taxa assigned to the two most predominant phyla (ie, Firmicutes and Bacteroidetes). In addition to EEN, recent systematic reviews have concluded that alternative dietary intervention strategies can modulate the symptoms of IBD. In particular, the exclusion of foods that caused symptoms or that resulted in a blood serum IgG response to food antigens are associated with improved outcomes. Low residue or fiber diets do not exert a therapeutic effect, although there is limited evidence that a diet with reduced FODMAPs may also help control the functional symptoms associated with IBD. Epidemiologic and animal studies have increasingly implicated high-fat diets and food additives, such as emulsifiers, as plausible triggers of IBD and it remains to be determined whether these risks could be ameliorated by custom dietary interventions.
In summation, EEN either alone or in combination with exclusion diets, and in young patients with CD in particular, result in reductions in the bacterial diversity associated with stool and mucosal samples, and the period of “bowel rest” and mucosal healing afforded by EEN may result from limiting the growth of the hungry microbiome and minimizing their triggers of inflammation. In addition, as outlined in our recent review, the process of mucosal healing that is characteristic of EEN treatments may also be partly supported by induction of the autophagy pathways to help repair damaged host cells. Surprisingly, however, current EEN formulations are considered to be ineffective for the treatment of UC, although it has been applied as an adjunct therapy; and exclusion diets specific for UC are in development (Levine and Lewindon, personal communication, 2016). However, randomized control trials have shown fecal microbiota transplants are a promising intervention for the treatment of UC, in addition to their use for the treatment of recalcitrant Clostridium difficle infections, although their impact in CD is more variable. For these reasons, we believe that diet × microbiome interactions will soon be further understood and effectively managed, to provide synergistic interventions (eg, exclusion diets, partial enteral nutrition, and/or microbiota transplants) that will advance the treatment and clinical management of both major types of IBD.
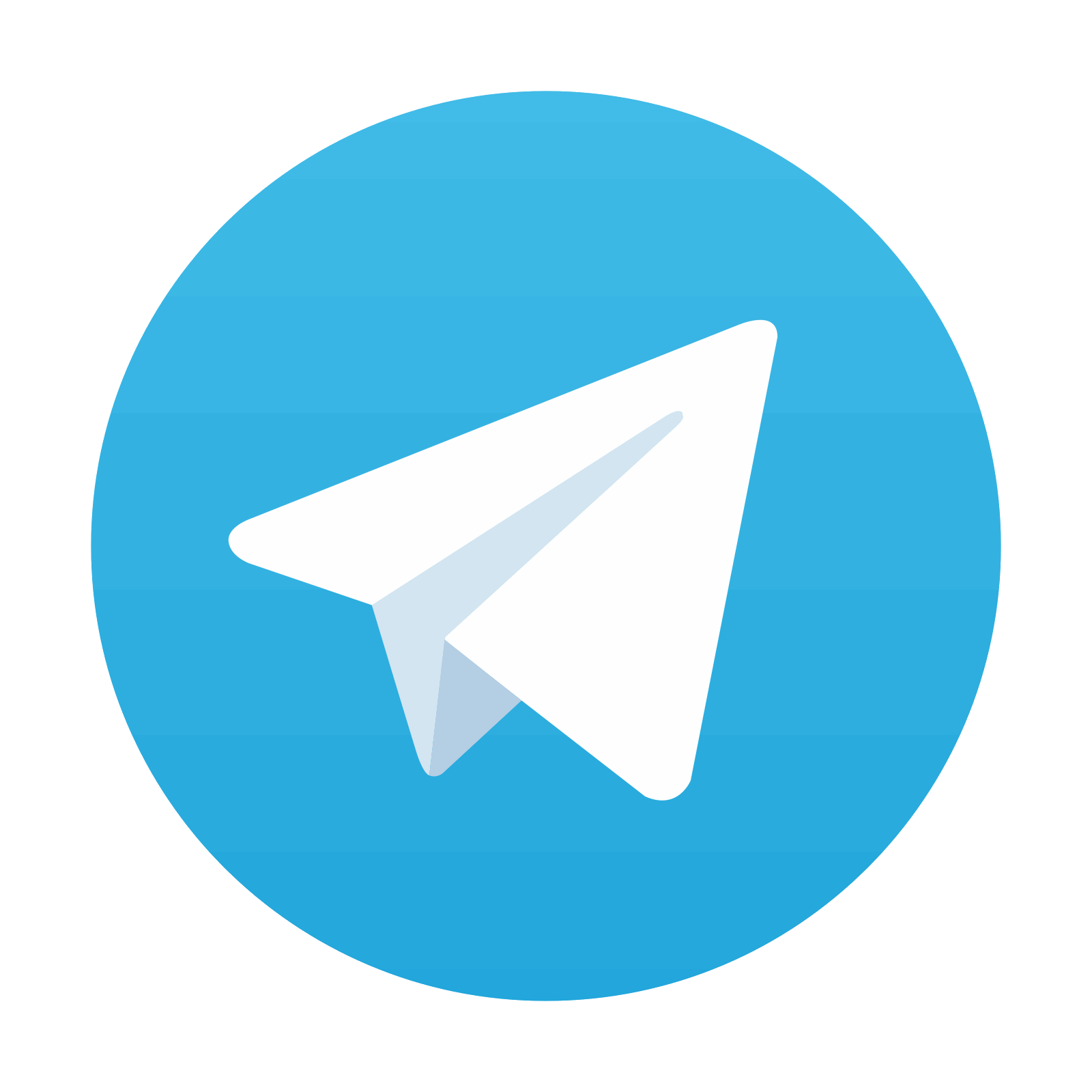
Stay updated, free articles. Join our Telegram channel

Full access? Get Clinical Tree
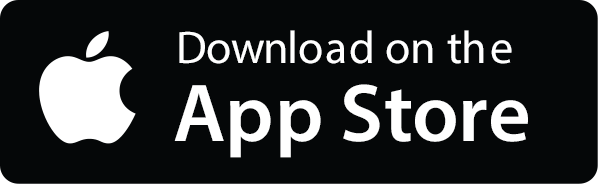
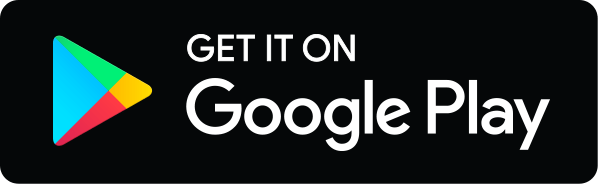