Fig. 4.1
Stones could be broken ureteroscopically using the tactile technique. The stone was visualized, grasped in a basket and pulled to the tip of the ureteroscope. The ultrasound probe was felt against the stone and then as it cleared the stone with fragmentation
The next step included downsizing the ultrasonic transducer to 4Fr and development of a rigid ureteroscope with a straight channel. The design of the ureteroscope included an angled eyepiece so that the working channel passed straight through the endoscope. There was a side port to introduce a basket through the scope. The ultrasound probe was reduced to 4Fr. With these devices, the stone could be engaged in the basket and the probe passed under direct vision to touch and fragment the stone. Only tiny fragments could be removed through the probe but the stone could be treated to be cleared (Fig. 4.2).


Fig. 4.2
An endoscope with an off angle lens, a straight channel and a side channel permitted visual ultrasonic lithotripsy
Another endoscopic device to fragment stones is the electrohydraulic lithotripter (EHL ). Developed in the 1970s, it had been used successfully to fragment bladder stones with probes as large as 7 and 9Fr [16]. When these were used blindly in the ureter there was significant damage with resultant stricture. After the probes were downsized to 3Fr they could be placed through the channel of the ureteroscope to fragment stones under direct vision. The safety of this combination was well documented [17, 18]. The probes have been downsized even further to 2.5, 1.9 and 1.7Fr and have the advantage of being very flexible. They could be used with flexible ureteroscopes as they became available.
The development of these instruments allowed routine access to the distal ureter and frequently access to the proximal ureter and even the renal pelvis. Thus it became evident that there was a need for a flexible instrument which could give visualization and access to the entire intrarenal collecting system.
Initial attempts with the non-deflecting or passively deflecting scopes showed their in-adequacies. Even attempts to place them through deflecting and irrigating sheaths were unsuccessful. Similarly, small ureteroscopes which could be passed through the rigid ureteroscope sheath experienced inadequate irrigation and deflection [19].
In the 1980s, deflectable flexible ureteroscopes became a reality. These instruments had a channel and could be inserted over a wire. They did not require the stabilizing sheath needed with the earliest models. Active primary and passive secondary deflection were used to gain access to the lower pole. Deflection of 175° was used as the benchmark to reach the lower pole. This was based on observations from contrast radiographs [20]. However, deflection is often limited by instruments in the channel or the pattern of the collecting system itself. Deflection has been further enhanced with active secondary deflection or continuous controlled deflection which allows the tip to advance further into the lower pole [21] (Fig. 4.3).


Fig. 4.3
Fluoroscopic image of a flexible ureteroscope entering an anterior lower pole calyx in a dilated collecting system
The first practical, deflectable flexible ureteroscope was a prototype from Olympus. One unit in the USA in the early 1980’s was shared between Robert Kahn in San Francisco and D. Bagley in Philadelphia and made two trips weekly via Federal Express to be available at both sites. This was a modification of a pediatric bronchoscope. It was designed with maximum deflection in the upward direction with downward movement of the control lever activated by flexion of the thumb.
The next entry was the AUR series from ACMI. The AUR8 was 8.5 French with a 2.5Fr channel while the AUR9 was 9.8 with a 3.6Fr channel. They both had deflection in a single direction. Downward deflection of the thumb lever gave downward deflection of the tip of the endoscope. This was chosen because most attempts at deflection would be to get the tip down into the mid to lower calyces of the kidney. It also uses the most efficient movement of the thumb, flexion, to activate downward deflection. It was considered logical deflection. Down is down. Another innovation in these endoscopes was angulation of the optical system at approximately 8° toward the working channel. In this way, the device exiting the working channel could appear in the center of the field of view. With a 0° angle, the device would be parallel to the imaging axis and would never enter the center of the field.
The next entry in the series was the AUR7 which had 2 way deflection and a 7.4Fr shaft throughout the 30 cm working length. This size was much easier to place into the ureter. Initially, the shaft was slightly tapered from the tip proximally to the handle. It was very difficult to manufacture and a change was made to put a step down segment at approximate 30 cm. It thus became unstable at that point and the shaft tended to twist as the endoscope was advanced and rotated. Only larger diameter flexible ureteroscopes were produced by any manufacturer after that point with a hope for durability.
Fiberoptics remained the optical system for visualization until the introduction of the first digital flexible ureteroscope. These were also termed videoscopes or chip on a stick [22]. The early versions suffered from their large size (nearly 12Fr) and high cost. After Storz first introduced their video ureteroscope with a shaft of 8.4Fr, other companies gradually decreased the size to approximately 8.5Fr currently used. Most of the video endoscopes have been filtered and shielded to allow use of the Homium laser. Most are not equipped to use the Neodynium:YAG laser and lose the image to white out when it is activated. In one disposable version, we noted that activation of the electrocautery unit also caused loss of the image. Although these endoscopes remain expensive, there is considerable competition and the cost of chips has been falling significantly. This may be reflected in the cost of the endoscopes in the near future (Fig. 4.4).


Fig. 4.4
Urinary neoplasms shown with (a) a fiber optic endoscope showing broken fibers and the fiber pattern (b) image with a digital endoscope
Flexible ureteroscopes suffer from expense and fragility. The longevity of scopes in clinical use has been reported to be from 10 to 100 uses before breakage. Most reports indicate a range of 20–40. The single report showing 100 uses was in a limited practice of only two urologists where the instruments are cleaned and then soaked for disinfection in the endoscopy suite [23].
One solution proposed for the problem of sterilization and breakage has been single use, “disposable” endoscopes. In the early models available from two different companies, the flexible portion of the scope was disposable while the handle containing the optics was reusable. These were both fiber optic instruments. The first practical disposable ureteroscope was produced by VanTec. There were two designs with flexible but nondeflectable tips of 7 and 8.5 French with a working channel (Fig. 4.5). T hey also created a small rigid endoscope at 7Fr. The entire project was discontinued after the company was acquired by Boston Scientific.


Fig. 4.5
The modular VanTec ureteroscope with interchangeable disposable tips
Bard presented a flexible but deflectable ureteroscope with a similar modular tip and reusable eyepiece/handle. The deflecting mechanism used a turn knob which was slow and difficult to use. The production models presented an image which was upside down and backward. The endoscope was not clinically useful.
Other entries have been seen and each has failed because of design flaws. In some, the deflection mechanism was difficult or impossible to use and in one model the shaft was not torque stable so that it could not be rotated.
The latest entry (2016) is the single use LithoVue (Boston Scientific, Marlborough, MA) which is a fully deflectable digital ureteroscope. The shaft is 9.5 French and is torque stable with a firm durometer which allows it to be placed into and advanced within the ureter. The image has the typical superior quality of the digital instruments.
During the 1980’s, rigid endoscopy was improved with the development of smaller, multi-channel scopes. The overall size was reduced by replacing the rod lens system with fiber optic imaging bundles. These have been referred to as “semi rigid ” ureteroscopes. They are composed of metal and are truly rigid but have some ability to flex without breaking. The first semi rigid scope had two working channels each 2.3F and the distal tip at 7.2F [24]. This scope could be passed directly into the ureteral orifice without dilation. It was designed specifically for the pulsed dye laser. The small channels were adequate for laser fibers but not for any retrieval devices available at the time. A similarly sized endoscope with a 3.4 and 2.3F channels was adequate for laser fibers and 3F working instruments soon became available [25].
This latter endoscope (the ACMI MR-6) became very popular because 3Fr working devices had become available and could fit through the larger channel. It was also available to any customer and not just laser owners. Both of these ureteroscopes had a flat tip. Their acceptance and widespread use demonstrated the capabilities of the design. Another design with a beak or a lip was a holdover from the tip of a cystoscope adapted from the Timberlake obturator. That curve at the tip had been made for the male urethra and is clearly not necessary for ureteral endoscopy.
Changes in endoscopes would have been meaningless without changes in working instruments, endoscopic lithotriptors and ablative devices. Size and effectiveness were the two factors resulting in true progress. Baskets and graspers were available in sizes of 5Fr and greater before the endourology revolution. As the limits of size for ureteroscopic instruments was recognized, they gradually decreased in overall diameter. As Jim Vance of VanTec stated in the 1980s when a 4Fr basket was requested, “that’s really small.” Now baskets and other devices have gradually decreased to 3Fr, 2.5 and even sub 2Fr sizes.
The initial baskets were helical, or Dormia design made in stainless steel. A major step was the introduction of the Segura or flat wire basket composed of two perpendicular loops. It offered a greater area between the wires so that larger stones could be captured. It was particularly useful percutaneously but still had a role in ureteroscopy for biopsy. Another, even greater, advance was the introduction of nitinol wires for the construction of baskets. This material had memory for shape and did not kink. This property in contrast to stainless steel, which can kink thus trapping the stone, allowed for baskets which could be used in the ureter [26].
The ultrasonic lithotriptor was the first endoscopic device for breaking stones. Its major advantage was that it could remove fragments. However, it was not very powerful and the probe was rigid. It still has a major role for percutaneous procedures but has not been useful for ureteroscopy. Impact devices for breaking stones date from their use in the nineteenth century for bladder stones. The development of small powerful probes made these devices very useful in ureteroscopy and percutaneous nephroscopy. The effect of the impactor is directly at the tip of the probe and there is no lateral scatter of energy. It is a very effective lithotriptor but the fragments remain in place to pass or to be removed. There are several impact devices available in the market now and they all have very high success rates [27, 28].
Lasers have gained a dominant role in ureteroscopy. The small fibers are perfectly suited to the small ureteroscopic instruments. The first practical laser for lithotripsy was the pulsed dye laser [29]. As the instruments became more powerful they became effective even for the very hard calcium oxalate monohydrate stones [30]. The disadvantages were that they were expensive and difficult to maintain and could only break stones.
As soon as the holmium laser became available, its many advantages were recognized. Johnson and Webb reported the capabilities of the laser including ureteroscopic lithotripsy [31, 32]. It could break stones, cut and ablate tissue. Despite these mixed capabilities, it was safe because its action was only at the end of the activated fiber. There was minimal penetration of approximately 0.5 mm in water. The laser energy could be delivered through fibers ranging from 100 to 1000 μm in diameter. It can be used throughout the ureter and the intrarenal collecting system. It can be positioned through a flexible ureteroscope into the lower pole. Despite its cost, it has become the dominant intracorporeal lithotriptor. The major concerns and reports have shifted to variations in techniques. These include dusting versus fragmenting stones, multiple pulse applications, pulse duration, optimal fiber size and shape, and fiber shielding.
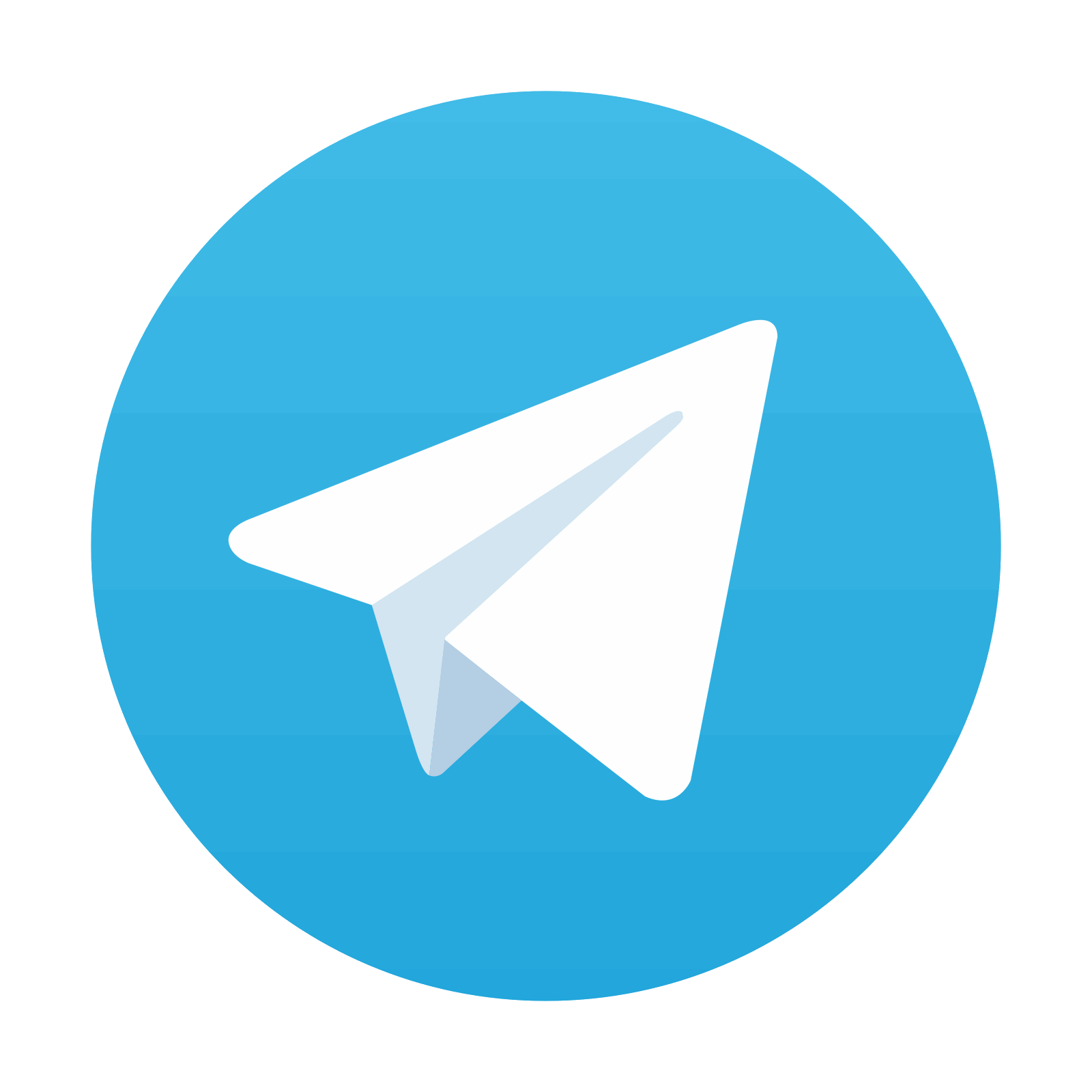
Stay updated, free articles. Join our Telegram channel

Full access? Get Clinical Tree
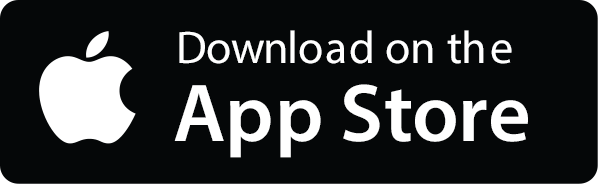
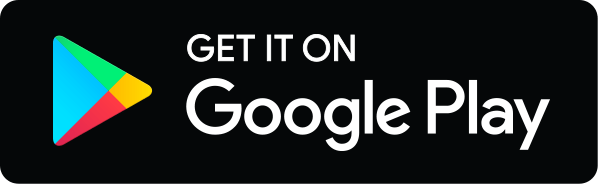