Computed tomographic colonography (CTC) has emerged as an alternative screening tool for colorectal cancer due to the potential to provide good efficacy combined with greater acceptability than optical colonoscopy or fecal occult blood testing. However, some organizations have raised concerns about the potential harms, including perforation rates and radiation-related cancer risks, and have not recommended that it currently be used as a screening tool in the general population in the US. In this article the authors review the current evidence for these potential harms from CTC and compare them to the potential harms from the alternatives including colonoscopy and double-contrast barium enema.
Colorectal cancer is a common cancer that can be detected in advance by screening. Unfortunately screening uptake is relatively low, partly because the acceptability of the available screening tools, such as optical colonoscopy and fecal occult blood testing, is low. Computed tomographic colonography (CTC) has emerged as a potential screening tool that may provide good efficacy combined with greater acceptability. Despite the evidence that it can be as sensitive as optical colonoscopy for large polyp and cancer detection, it is not yet recommended as a routine screening tool by all organizations in the United States. The American Cancer Society recently added CTC to its list of recommended screening tools for colorectal cancer. However, concerns were raised by the US Preventive Services Task Force and Medicare about the potential harms, including perforation rates, radiation doses, and the risk of radiation-related cancer. In this article, the authors review the current evidence for these potential harms from CTC and compare them to the potential harms from the alternatives, including colonoscopy and double-contrast barium enema. The related issue of the consequences of identifying extracolonic findings, including anxiety and additional testing, (see article by Yee and colleagues elsewhere in this issue for further exploration of this topic).
Colonic perforation
Perforation of the colon is an exceedingly uncommon complication of CTC. An advantage of CTC is that unlike colonoscopy, it does not require the insertion and maneuvering of an endoscope to the cecum. Instead, the patient undergoes gas insufflation of the colon using a small rectal tube, which is considered to be safer than colonoscopy. In addition, patients are sedated for their colonoscopy procedure, whereas patients who undergo CTC are able to avoid the cardiopulmonary risks of sedation because they remain awake during the scanning and are also able to be immediately assessed for any signs and symptoms of overdistention of the colon or possible perforation.
The first reports of colonic perforation related to CTC were published in 2004, about 10 years after the introduction of the technique. Perforation generally occurred in patients who had known colonic pathology. Kamar and colleagues reported a case of rectal perforation in a patient with a large obstructive rectosigmoid carcinoma. An intrarectal balloon had been inflated in the patient, and the perforation was thought to be related to manual overinflation of the rectum with room air in the presence of an obstructing mass. Two other instances of perforation related to CTC occurred in patients with active inflammatory bowel disease. Coady-Fariborzian and colleagues reported a patient with long-standing steroid-dependent ulcerative colitis who developed cecal perforation during CTC. Triester and colleagues reported colonic perforation during CTC in a patient with active fibrostenosing Crohn disease. The patient had multiple strictures in the rectum and sigmoid colon and at an ileoascending colon anastomosis, which placed her at high risk for possible perforation.
A national survey performed at 50 sites in the United Kingdom evaluated adverse events of CTC in 17,067 patients who had symptoms of possible colorectal cancer. There were 9 perforations reported occurring at 6 centers for an overall perforation rate of 0.05%. However, for 5 of the 9 patients, perforations had identifiable causes, such as insufflation of a rectal stump; forced placement of a rectal catheter; ulcerative colitis; obstructive sigmoid carcinoma; and perforation before CTC, which was thought to be related to the bowel preparation. Four of 9 patients were entirely asymptomatic, and extraluminal gas was noted on the computed tomographic (CT) scan between 6 hours and 4 hours after the procedure. All 4 patients had already returned home, did not have any clinical symptoms of perforation when contacted, and were treated conservatively. Thus, the symptomatic perforation rate from this study was 0.03%. The investigators note that the symptomatic perforation rate is the clinically relevant perforation rate that should be used for comparison with other types of colon studies because CTC inherently detects even tiny amounts of free air that would otherwise not be detected by other examinations such as colonoscopy, sigmoidoscopy, or barium enema.
A retrospective study assessed the risk of perforation from CTC in 11,870 patients at 11 centers in Israel. There were 7 cases of colon perforation occurring at 5 sites, yielding a total perforation rate of 0.06%. Four cases of perforation occurred in patients referred for CTC after incomplete colonoscopy. The mean age of patients in this study was high at 78 years. All studies were performed after room air insufflation, and an intrarectal balloon was inflated in 6 of 7 cases of perforation. Surgery was performed in 4 of 7 patients with colon perforation, and 3 patients were treated conservatively for a symptomatic perforation rate of 0.03%. Risk factors for perforation that were determined in this study included severe diverticulosis in 3 patients, obstructive carcinoma in 1 patient, and left inguinal hernia trapping sigmoid colon in 4 patients with some diseases occurring concomitantly.
The largest study to date was a survey of 16 medical centers across 5 countries (Working Group on Virtual Colonoscopy) conducted to evaluate the symptomatic perforation rate and the overall significant complication rate of CTC. A total of 21,923 CTC examinations were included, with about half of the cases performed for screening and the other half being diagnostic cases. Two perforations were reported in patients undergoing diagnostic CTC. Manual room air insufflation was used in the 2 patients. One patient had a known annular sigmoid carcinoma and was symptomatic before the CTC. One patient was asymptomatic and did not require hospitalization or any treatment for the perforation. The overall perforation rate was 0.009% (2 of 21,923), and the symptomatic perforation rate was determined to be 0.005% (1 of 21,923). It was concluded that the safety profile for CTC is favorable, particularly for screening purposes.
In comparing the published series of perforations occurring during CTC, the symptomatic perforation rate has been found to range from 0.005% to 0.03%. These rates impart a significantly more favorable safety profile for CTC compared with colonoscopy. Most patients who have perforations during CTC have had conditions that likely contributed to or exacerbated barotrauma to the bowel wall during insufflation of the colon. Obstructive or occlusive lesions, such as those from carcinoma, diverticulosis, benign inflammatory stricture, and left inguinal hernia containing sigmoid colon, have been found to be associated with perforation. Specific attention is recommended to the left groin in patients with known preexisting left inguinal hernia during the insufflation process of CTC. Insufflation is discontinued if there is increase in size of the hernia sac. Active inflammatory bowel diseases, such as ulcerative colitis, with weakening of the bowel wall can increase the risk for perforation. Other causes of weakening of the colon wall such as a colonoscopic biopsy or polypectomy may increase the likelihood of perforation during CTC. In some sites, performing CTC is postponed in this situation until 1 to 2 weeks after the biopsy. The inflation of a balloon-tipped catheter may increase the likelihood of rectal perforations, and the catheter is recommended to be used with caution by appropriately trained personnel. There are also reports of colonic perforation during CTC in patients without known colonic disease. These perforations occurred in elderly patients who were managed conservatively with antibiotic treatment.
Colonic perforation is a known risk of conventional colonoscopy screening and occurs in 0.06% to 0.19% of cases. The perforation rate is approximately 0.1% for diagnostic colonoscopy and 0.2% for therapeutic colonoscopy in larger series. Thus, perforation rates for diagnostic colonoscopy are significantly higher than for CTC. However, positive findings from CTC screening typically require a subsequent colonoscopy, and, therefore, the total perforation rate for the combination of procedures is somewhat higher than the rate for the CTC alone. Mechanical trauma caused by the colonoscope is thought to be the primary cause of perforation. The sigmoid colon is a common site for perforation during colonoscopy. Diverticulosis typically occurs in the sigmoid, which is also often redundant and tortuous and which may be more fixed if pelvic adhesions are present. High pneumatic pressure during insufflation of the colon can contribute to an increased risk for perforation during colonoscopy. The risk of perforation also increases during a therapeutic colonoscopy when polypectomy is performed. Colonoscopic biopsy, particularly when deep, is also a risk factor for perforation of the colon. The reported mortality rate for colonoscopy ranges from 0% to 0.07%. To date there have been no deaths associated with the performance of CTC.
The double-contrast barium enema has been reported to have a risk of perforation ranging between 0.004% and 0.2%. The complications related to colon perforation caused by barium enema are thought to be more clinically significant than those that occur during CTC. In one study, 10% of patients who suffered colon perforation from barium enema died of barium peritonitis. Perforations occurring as a result of barium enema are typically due to trauma from insertion of the rectal tube or overinflation of the intrarectal retention balloon. Perforation during barium enema may also occur if the procedure is performed in patients with a weakened colon wall, such as caused by ischemia, steroid use, or a deep colonic biopsy. Similar to CTC, to decrease the likelihood of perforation during barium enema, it is recommended to wait for at least 1 week after biopsy.
Radiation dose and cancer risk
Several recent studies have raised concerns about the radiation-related cancer risks from CT scans. The typical radiation doses from CTC, the sensitivity and specificity of low-dose CTC, the potential radiation-related cancer risks from the screening, and the follow-up examinations for extracolonic findings are discussed.
Radiation Dose
Most medical examinations that involve ionizing radiation only expose certain parts of the body to radiation,which is referred to as partial body exposure. Absorbed organ doses and effective doses are the key quantities used to summarize doses from such partial body exposures. Here the authors briefly describe these quantities, estimation methods, and sample dose estimates for CTC screening.
The amount of radiation energy that is absorbed per gram of tissue is called the absorbed dose. It is measured in grays (Gy) whereby 1 Gy corresponds to 1 joule of energy absorbed per kilogram. The biologic effect per unit of absorbed dose depends on the particular tissue that is exposed, as some tissues have been shown to be more sensitive to radiation than others. For example, a dose given to the breast is more likely to cause cancer than the same dose given to the rectum. To compare doses from different tests involving partial body exposure, the effective dose is typically used. The effective dose is a weighted average of the absorbed doses to each exposed organ with weights that reflect the relative radiosensitivity of each organ.
Radiation dose from CT scanning depends on patient size, CT scanner model, and protocol. For the same CT scanner and the same protocol, patients with smaller body size (eg, pediatric patients) receive higher radiation doses because there is less tissue to attenuate the radiation before it reaches a particular organ. Even for the same CT scanner model, the protocol used may vary between hospitals or technicians. The operational factors specified in the protocol include number of scans, scan length, image thickness, degree of overlap between adjacent x-ray beams (pitch or table feed), tube current-time product (mAs), and tube potential (kVp).
There are several operational factors that typically result in higher doses. Repeated CT scanning, such as multiphase examinations, increases the radiation dose. For example, 2 sets of images are obtained during CTC, with the patient first in the supine position and then in the prone position, and so the radiation dose is typically doubled. Radiation dose also increases rapidly with the beam potential (kVp): as a rule of thumb, by a power of 2.5. A longer scan length results in radiation exposure to a greater anatomic region and hence a higher radiation dose. Thinner images provide better image resolution and improved visibility of small objects. However, beam intensity needs to be increased to reduce the noise in these thinner images, which concurrently increases the radiation dose. CT scan images can be obtained in contiguity, as well as with an overlap, or a gap between x-ray beams. The degree of the overlap or the gap between the beams is expressed by pitch. A pitch of less than 1 indicates overlapped beam exposure. Therefore, radiation dose is inversely proportional to pitch. Tube current-time product (mAs) is the product of tube current (mA) and gantry rotation time (sec), and radiation dose is linearly proportional to mAs. In general, the key factors that are modified to reduce the radiation exposure from a CT scan are the tube current and the beam potential.
Because there are multiple factors in the CT protocol that influence radiation exposure in different directions, special software programs have been developed to estimate organ-specific and effective doses from CT scans for a user-defined input involving CT scan parameters and scanner type (eg, CT Expo and ImPACT ). These programs use preestablished organ dose databases generated by radiation transport computer simulations, which simulate radiation interactions in the human body. The patient is assumed to be a “standard man,” who is defined as measuring 170 cm in height and 70 kg in weight. These programs are useful for comparing doses across protocols or scanners but cannot be used to estimate the dose to a specific individual.
Since the introduction of CTC using single-detector CT scanners, there has been the development of multidetector CT scanners with incorporation of low-dose CT techniques. The 2009 American College of Radiology practice guideline for the performance of CTC in adults specifies that screening studies that are performed in individuals without signs or symptoms of colorectal cancer should be performed using a low-dose, nonenhanced multidetector CT technique. The use of technique charts or automatic exposure control is suggested so that patient size may be accounted for. The CT protocol used also varies depending on the clinical indication for the test. Although there are parameters that may be specific to particular CT scanners, in general for a tube potential of 120 kVp, an effective mAs of between 50 and 80 is recommended when performing screening CTC (low-dose CTC). If diagnostic CTC is being performed in a patient with symptoms of possible colorectal carcinoma, intravenous contrast may be necessary and CT acquisition parameters may typically require higher mAs. The performance characteristics of low-dose CTC are reviewed in detail below.
Sensitivity and Specificity of Low-Dose CTC
Early studies have shown that tube current may be decreased to reduce radiation exposure without compromising polyp detection on CTC. This detection is possible because of the inherent large difference in density between the air-filled lumen and the soft tissue attenuation of colonic polyps. In one such study, CTC was performed using single-detector CT in 2 patient groups. In the first group, 8 patients with 31 polyps were scanned using 140 mA, 5-mm collimation, pitch of 1.3, and 3-mm reconstruction interval. In the second group, 10 patients with 30 polyps were scanned using 70 mA, 5-mm collimation, pitch of 1, and 1-mm reconstruction interval. There was 100% sensitivity for detection of polyps of size 5 mm and larger for both groups. The radiation dose for dual position CTC at 70 mA was determined to be 50% lower than the dose for a standard CT scan of the abdomen and pelvis and similar to the radiation dose for a barium enema.
A study evaluating multidetector CTC at various radiation dose levels was performed in 50 patients at high risk for the development of colorectal carcinoma and showed no compromise in polyp detection ability with lower-dose protocols. Patients were scanned using 120 kVp and 100 mAs. Two low-dose CT scans were then obtained by simulating 50 mAs and 30 mAs. Although there was overall decreased image quality at 30 mAs, the per-patient sensitivity for the detection of polyps measuring 5 mm and larger was 90% at all dose levels. Similarly, the per-polyp sensitivity for detecting polyps measuring 5 mm and larger ranged between 85% and 92% for all doses.
Low-dose thin-section multidetector CTC, followed by conventional colonoscopy, was performed in 105 patients who had symptoms of possible colorectal cancer. CT scan parameters included 120 kVp, 50 mAs, and thin sections with 1.25-mm reconstructions at a 1.0-mm interval. The per-lesion sensitivities for detection of polyps of size 6 to 9 mm and 10 mm and larger were 70% and 93%, respectively. Overall specificity was excellent at 98%. The effective doses for dual position CTC were 5.0 mSv for men and 7.8 mSv for women. This study demonstrated that low-dose multidetector CTC has excellent sensitivity and specificity for the detection of large polyps.
Most recently, low-dose CTC technique has also been evaluated successfully in average-risk patients without symptoms of colorectal disease. A performance trial using the newest 64-slice multidetector CT was conducted in 307 subjects who underwent CTC for screening. Patients were scanned using a tube voltage of 120 kVp and a tube current of 70 mAs in the supine position, which was decreased to 30 mAs in the prone position. A new dose-modulation technique was used to adapt the tube current automatically to patient anatomy. The calculated mean radiation dose to patients was 4.5 mSv for the entire examination, which is significantly reduced from earlier studies. The sensitivity and specificity of CTC for the detection of adenomatous polyps larger than 5 mm were 91% and 93%, respectively, and for adenomatous polyps larger than 10 mm were 92% and 98%, respectively.
Several studies have evaluated the potential for decreasing the radiation dose for CTC even further. A pilot study reported excellent results with very low-dose CTC in 27 patients with symptoms of possible colorectal cancer. Multidetector CT was performed with 140 kVp and 10 mAs. All 9 cancers were identified, and there was 100% sensitivity for polyps 10 mm and larger (3 of 3) as well as for polyps between 6 and 9 mm (3 of 3). Total radiation dose for dual position CTC was 1.7 mSv for men and 2.3 mSv for women, which was a 40% to 70% decrease in radiation dose compared with prior studies. A follow-up study using the same lower-dose CTC protocol was performed in 158 patients and revealed similar excellent results. CTC performed with 10 mAs resulted in 100% sensitivity for carcinomas (22 of 22), 100% sensitivity for large polyps (13 of 13), and 83% sensitivity for small polyps (20 of 24). There was 97% specificity, and the positive and negative predictive values were 94% and 98%, respectively. The simulated effective doses for combined supine and prone acquisitions were 1.8 mSv in men and 2.4 mSv in women. CTC using effective mAs of 10 was performed in 88 patients who underwent 2 sequential colonoscopies with results of the second colonoscopy serving as the reference standard for determination of the performance of the CTC and the first colonoscopy. The per-polyp sensitivities for lesions 6 mm and larger for CTC and the initial colonoscopy were 86% and 84%, respectively. The initial colonoscopy missed 16 polyps, 6 of which were identified by low-dose CT. The per-patient sensitivities for polyps of size 6 mm and larger for CTC and the first colonoscopy were 84% and 90%, respectively, with specificities of 82% and 100%, respectively.
An alternative approach that has been proposed for dose reduction is to scan patients in only 1 position. However, it is not clear if scanning in 1 position will provide adequate colonic cleansing and distention, given that dual position scanning typically allows for shifting of residual material in the colon and improved distention. In a study evaluating low-dose CTC, 137 patients were scanned only in the supine position. CT scan parameters included 120 kVp and effective mAs of 10. The effective dose using this type of protocol was 0.7 mSv for men and 1 mSv for women. The sensitivities for detection of polyps larger than 10 mm, between 5 and 9.9 mm, and less than 5 mm in size were 78.6%, 85.7%, and 57%, respectively, with the specificity for each size group at 100%, 92.8%, and 85.9%, respectively. It was concluded that low-dose CTC is feasible with substantial radiation dose reduction, but additional studies are required to evaluate whether there is a compromise in small poly detection ability when scanning in 1 position. Current standard practice consists of scanning patients in supine and in prone positions.
There have been other efforts to determine whether radiation dose could be lowered even more. A pilot study was performed in 15 patients who were scanned using different mAs levels ranging between 0.4 mAs and 100 mAs correlating with doses ranging from 0.05 mSv to 12 mSv. Sensitivity was 80% to 100% for the detection of large polyps (>10 mm) for all mAs and dose levels. The mean sensitivity for identifying polyps of size 5 mm and larger was 74% across all mAs and dose levels except for the lowest level of 0.4 mAs. The number of false-positive results decreased as the mAs decreased for 5-mm and larger lesions. This decrease was thought to be due to increased noise and smoothing algorithms applied to the lowest-dose images. Continued investigation is needed to explore the clinical value of very low–dose CTC.
A recent international survey of 34 institutions aimed to evaluate what protocols were actually being used in practice at present. Based on the institutions’ protocols, the estimated effective dose for CTC screening ranged from 2.6 mSv to 14.7 mSv per examination and the median dose was 5.6 mSv. The largest source of variation in the protocols was the mAs, which varied from 25 to 100 mAs for the supine scan. These survey results suggest that low-dose protocols are being used in many different countries, but there is still wide variation in practice. The effective dose from a double-contrast barium enema is of a similar magnitude to that from CTC, but also highly variable. A recent literature review found that the average dose per procedure was 8 mSv but with a range of 2 to 18 mSv.
Radiation-Related Cancer Risk
Despite the fact that low-dose protocols are being used routinely and have shown excellent sensitivity and specificity for lesion detection, there are still concerns about the potential cancer risks. Numerous epidemiologic studies have established that radiation can cause most types of cancer and that there is unlikely to be a threshold below which there is no risk. The study of the Japanese atomic bomb survivors in Nagasaki and Hiroshima, known as the Life Span Study, is the most important study of the long-term effects of radiation exposure. The key strengths are its large size (N ≈ 120,000), long-term follow-up (>50 years), and the fact that the population was not selected because of a specific disease or an occupation and therefore includes a wide range of ages of healthy men and women who were exposed to a broad range of radiation doses (0–4 Gy). A common misconception about the Life Span Study is that it is a study of high-dose radiation exposures. The median dose in the exposed population is 250 mGy, and about 25% of the population had exposures in the range of 5 to 100 mGy. For solid cancers the risk is approximately linear in dose, whereas for leukemia the dose-response is curvilinear so that the risk per unit dose is lower at lower dose levels. There is also evidence of a significantly increased cancer risk in the 0- to 150-mGy dose range, and the magnitude of the risk per unit dose is similar to the risk across the whole dose range. Risks are generally found to be higher if exposure occurs at younger ages, and after exposure there seems to be a lifelong elevation in cancer risk.
Other populations that have been studied for the long-term effects of radiation include those exposed for medical reasons (both therapeutic and diagnostic), and occupational groups such as radiologists and nuclear power workers. These studies also contribute important information on the risks from repeated low-dose radiation exposures, as opposed to the acute single exposure that was received in the Japanese atomic bombing. Although they provide additional evidence of significantly elevated cancer risks for low doses of radiation (<100 mGy), most studies do not have adequate power to enable precise risk estimates at these low dose levels, especially taking into account factors such as age at exposure and site of cancer. Therefore, risks from low doses are generally estimated by extrapolating models based on the results from the whole range of exposures received in the Life Span Study. Such risk projection methods based on existing data can provide a much more timely estimate of the long-term risk of radiation-related cancer.
A recent committee for the National Research Council (the Biological Effects of Ionizing Radiation [BEIR] VII committee) developed detailed models for estimating the lifetime risk of radiation-related cancer for the US population. These risk models are based primarily on the most recent follow-up of the Japanese atomic bomb survivors because for most cancer sites, they remain the most detailed models available for estimating the risks by sex, age, and time since exposure. These risk models, with minor modifications (mainly the addition of cancers of several sites, such as pancreatic, rectal, kidney, esophageal, oral, and brain cancers), combined with the organ-specific dose estimates (described later in this article) have been used to estimate the lifetime risk of radiation-related cancer after CTC screening.
Whereas effective dose estimates are useful for comparing doses from different protocols, cancer risk estimation is best performed with organ dose estimates. Because there are no recently published estimates of organ-specific doses from CTC in the United States, the authors used CT-Expo and a CTC screening protocol from the recent American College of Radiology Imaging Network (ACRIN) trial for the LightSpeed 64-slice scanner (GE Healthcare, Waukesha, WI, USA) (120 kVp, 50 mAs, pitch of 1, and image thickness of 1.25 mm ) to estimate organ doses for a typical screening examination in the United States. Organ dose estimates varied from 14 mGy to the stomach to 2 mGy to the lung for men and from 15 mGy to the kidney to 1 mGy to the breast for women. The effective dose per screening examination was 8 mSv for a man and 9 mSv for a woman. The scanner that the authors used for these calculations is one that is commonly used in the United States, and the protocol is in line with the recommendations for CTC screening from the American College of Radiology.
The authors estimated that a single CTC screen at age 60 years would result in a lifetime risk of radiation-related cancer of approximately 0.05% (ie, 5 cancers per 10,000 individuals screened). The risks were similar for men and women. For a single screen at age 50 years, the risks were slightly higher (0.06%) and at age 70 years were lower (0.03%), primarily because of the longer versus shorter life expectancy. If an individual undergoes multiple screens, for example, screening every 5 years from age 60 to 75 years, then the total risk would be approximately 0.016% (2 × 0.05% + 2 × 0.03%). Because the effective dose estimates for barium enema are broadly similar to those for CTC, the radiation-related cancer risks will also be similar.
Previous estimates of the radiation-related cancer risk from CTC screening were approximately twice as high, even though they were based on similar organ doses (0.14% for a single screen at age 50 years compared with 0.06%). The difference between these risk estimates was mostly due to the assumptions underlying the radiation risk models. The previous study used the BEIR V committee’s risk models from a report published in 1990. The main change between the 2 reports was the assumption of how risks are transported from the Japanese to the US population. The BEIR VII report provides an in-depth description of the new data and justification for these alternative assumptions. Most other national and international committees that provide risk projection estimates currently use similar methods to those used in the BEIR VII report (eg, United Nations Scientific Committee on the Effects of Atomic Radiation [UNSCEAR] ). There are several uncertainties and assumptions that go into the estimation, and it is not unreasonable to assume that the risks may vary by a factor of 2. Therefore, the previous estimates of 0.14% for a screen at age 50 years could be taken as approximate upper bounds for the potential risk.
Unlike colonoscopy, the whole abdomen is visible during CTC screening. Potential abnormalities outside of the colon can therefore be picked up. Many US screening studies have collected data on the number of patients who had clinically significant extracolonic findings that required further imaging. The proportion of patients who had follow-up CT scans to investigate these findings was generally in the range of 5% to 10% ; in one small study it was 24%. The most common follow-up scan was a CT scan of the abdomen. Abdomen/pelvis and chest CT scans were also performed. The dose from an abdomen/pelvis CT scan performed with and without contrast is about 20 mSv, which results in a radiation risk that is about twice as high as the risk from CTC. However, because only a small proportion (eg, 10%) of the screening population will receive these additional scans, it is unlikely that these scans will increase the average risk to the whole screening population by more than 20%.
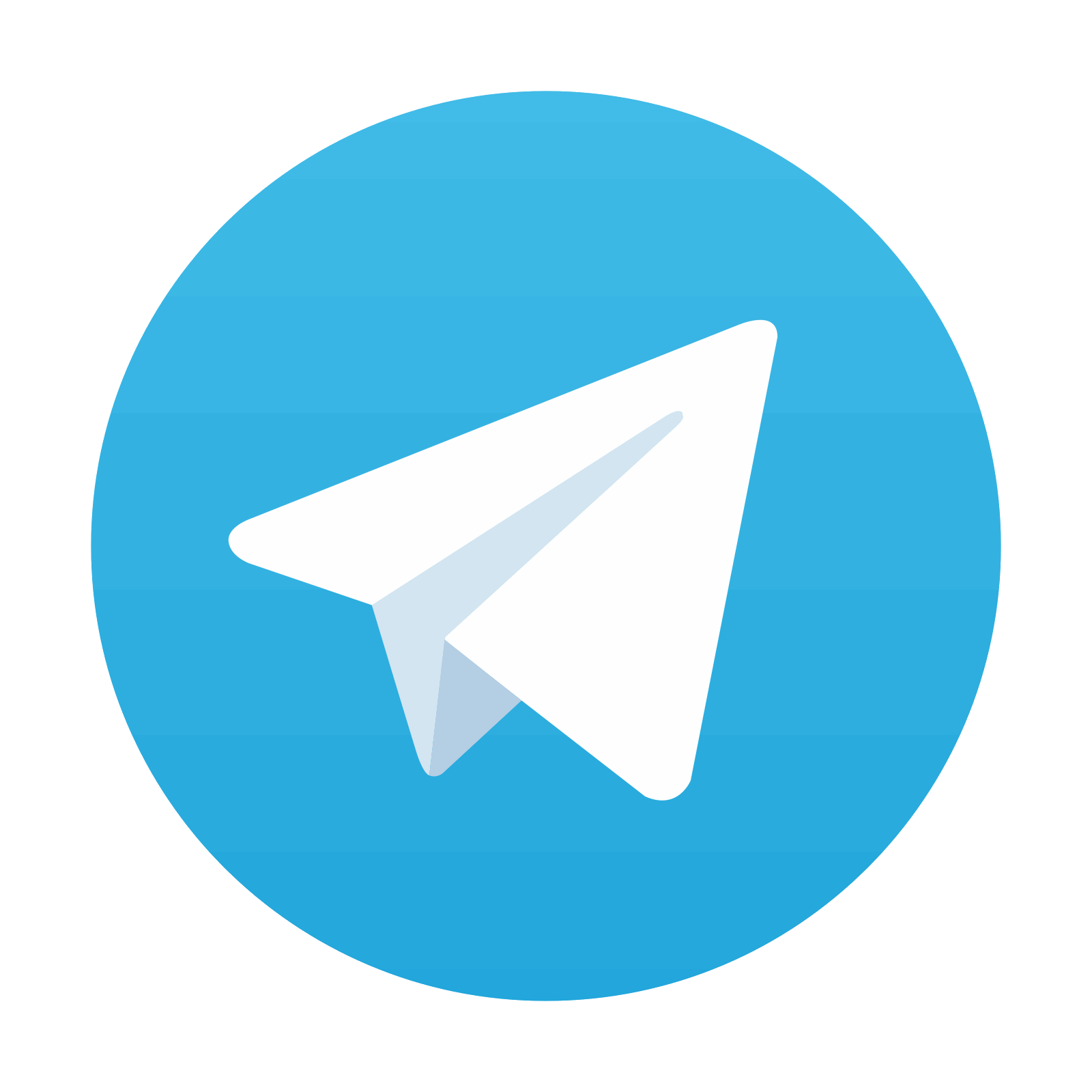
Stay updated, free articles. Join our Telegram channel

Full access? Get Clinical Tree
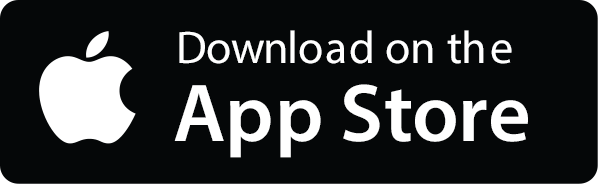
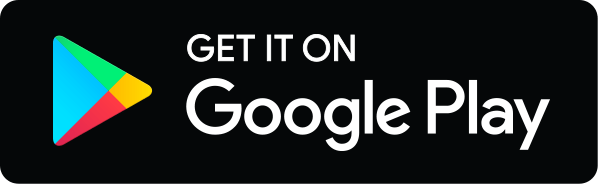