Male infertility associated factor
Percentage
Idiopathic
31
Maldescended testis
7.8
Infection
8
Disorders of semen deposition and sexual factors
5.9
Systemic disease
3.1
Varicocele
15.6
Endocrine
8.9
Immunologic
4.5
Obstructions
1.7
Other
5.5
Beyond these basic tests the evaluation for UMI may include postcoital testing, motility assessment, and sperm function tests, including fertilization potential , reactive oxygen testing, and chromatin defects [7]. Based on the findings from these studies treatments can be recommended. In this chapter, we will continue to define and discuss the epidemiology and potential etiologies of UMI.
Semen Analysis
Semen analysis is standard for the evaluation of male factor infertility. Approximately 40 % of infertile men have normal semen analysis parameters [8]. The test is generally repeated if the first sample is abnormal. Tested parameters include semen volume, sperm concentration, total count, motility, and morphology [5]. All these parameters can reveal significant information about testicular and auxiliary gland function and reproductive anatomy. In 2010, the World Health Organization (WHO) changed the standard values where the reference values were lowered based on a population study of fertile men from seven countries [9, 10]. It is recommended to use the WHO guidelines with caution for men who have normal semen quality because men can be infertile even though their sperm counts are above the lower limit of WHO criteria [11].
Low sperm concentrations are associated with low likelihood of pregnancy but in contrast higher sperm concentrations are not associated with increased likelihood of pregnancy [11]. Although semen analysis is critical in the evaluation of male factor infertility it rarely provides a diagnosis [12]. Semen analysis can provide reasonably precise prognosis only if the parameters are at extreme levels [12]. Spontaneous pregnancy rates in couples where the male had normal and abnormal sperm parameters have been 24 and 23 %, respectively [8] .
In UMI, the semen analysis is generally normal and given the recent changes in the WHO classification more men are now placed into this category. In these men, specialized testing is undertaken since standard semen analysis does not take into account several steps of the fertilization process such as sperm transport , sperm interaction with the cervical mucus , and/or oocyte. In a small number of patients, these tests may assist in deciding treatment options . These tests can focus on immune disorders, sperm genetic defects, environmental factors , and fertilization defects.
Immune Disorders
Since sperm are produced after puberty they are at risk for an autoimmune response from the developed immune system. They remain protected by the testicular blood-testis barrier and secretion of immunosuppressive agents by macrophages and/or sertoli cells [13]. The barrier protects postmeiotic spermatocytes and mature germ cells in the adluminal compartment [14]. However, spermatogonia and early spermatocytes are not protected because they develop below this layer and depend on the secretion of immunosuppressive agents. These barriers can be destroyed after trauma, vasectomy, orchitis and/or epididymitis, varicocele, and spinal cord injury [15−21]. This then allows the formation of antisperm antibodies (ASA) , which can be found outside the sperm on seminal plasma, cervical mucus , and follicular fluid. Sperm antibodies are polyclonal and can bind multiple sperm antigens. The presence of antibodies does not always lead to functional sperm impairment.
Direct (detecting ASA on the sperm surface) and indirect (detecting ASA in the female genital tract or partners serum) testing can help identify ASA [22]. Both direct immunobead (D-IBT) and mixed antiglobulin reaction (MAR) tests show sperm bound ASA. Sperm immobilization test (SIT), tray agglutination test (TAT), gel agglutination test (GAT), enzyme-linked immunosorbent assay (ELISA), flow cytometry, and radioimmunoassay tests can indirectly measure ASA within seminal fluid, sperm extract, cervical mucus, and/or sera. Each of these tests has its advantages and disadvantages in terms of sensitivity, specificity, providing quantitative information, requirement of skilled staff, and cost [23].
The actual ASA prevalence is limited by the test utilized for detection. Analysis of serum (indirect) from 698 infertile couples revealed 31.1 % of couples (16.5 % of the men and 21.6 % of the women) possessed at least one positive result for ASA [24]. In another study, serum from 186 men and 194 women and cervical mucus of 155 women and semen of 202 men were evaluated with direct and indirect immunobead binding tests. ASA positivity was seen 11.1 % (7 % males and 4.1 % females). Overall, 3.2 % of cervical mucus and 10.4 % of semen samples were also ASA positive [25]. In a study evaluating 471 couples with direct testing using D-IBT, TAT, and GAT, 8.1 % of males and 3.1 % of females were found to be ASA positive [26]. Busacca et al. demonstrated that 16.2 % of the men and 7.3 % of the women in infertile couples had antibodies for sperm antigens [27].
Menge et al. found that the incidence of pregnancy was influenced significantly by the presence of circulating ASA in an infertile couple [24]. Prefertilization effects from ASA include sperm agglutination , sperm cytotoxicity , poor cervical penetration, acrosome reaction, and poor oocyte binding [28, 29]. Sperm agglutination can impair motility and cervical penetration and is higher in patients with the presence of ASA [30, 31]. ASA can also activate complement-mediated cellular lysis of the sperm [32]. A study comparing fertile nonautoimmune and infertile autoimmune men showed that sperm counts and motility were significantly lower in autoimmune infertile men than their fertile controls [33]. The presence of autologous cytotoxic antibodies lowers motility and leads to decreased sperm survival [34]. ASA also leads to decreased sperm penetration into cervical mucosa [27, 35] and decreased capacitation by inhibiting the fluidity of the plasma membranes of human spermatozoa [36]. Acrosome reaction is another important phase of fertilization process and Bandoh et al. found the inhibition of this reaction by ASA [37]. Furthermore, inhibited sperm-zona pellucida binding has been suggested by Liu et al [38].
Postfertilization antibodies can affect the viability of the embryo. ASA against sperm-derived protein CS-1 may play a role in postfertilization embryo defects [39]. Witkin and David demonstrated ASA on female sera and ejaculated sperm can cause unsuccessful conception and first trimester abortion [40]. In another study, Mandelbaum et al. found significant levels of ASA in women with unexplained infertility who had undergone in vitro fertilization/embryo transfer (IVF/ET) than women who had only tubal infertility [41]. All these studies suggest that ASA can cause infertility blocking different phases of fertility in the presence of normal semen parameters .
Genetic Disorders
Genetic disorders can lead to infertility by altering spermatogenesis and/or sperm function . These disorders include karyotype abnormalities (both autosomal and/or sex chromosomal) and deletions or mutations of specific genes within various chromosomal loci. In a review of 9766 infertile men with azoospermia and/or oligozoospermia the incidence of chromosomal abnormalities was 5.8 % (autosomal anomalies 1.5 % and sex chromosome anomalies 4.2 %) [42]. This incidence was significantly higher compared to the 0.38 % (0.14 % sex chromosomal and 0.25 % autosomal) reported in routine newborn screens [43]. The incidence of karyotype anomalies is inversely proportional to sperm concentration and is therefore less than 1 % in patients with normal sperm count [44].
Polymerase gamma (POLG) is a key enzyme involved in the elongation and repair of mitochondrial DNA strands that encode for the POLG gene. Studies have shown an association between POLG gene polymorphisms and UMI [45, 46]. It has been demonstrated that polymorphism of this gene can decrease sperm oocyte penetration and fertilization when sperm parameters are normal [45, 46]. This information may prove beneficial when recommending infertile couples for assisted reproduction.
Previous studies have shown that sperm DNA integrity has predictive value for both normal physiologic and assisted reproduction [47−49]. Protamine deficiency, oxidative stress , unrepaired DNA breaks during chromatin remodeling, abortive apoptosis during spermatogenesis , endogenous endonucleases, caspases, exogenous gonadotoxic agents, and the reactive oxygen species can cause DNA damage [50]. It has also been found that a negative correlation exists between semen parameters and sperm with fragmented DNA [48]. In comparison, infertile men who have normal semen analysis may also have higher percentage of sperm DNA fragmentation. Saleh et al. demonstrated that 43 % of infertile men who have normal sperm parameters have higher DNA fragmentation index then fertile counterparts [51]. Avendano et al. have demonstrated that the DNA fragmentation in morphologically normal spermatozoa has a statistically significant negative effect on embryo quality and pregnancy outcome in intracytoplasmic sperm injection (ICSI) patients [49]. Therefore, the sperm DNA damage can be another responsible factor for UMI. Terminal deoxynucleotidyl transferase-mediated deoxyuridine triphosphate nick end labeling (TUNEL), sperm chromatin structure assays (SCSA), and sperm chromatin dispersion (SCD) have been utilized to measure sperm DNA fragmentation.
Environmental Factors
Oxygen is essential in maintaining the lifecycle and is vital to several biochemical reactions. Reactive oxygen species (ROS) are free radicals that are derived from the metabolism of oxygen. Approximately 30 to 80 % of infertile patients may have high levels of seminal ROS [52]. The term free radical is used to define molecules that contain an unpaired electron in their outer layer. These molecules are highly unstable and include superoxide (O2 −), hydrogen peroxide (H2O2), and hydroxyl (OH−) radicals [52]. These molecules have an important role in the phagocytosis of microorganisms by neutrophils and macrophages. In order to protect the harmful effects of ROS, there are a variety of defense mechanisms, including enzymes (superoxide dismutase and catalase) and small molecules called antioxidants (vitamin E, C, and uric acid). Total antioxidant capacity (TAC) can be measured with enhanced chemiluminescence techniques [53]. Normally there is a balance between ROS and antioxidant system; distortion of this balance can cause excessive accumulation of ROS. Oxidative stress is used to describe this state of excess generation of ROS and diminished capacity of free radical scavenging by antioxidants [52].
Oxidative stress is a potential source for DNA fragmentation as well as abortive apoptosis and deficiencies in DNA recombination and chromatin packing [54]. ROS-induced DNA damage can lead to apoptosis and resultant decrease in sperm counts. The significance of sperm DNA integrity in both physiologic and assisted reproduction has been demonstrated [47−50]. Host et al. demonstrated the negative correlation between disrupted DNA integrity and fertilization rates in men with UMI undergoing IVF treatment [55]. They suggest the measurement of DNA strand breaks in spermatozoa in patients undergoing IVF with a history of unexplained infertility .
It has been shown that ROS-mediated damage to the sperm plasma membrane can change the membrane fluidity through lipid peroxidation, which adversely affects sperm-zona interactions [56]. De Lamirande et al. also found that ROS can decrease sperm motility reducing ATP levels [57]. All these are factors that can affect potential fertility.
Normal physiologic levels of ROS are needed for maturation, capacitation hyperactivation, acrosome reaction, and sperm–oocyte fusion [58]. Excessive levels of ROS are detrimental through lipid peroxidation, DNA damage , and apoptosis. It has been established that endogenous sources of ROS are leucocytes and immature or abnormal morphologic sperm [59, 60]. Henkel et al. found that leucocyte-derived (extrinsic) ROS negatively correlates with sperm concentration and motile sperm count. They also found another negative correlation between sperm-derived (intrinsic) ROS and motility and motile sperm count [61]. Increased ROS has been linked to smoking, alcohol, varicocele, and environmental factors such as radiation, heavy metals, and biological hazards [51, 62−65]. Therefore, a detailed patient history is essential when evaluating UMI .
The association between oxidative stress and idiopathic male infertility is well demonstrated by Pasqualotto et al. They found higher ROS levels as well as lower TAC levels in idiopathic infertile men compared to the control group [66]. Oxidative stress is clearly associated with male factor infertility by altering sperm quantity and quality, sperm function , and sperm–oocyte interaction [58, 66, 67]. It has also been verified that higher ROS levels can reduce fertility capacity without altering sperm parameters [68]. Pasqualotto et al. reported higher level of ROS in normospermic infertile patients than normal healthy man. They also concluded that oxidative stress may explain previously unexplained infertility in men [68]. Shekarriz et al. showed 40 % increased ROS formation in suspected subfertile men who had at least 60 × 106/ml sperm count [69]. In another study, 28 UMI patients with normal semen parameters compared with 30 normal fertile controls had significantly higher ROS and fragmented DNA levels (79 and 89 %) [70]. These studies suggest that ROS is one of the possible causes for UMI .
Fertilization Defect
Hyperactivation of the human spermatozoa during capacitation is associated with infertility [71−74]. Defects in capacitation and sperm mobility in males who have UMI are related to lower IVF rates [75]. Increased flagellar Ca+2 through plasma membrane CatSper channels triggers sperm hyperactivation [76]. Mutations in the CatSper channel genes can be considered another potential cause in UMI [7, 77].
Another important process of fertilization is sperm–oocyte interaction and acrosome reaction in sperm after this interaction. Defective sperm-zona pellucida binding is found in 13 % of infertile men with normal semen parameters. This study also showed that 27 % of these men have defective zona pellucida-induced acrosome reaction [78] .
Conclusion
Approximately half of infertility is secondary to a male factor. Males with UMI have normal examination and semen parameters . The causes of UMI can be immunologic, genetic, structural, or environmental. A high index of suspicion needs to be present for these disorders to trigger further testing.
References
1.
Practice Committee of the American Society for Reproductive Medicine. Definitions of infertility and recurrent pregnancy loss: a committee opinion. Fertil Steril. 2013;99(1):63.CrossRef
2.
3.
Rowe PJ, Comhaire FH, Hargreave TB, Mahmoud AMA. WHO manual for the standardized investigation, diagnosis and management of the infertile male. Cambridge:Cambridge University Press; 2000.
4.
Thonneau P, Marchand S, Tallec A, et al. Incidence and main causes of infertility in a resident population (1,850,000) of three French regions (1988–1989). Hum Reprod. 1991;6:811–6.PubMed
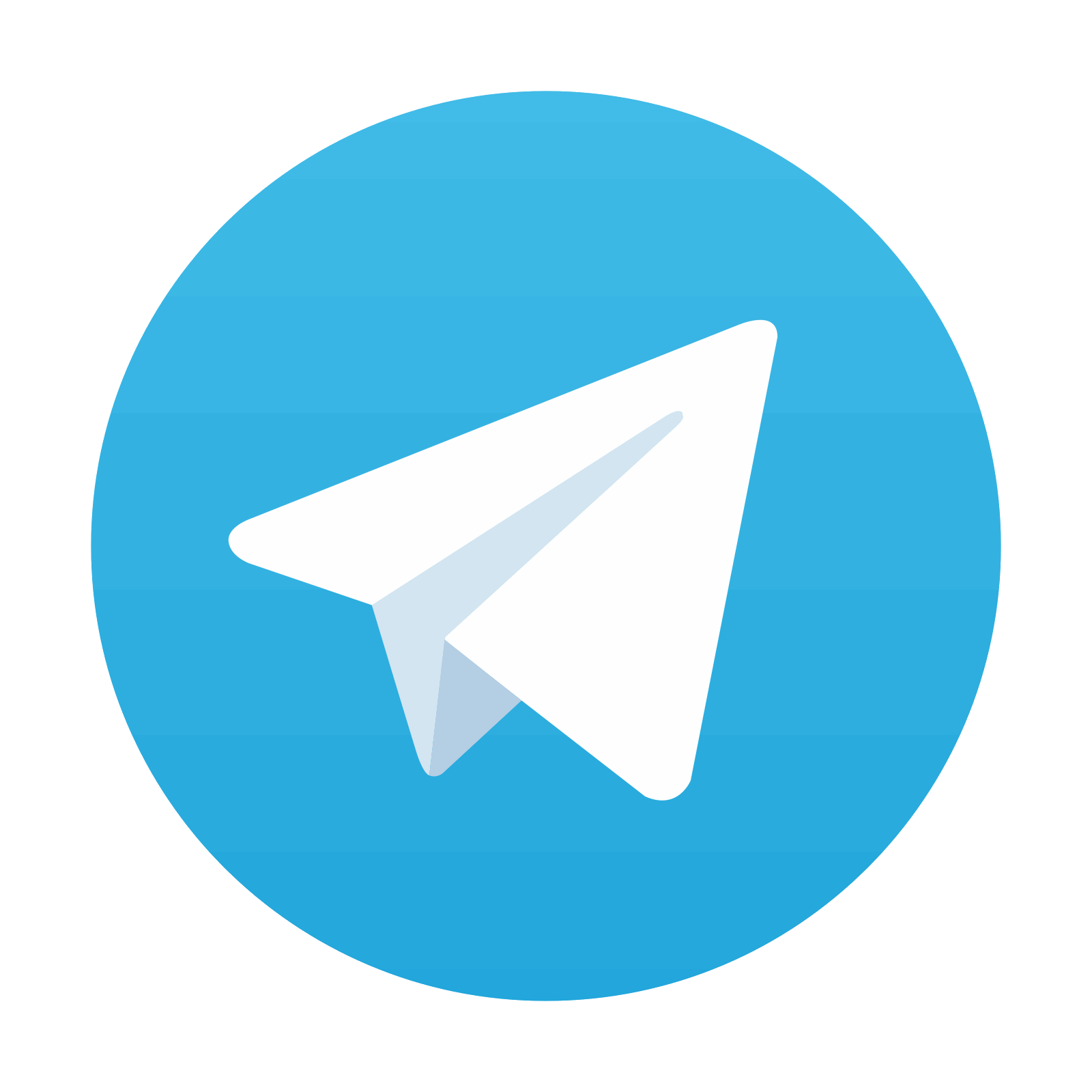
Stay updated, free articles. Join our Telegram channel

Full access? Get Clinical Tree
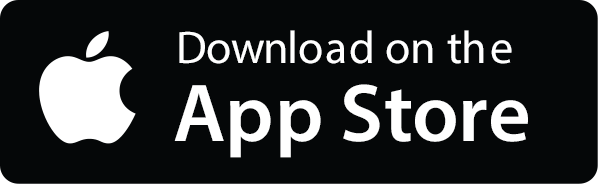
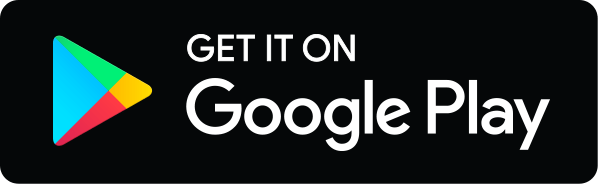