© Springer International Publishing Switzerland 2017
Eduardo de Santibañes, Victoria Ardiles, Fernando A. Alvarez, Virginia Cano Busnelli and Martin de Santibañes (eds.)Extreme Hepatic Surgery and Other Strategieshttps://doi.org/10.1007/978-3-319-13896-1_66. Conversion and Neoadjuvant Therapies
(1)
Department of Surgery, Sanatorio San Lucas, San Isidro, Buenos Aires, Argentina
Keywords
ChemotherapyChemotherapeutic agentsDisappearing liver metastasesNeoadjuvant chemotherapyConversion chemotherapyAdjuvant chemotherapyColorectal liver metastasesIntroduction
We define, as metastatic colorectal cancer (CRC), all cases of adenocarcinoma of the colon and/or rectum with documented secondary sites at the moment of diagnosis. The liver is the most common site for CLMs due to the enteric venous drainage via the portal circulation, with up to 50% of CRC patients developing liver metastases. Furthermore, the liver is the only site of metastasis in 35% of cases [1]. Globally, CRC is the commonest malignancy of the digestive tract and the third most common type of cancer, making up about 10% of all cases. It is more common in developed countries, where more than 65% of cases are found, and it is slightly less common in women than men [2, 3]. The majority of CRC develop sporadically; however, family history of CRC in a first-degree relative confers a two- to three-fold increased risk of disease. Environmental risk factors include obesity, physical inactivity, low fibre diet, and a high intake of red and processed meats [3]. The International Agency for Research on Cancer, the GLOBOCAN 2012, estimated the cancer incidence, mortality, and prevalence worldwide in 2012, and has published incidence and mortality of 27 major cancers for that year. They have reviewed the sources and methods used in compiling the national cancer incidence and mortality in 20 large “areas” of the world. Overall, there were 14.1 million new cases and 8.2 million deaths in 2012 [4]. The most commonly diagnosed cancers were lung (1.82 million), breast (1.67 million), and colorectal (1.36 million); the most common causes of cancer death were lung cancer (1.6 million deaths), liver cancer (745,000 deaths), and stomach cancer (723,000 deaths) [4]. For instance, GLOBOCAN 2012 published that the number of new CRC cases in all ages and both sexes in United States would be144,309 in 2015 (75,157 men and 69,152 women), and the number of deaths in all ages and both sexes would be 59,283 (31,434 men and 27,849 women) [4]. In our country, Argentina, the number of new CRC cases in all ages and both sexes would be 14,295 in 2015 (7626 men and 6669 women), and the number of deaths in all ages and both sexes would be 8391 (4537 men and 3854 women) [1]. In United States and in Argentina, CRC is the third most common cancer in both men and women and, the third leading cause in both sexes of cancer death in men and women [3]. Each year, the American Cancer Society estimates the numbers of new cancer cases and deaths that will occur in the United States in the current year and compiles the most recent data on cancer incidence, mortality, and survival [2]. The overall cancer death rate in the Unites States decreased from 2151 (per 100,000 population) in 1991 to 1687 in 2011, a total relative decline of 22%. However, the magnitude of the decline varied by state of the country [2, 3]. CRC incidences rates also have been decreasing in United States for most of the past two decades, which has been attributed to both changes in risk factors and the uptake of CRC screening among adults 50 years and older. Further, from 2007 to 2011, incidence rates declined by 4.3% per year among adults 50 years of age and older, but increased by 1.8% per year among adults younger than age 50 [5]. CRC mortality rates have been declining since 1980 in men and since 1947 in women, with the decline accelerating in both sexes in the most recent time period. In addition, from 2007 to 2011, the overall death rate declined by 2.5% per year. This trend reflects declining incidence rates and improvements in early detection and treatment [5]. Treatments used for CRC may include some combination of surgery, radiation therapy, chemotherapy, and targeted therapy. Tumors that are confined within the wall of the colon may be curable with surgery, while cancer that has spread widely is usually not curable, with management focusing on improving quality of life and symptoms. Five-year survival rates in Western countries are around 60–65%. This, however, depends on how advanced the CRC is, whether or not all the cancer can be removed with surgery, and on the person’s overall health. It is well known that the liver is the most common site of metastases from CRC [3, 4]. Approximately 15–20% of patients have liver metastases at diagnosis, and another 50% develop metastatic disease to the liver over the course of their disease [4–7]. For these patients, hepatic resection currently offers the best chance for long-term survival [6–9]. Related to the way of referring to disease-free survival versus progression-free survival, currently, there are trends in medical literature to change the word “disease” to “progression” because the first one has the wrong connotation of surviving without cancer disease, but the true situation is that the only way we can know whether the patient has a progression of disease or not is through imaging studies and/or blood tumor marker levels. Based on current evidence, the primary aim of treatment is to achieve a long progression-free survival (PFS) interval following resection. Whether or not initially resectable or unresectable CLMs are cured or at least whether a long overall survival (OS) duration is possible after complete resection of the metastases has improved significantly in recent years [6–8]. Although the reasons for this OS trend are not clear, contributing factors may include the use of newer preoperative imaging studies, increased use of chemotherapy, and salvage surgical therapy [9]. Adam, R and colleagues published in 2014 the results of “LiverMetSurvey” related to patient survival after a first liver operation for CLMs in a cohort of 23,444 cases coming from 302 surgical centers of 69 countries. A total of 22,210 were resected, and the other 1234 were not resected. The first group had 41% and 24% of OS at 5 and 10 years respectively, whereas the second group had only 9% at 5-year survival [7]. Advances in surgical and medical treatments have significantly changed the management of CLMs. In particular, new drugs and modern combination chemotherapy regimens, together with the improvement of surgical techniques, allow a potentially curative approach in an increasing number of patients. Nevertheless, there is no strong evidence for an optimal treatment strategy for CLMs, mainly because of the extensive heterogeneity in the patients [10]. In fact, in our opinion we believe that patients with CLMs are not all a unique population, because they are integrated by diverse clinical and biological subtypes that require different approaches. Moreover, the results of many published studies in this setting may be difficult to interpret, partly because the definitions of the various subgroups of patients are unclear and have overlapping between them.
Chemotherapeutic Agents: Overview and Toxicity
Early studies In the 1980s and 1990s, highlighting the publications of Adson, M and colleagues in 1984; Sugihara, K and colleagues in 1993 and Fong, Y and colleagues in 1997, had reported 5-year survival rates of 25–48% following complete resection of the CLMs, in an era when the only active chemotherapeutic agent used was the antimetabolite 5-fluorouracil [11–13]. With increased use of systemic preoperative chemotherapy (SPC) for CLMs, clinical oncologists and liver surgeons are becoming more aware of the toxic effects of chemotherapy on liver parenchyma and how these changes affect postoperative outcome [14]. The entity “chemotherapy-associated liver injury or CALI” is described as the hepatotoxic effects of chemotherapy agents on the non-tumoral liver parenchyma. Three types of CALI are recognized: steatosis, steatohepatitis, and sinusoidal obstruction syndrome [15]. SPC alters the liver parenchyma in such way that it may increase the risks of liver resection [16]. Many studies have been published to assess the effects of SPC on the morphology of non-tumoral liver, which could produce histologic changes in the histology of the liver that may impact on surgical outcomes [17]. Pathologists have shown a wide spectrum of histopathological changes to the underlying liver parenchyma in resection specimens. The importance of CALI has been highlighted with regard to preoperative, operative, or early postoperative period [16]. Postoperative morbidity is correlated with the number of cycles of SPC administered before surgery, but not specifically due to the type of chemotherapy [16–18]. CALI can in particular prolong surgery and hospitalization, decrease accuracy of metastases at time of preoperative imaging assessment, increase risk of perioperative hemorrhage, postoperative risk for infections, lead to liver failure after major hepatectomy, cause portal hypertension with splenomegaly and ascites, and be responsible for persistent thrombocytopenia [18, 19]. It is mandatory that liver surgeons should have an adequate knowledge of the chemotherapy-associated hepatotoxicity [20]. However, with appropriate patient selection, liver resection for CLMs can be safely performed in patients treated with SPC [21]. Even though the association between chemotherapy and histopathologic changes has been well documented, the impact of chemotherapy-associated hepatotoxicity on surgical management of CLMs remains somewhat ill-defined. Many authors have concluded that the risk for postoperative complications in surgery of CLMs is related to the duration of SPC administration [14–16, 21–24]. Although the optimal regimen and duration of SPC is still being assessed, dialogue between clinical oncologists and liver surgeons regarding the timing of surgery is critical. Theoretically, a longer interval may provide the liver time to recover from any reversible hepatotoxic effect of chemotherapy. But on the other hand, a longer interval prior to hepatic resection may result in progression of disease. With this in mind, most liver surgeons will proceed with resection between 4 and 6 weeks after the last dose of chemotherapy [21–23]. SPC for CLMs induces regimen-specific hepatic changes that can affect patient outcome. Both response rate and toxicity should be considered when selecting preoperative chemotherapy in patients with CLMs [25].
Fluorouracil or 5-FU is a pyrimidine analog which is used in the treatment of cancer. It is a suicide inhibitor and works through irreversible inhibition of thymidylate synthase. It belongs to the family of so-called antimetabolite drugs [26]. 5-FU has remained as the backbone of systemic chemotherapy for CLMs for decades. This drug inhibits thymidylate synthase, thereby lowering production of pyrimidine thymidine for DNA synthesis.
Folinic acid or leucovorin (LV) is generally administered as calcium or sodium folinate (or leucovorin calcium/sodium). Folinic acid, also called 5-formyltetrahydrofolate, was first discovered in 1948 as a citrovorum factor and occasionally is still called by that name. Folinic acid should be distinguished from folic acid (vitamin B9). However, folinic acid is a vitamer (any combination of substances that together function as a vitamin) for folic acid, and has the full vitamin activity of it. LV is an adjuvant used in cancer chemotherapy involving the drug methotrexate. It is also used in synergistic combination with the chemotherapy agent 5-FU [27]. An updated meta-analysis published by Thirion, P and colleagues in 2004 demonstrated, on a large group of patients with advanced CRC, that infusional 5-FU and LV improves both overall response rate (ORR) and OS compared with 5-FU alone, and that this benefit is consistent across various prognostic factors [28]. With the single-agent regimen of 5-FU, or 5-FU plus LV, tumor response in CLMs was seen in only 10–20% of those treated, which provided an OS of approximately a year in patients who were not candidates for an operation [27]. A multicenter study compared the therapeutic ratio of a monthly schedule of low-dose LV and 5-FU bolus with a bimonthly schedule of high-dose LV and 5-FU bolus plus continuous infusion in patients with advanced CRC. The conclusion of this study is that the bimonthly regimen was more effective and less toxic than the monthly regimen and definitely could increase the therapeutic ratio. However, there was no evidence of increased survival [29]. Adverse effects: 5-FU treatment can potentially include hepatotoxic effects on the non-tumoral liver parenchyma that have been reported as steatosis, which is a non-alcoholic fatty liver disease at the early stage and corresponds to accumulation of lipids in hepatocytes, and has a prevalence that ranges between 16–31% which may increase to 46–75% in patients with high alcohol consumption and/or obese [25]. From the transplant literature, is well known that hepatic steatosis can impair liver function and postoperative regeneration. In a study published by Kooby, D and colleagues in 2003 of 485 patients undergoing liver resections for hepatic tumors (325 steatotic livers and 160 matched controls), steatosis was found to be an independent predictor of postoperative complications on multivariate analysis (p < 0.01). Overall and infective complication rates of 62% and 43% for the marked steatosis group (n = 102) were significantly higher than in the control group, at 35% and 14% respectively (p < 0.01) [30]. Studies associating steatosis and 5-FU are mainly based on radiological evaluation; however, ultrasound sensitivity for diagnosis of steatosis is 60–94% and specificity is of 66–95%, while CT-scan sensitivity is 82% and specificity is 100%. Further, imaging does not distinguish steatosis from steatohepatitis [26]. Although 5-FU based chemotherapy may cause profound changes in liver parenchyma, it can be safely applied [31–33].
Capecitabine is the orally administered pro-drug of 5-FU, which has lower hepatotoxicity and provides similar survival rates to those of an intravenous 5-FU/LV regimen. Capecitabine was more active than 5-FU/LV in the induction of objective tumor responses, and the times to PFS and OS were at least equivalent for capecitabine compared with the 5-FU/LV arm. In addition, capecitabine also demonstrated clinically meaningful benefits over the administration of bolus 5-FU/LV in terms of tolerability [34]. Chemotherapeutic regimens that combine continuous infusion 5-FU and LV with either oxaliplatin and irinotecan yielded ORR over 50% and provided a doubling of OS time in patients with unresectable disease [35, 36].
Oxaliplatin (oxal) is a platinum-based antineoplastic agent used in cancer chemotherapy that was discovered in 1976 at Nagoya City University by Professor Yoshinori Kidani. It is a novel platinum complex used for the treatment of metastatic CRC. It gained European approval in 1996, and in 2002 was approved by the U.S. Food and Drug Administration (FDA) [37]. According to in-vivo studies, the oxal fights against CRC through non-targeted cytotoxic effects. Like other platinum compounds, its cytotoxicity is thought to result from inhibition of DNA synthesis in cells. In particular, oxal forms inter- and intra-strand cross links in DNA, which prevent DNA replication and transcription, causing cell death [38]. Oxal is mainly used for treatment of CRC, in particular, in combination with other chemotherapy agents, including the regimen that is so-called FOLFOX, which consists of infusional 5-FU, LV, and oxal [35]. In clinical studies, oxal by itself has modest activity against advanced CRC. When compared with just 5-FU and LV administered according to the de Gramont regimen, a FOLFOX4 regimen produced no significant increase in OS, but did produce an improvement in PFS, which was the primary end-point of the phase III randomized trial [35]. Different drug doses and timed regimens—FOLFOX4, FOLFOX6, and FOLFOX7—have been evaluated, but no data support the superiority of any one over another in terms of patient OS. Recently, some authors have published that FOLFOX is a feasible and safe option in patients with CLMs with severe liver dysfunction [39, 40].
Adverse effects: First of all, neurotoxicity due to oxal-based chemotherapy is their main toxicity and is focalized as a peripheral neuropathy [41]. The symptoms are progressive, involving numbness, tingling, intense pain and hypersensitivity to cold, beginning in the hands and feet and sometimes involving the arms and legs, often with deficits in proprioception. This neurotoxicity sometimes is very annoying for patients and they suffer a lot with these symptoms [41]. Further, this adverse effect can be maintained for a long time after finished the oxal-based chemotherapy [30]. Other side-effects are fatigue, nausea, vomiting, diarrhea, neutropenia (low number of a type of white blood cells), ototoxicity (occasionally hearing loss), and persistent hiccups. Further, extravasations if oxal leaks from the infusion vein may cause severe damage to the connective tissues [31]. In addition, some patients may experience an allergic reaction to platinum-containing drugs. This is more common in women. Oxal has less ototoxicity and nephrotoxicity than cisplatin and carboplatin [37]. In patients with CLMs, chemotherapy-induced hepatic injury is associated with increased splenic volume, thrombocytopenia, and decreased long-term survival. Slade, J and colleagues described in 2009 the use of oxal-based chemotherapy in six patients with stage III or IV CRC who developed evidence of non-cirrhotic portal hypertension. These patients developed complications of portal hypertension, including esophageal or hemorrhoidal varices with bleeding, splenomegaly with associated thrombocytopenia, and ascites. In each case, oxal-induced hepatic sinusoidal injury was identified as the most likely factor contributing to the development of non-cirrhotic portal hypertension [32, 33]. Simpson, A and colleagues reported in 2015 the relationship between change in splenic volume after SPC and the development of postoperative complications. The study group consisted of 80 patients who underwent resection of CLMs; half received SPC with oxal for 6 months before resection (n = 40) and the other half did not (n = 40). They concluded that the presence of CLMs and SPC is associated with higher splenic volume. Percent splenic volume increase after 6 months of chemotherapy can aid preoperative risk stratification, as it was an independent predictor of major postoperative complications [34]. Finally, splenomegaly would decrease in size over 1–3 years after end of oxal-based chemotherapy [32, 34]. Specific hepatotoxic effects on the non-tumoral liver parenchyma have been reported, and are included in the so-called “sinusoidal obstruction syndrome or SOS”, formerly veno-occlusive disease, which is a well-established complication of hematopoietic stem cell transplantation, pyrrolizidine alkaloid intoxication, and widely used chemotherapeutic agents such as oxal [42, 43]. SPC frequently causes morphological lesions that involve the hepatic microvasculature [44, 45]. Macroscopically, liver has typically a blue-red marbled appearance, commonly so-called “blue liver” [17]. SOS is the consequence of an initial toxicity to sinusoidal endothelial cells. Histologically, it is characterized by centrilobular sinusoidal dilation, often associated with erythrocyte extravasations in perisinusoidal space (hemorrhage), compatible with a rupture of sinusoidal wall. It is occasionally associated with persinusoidal fibrosis and centrilobular vein obstruction, to peliosis or development of nodular regenerative hyperplasia [46]. Sinusoidal injury has been shown to persist several months after end of chemotherapy, and fibrosis may progress [24, 33]. A case of systemic capillary leak syndrome was reported in association with oxal-based chemotherapy [31]. Rubbia-Brandt, L and colleagues showed in 2004 that 44 (51%) of the 87 post-chemotherapy liver resection specimens had sinusoidal dilatation and hemorrhage, related to rupture of the sinusoidal barrier. In contrast, the 66 livers treated by surgery alone remained normal. Light microscopy, electron microscopy, and immunohistochemistry using antibodies against endothelial cells (CD31) and hepatic stellate cells (alpha-SM actin, CRBP-1) were performed to identify the sinusoidal wall integrity [15]. The main hepatic lesion induced by preoperative oxal-based chemotherapy in patients with CLMs is vascular and not steatosis [42]. Detailed pathologic analysis has determined that the most severe vascular lesions are associated with increased intraoperative blood transfusions [15]. Tamandl, D and colleagues published in 2011 that the patients who developed grades 2 or 3 of hepatic sinusoidal dilatation had a significantly shorter PFS (HR: 2.05; 95% CI, 1.23–3.39, p = 0.005) and OS (HR: 2.90; 95% CI, 1.61–6.19, p < .001) than patients without this alteration. Those cases also had significantly more intrahepatic recurrences (66.7% vs 30.5%, p = 0.003). So, these authors conclude that SOS due to oxal-based chemotherapy may not only compromise perioperative outcome, but in addition can lead to early recurrence and could decrease survival in the long term. Strategies to prevent this condition are clearly needed [47]. To those who have resectable disease, there is a price to be paid in the development of chemotherapy-induced hepatic injuries [42, 45, 48]. There are four degrees of sinusoidal dilatation described: G0: absent, G1: mild, with centrilobular involvement but limited to one-third of the surface lobular, G2: moderate, with centrilobular involvement but limited to two-third of the surface lobular, and G3: severe, with complete lobular involvement [15]. Prolonged SPC alters liver parenchyma and increased morbidity after major resection under total hepatic vascular exclusion, but it does not increase operative mortality. This should be taken into consideration before deciding a major liver resection in patients who have received SPC [16]. Morbidity rate in patients with SOS is about 36%, and it is well demonstrated that this is correlated to number of cycles oxal-based chemotherapy [46]. SOS is well associated with the use of oxal-based chemotherapy, and represents a spectrum of hepatotoxicity, with nodular regenerative hyperplasia (NRH) representing the most significant degree of injury [19]. Morris-Stiff, G and colleagues published in 2014 a study to determine the prevalence of NRH in patients undergoing resection of CLMs, and to evaluate its impact on outcome, and they concluded that NRH is not an uncommon finding amongst patients with SOS in patients having received oxal-based chemotherapy. However, data on outcome would suggest no increased morbidity and mortality associated with the presence of NRH [49]. Even though multiple studies have shown that patients with damaged livers have higher perioperative morbidity and mortality rates than those with healthy livers, others have directly compared perioperative outcome with and without SPC, and have not been able to significantly demonstrate this phenomenon [22]. The reason for this discrepancy probably lies in factors of timing and dose of SPC treatments and extent of hepatic resection. Moreover, age and oxal predispose for the development of sinusoidal dilatation; therefore, caution must be taken in old patients treated with oxal [50, 51]. Prolonged SPC treatments and short intervals between the cessation of chemotherapy and surgery have been found to be associated with significantly increased morbidity [22, 24]. Finally, better comprehension of the molecular events underlying chemotherapy-associated hepatic injury might also be a source of help in patient management. Global gene analysis has shown activation of several pathways in human liver with oxal-related SOS, namely acute phase response, coagulation, fibrosis/hepatic stellate cell activation, oxidative stress, hypoxia, and angiogenesis [43]. This provides new insights into mechanisms underlying CALI in humans and potential targets relating to its diagnosis, prevention, and treatment. Activation of vascular endothelial growth factor and coagulation pathways could explain, at a molecular level, the clinical observations that bevacizumab and aspirin have preventive effect in SOS [46, 52, 53]. Ribero, D and colleagues reported in 2007 that the addition of bevacizumab to oxal-based chemotherapy reduced the incidence of SOS of any grade by half (27% vs 54%) and lowered severe, grade 2–3 sinusoidal dilation (8% vs 28%) to about a third of reviewed pathologic specimens [52]. In patients treated by oxal-based chemotherapy, aspirin intake appears to be associated with a reduced risk of sinusoidal lesions but should be tested in a randomized phase II study [54].
Irinotecan (irino) is a topoisomerase I inhibitor which prevents DNA unwinding and results in failure of DNA replication, DNA strand breaks, and cell death. During its development, it was known as CPT-11 [55, 56]. DNA topoisomerases are the targets of important anticancer and antibacterial drugs. Irino is activated by hydrolysis to SN-38, an inhibitor of topoisomerase I. This is then inactivated by glucuronidation by uridine diphosphate glucoronosyltransferase 1A1 (UGT1A1). The inhibition of topoisomerase I by the active metabolite SN-38 eventually leads to inhibition of both DNA replication and transcriptions [55, 56]. In 1996, Irino received accelerated approval by the FDA and then full approval in 1998. Its main use is in CRC, in particular in combination with other chemotherapy agents. In a randomized trial that evaluated irino in patients with unresectable CLMs, OS after irino alone was equivalent to that of patients treated with standard bolus 5-FU/LV, but the combination of irino and 5-FU/LV improved OS by 2.2 months over 5-FU/LV alone (14.8 vs 12.6 months; p = 0.04) [57]. The current regimen is so-called FOLFIRI, which consists of infusional 5-FU, LV, and irino, and was associated in early publications in the 2000s with further improvement in OS (17.4 vs 14.1 months; p = 0.03) [58].
Adverse effects: The most significant side effects of irino are severe diarrhea and extreme suppression of the immune system [56]. Irino-associated diarrhea is severe and clinically significant, sometimes leading to severe dehydration requiring hospitalization or intensive care unit admission. This side-effect is managed with the aggressive use of anti-diarrheal such as loperamide or co-phenotrope after the first loose bowel movement [48]. The immune system is adversely impacted by irino. This is reflected in dramatically lowered white blood cell counts in the blood, in particular the neutrophils. The patient may experience a period of neutropenia (a clinically significant decrease of neutrophils in the blood) while the bone marrow increases white-cell production to compensate [48]. Several specific forms of liver injury have been associated with various chemotherapeutic regimens, including steatosis and steatohepatitis with prolonged treatment with 5-FU or with irino-based therapy. Hepatotoxic effects on the non-tumoral liver parenchyma have been reported, and are included in the so-called “Chemotherapy associated steatohepatitis or CASH” [59]. Steatohepatitis diagnosis is based on a histological triad: steatosis, balloonisation, and rich inflammation. Macroscopically, liver has typically yellow appearance, commonly so-called “yellow liver” [60]. The progressive features of CASH will begin in the early stages as steatosis, continuing in an intermediate step as steatohepatitis and then with lobular inflammation and finally, in the late stage with the hepatocyte necrosis that will finish in liver cirrhosis [61]. It has been shown at large that steatohepatitis is associated with an increase in morbidity and occasionally mortality after hepatic surgery and patients with CLMs were recommended that the chemotherapy regimen should be carefully considered because the risk of hepatotoxicity is significant [15, 17]. Vauthey, J and colleagues reported in 2006 a systematically analyzed hepatic injury in 158 patients treated with varying SPC regimens for CLMs, and correlated them to postoperative outcomes after hepatic resection [17]. This study distinguished steatosis and steatohepatitis as separate pathologic findings, and demonstrated that steatohepatitis induced by irinotecan-based chemotherapy was associated with an increase in 90-day mortality following major hepatectomy for CLMs (14.7% vs 1.4%, no steatohepatitis; p = 0.001). Furthermore, patients with steatohepatitis have a higher risk of death from postoperative liver failure compared with all other patients (6% vs 1%; p = 0.01) [17]. Morbidity rate in patients with CASH is about 33%, and it is well demonstrated that this correlated to the number of cycles of irino-based chemotherapy [17]. Analysis of the impact of steatosis on outcome after liver resection suggests that morbidity is increased but not mortality. While steatohepatitis may be associated with increased 90-day mortality due to liver failure after surgery [62], irino-based regimens are associated with increased risks of CASH, and consequently there is an increase in morbidity and mortality rates after hepatectomy, especially after major liver resections; therefore, it is important that liver surgeons should have an adequate knowledge of the chemotherapy-associated hepatotoxicity [44]. Over a 10-year period, approximately 9–20% of patients with steatohepatitis have developed cirrhosis. Of these cirrhotic patients, 22–33% of them have developed end-stage liver disease. Incidence of chronic liver disease is however not yet evaluated, particularly for patients who receive multiple cycles of adjuvant or maintenance chemotherapy and, despite an apparent initial indolent course, delayed complications could develop [60].
An open question therefore is whether CALI, notably SOS and NRH, are reversible once the cause has stopped, and in which time frame. In the short term, histological persistence of SOS and NRH in the setting of two-stage hepatectomy was observed, suggesting that there is no advantage in delaying an operation in terms of tumor response to chemotherapy. In the long term, the issue is more open: the analogy with settings in which NRH and portal hypertension was noted 2–3 years after treatment, suggests that changes are not always reversible, and persistent SOS, NRH, and even fibrosis may occur several months after end of chemotherapy.
Molecular Targeted Agents: Overview and Toxicity
In the third millennium, the integration of molecularly targeted agents, such as bevacizumab, cetuximab, and panitumumab into treatment strategies has further increased response rates to an impressive 70% [52]. Further, by combining surgery with these newer chemotherapeutic agents and regimens, 5-year survival rates approach 50–60% following hepatic resection of CLMs [11, 53, 54]. The epidermal growth factor receptor (EGFR), also called ErbB-1 and HER1 in humans, is a trans-membrane glycoprotein receptor [38, 63]. The EGFR is a member of the human epidermal growth factor receptor (HER-erbB family of receptor tyrosine kinases). It is selectively activated by ligands belonging to the epidermal growth factor family of the peptide growth factors. The receptor auto-phosphorylation leads to the activation of multitude pathways, including the RAS/RAF mitogen-activated protein kinase MAPK, the PI3K/AKT, and the JAK/STAT3 pathways, which can be responsible for cancer cell proliferation, survival, invasion, metastases, and neo-angiogenesis [64]. Currently, two monoclonal antibodies target EGFR; the chimeric IgG1 moAb cetuximab and the fully humanized IgG2 moAb panitumumab have been developed and introduced into clinical practice. By binding the EGFR extracellular domain, these moAbs inhibit its dimerization, its subsequent phosphorylation and its downstream signaling [64]. EGFR amplification and EGFR gene copy number variation have also been investigated, but only a small amount of evidence can support their use as predictive markers of response [65]. The KRAS test was the first genetic test to guide treatment of CRC. Currently, this KRAS testing should be performed on all presenting cases of CLMs to ensure access to this treatment option [51]. Approximately 30–40% of CRC patients carry alterations or mutations in the KRAS gene which are associated with lack of activity of anti-EGFR moAbs [58]. In our group (Grondona, J and colleagues), since 2009 to 2014, in a total of 300 KRAS tests, 109 KRAS gene mutations were observed (36.66%). The concordance between the primary tumor and metastasis is high, with only 3–7% of the discordant tumors. Most mutations occur in Exon 1, codons 12 and 13; Exon 3, codon 61, and Exon 4, codon 146 of the KRAS gene. The result of these mutations is the constitutive activation of signaling pathways KRAS. Multiple studies have shown that patients with tumors harboring mutations in KRAS are unlikely to benefit from therapy anti-EGFR antibody, either as monotherapy or in combination with chemotherapy [66, 67]. So, the presence of these mutations correlates with primary resistance to anti-EGFR moAbs [57]. In the last decade, one of the questions of the researches undertaken was whether chemotherapy can cause RAS mutations. A study by Kawamoto, Y and colleagues in 2012 demonstrated that the mutational status of predictive biomarker genes were not altered by FOLFOX therapy [68]. Related to RAS family, the two most common isoforms in CLMs are KRAS/NRAS, both together so-called ALL RAS. Several groups have been focusing on the molecular analysis of additional genes involved in the downstream of the EGFR signaling such as BRAF, NRAS, and PIK3CA. BRAF is one of the primary downstream effectors of KRAS signaling, and the V600E point mutation is the most common alteration that involves this gene, with a frequency in CLMs of about 9–10%. Some retrospective studies suggest that the BRAF/V600E mutation is associated with an unfavorable prognosis regardless of the treatment administered, and with primary resistance to anti-EGFR moAbs. Based on data from different clinical trials, the authors cannot conclude if the BRAF/V600E mutation is a negative predictive marker of response to anti-EGFR moAbs. NRAS mutations in the gene occur in approximately 1–6% of CRC. Tumors without alterations in any NRAS, BRAF, and PI3K genes are associated with good response to anti-EGFR antibody therapy. On the contrary, All RAS (KRAS/NRAS) mutation can be considered as the first negative marker indicative of response to anti-EGFR agents in CLMs. RAS mutation predicts early lung recurrence and worse OS after curative resection of CLMs. This information may be used to individualize systemic and local tumor-directed therapies and follow-up strategies [69]. Currently, the information provided by the RAS mutation status for patient selection is the only one with robust evidence and which warrants its clinical application for cost-effective use of anti-EGFR agents in CLMs. Therefore, choice of the biologic agent to add to the doublet chemotherapy could be individualized based on the RAS status and the clinical scenario [36]. The array of chemotherapeutic and molecular targeted agents available to treat CRC and CLMs is growing. As such, patients who were not typically considered candidates for liver-directed surgery for CLMs are now sometimes considered for potentially curative resection. With the incorporation of targeted therapies in routine cancer therapy, it is imperative that the array of toxicities associated with these agents are well-recognized and managed, especially since these toxicities are different from those seen with conventional cytotoxic agents [60]. The development of targeted therapies is a major breakthrough in the treatment of cancer in general [61, 70].
Cetuximab (cetux) is an EGFR inhibitor used for the treatment of CLMs, metastatic non-small-cell lung cancer, and head and neck cancer. It is a chimeric (mouse/human) monoclonal immunoglobulin G that recognizes and binds to the extracellular domain of the EGFR. Binding of cetux to this receptor does not cause receptor activation, but rather results in a steric interference with the ligand binding site. This effectively prevents ligand activation of the receptor [38]. Cetux has sometimes been used as first-line therapy of unresectable CLMs. By competitively blocking the transmembrane tyrosine kinase EGFR, this agent inhibits cell growth, induces apoptosis, and decreases matrix metalloproteinase and VEGF production; however, the effectiveness of this drug seems to be significantly dependent on the wild-type phenotype of KRAS, as this protein is part of the downstream signal-transduction pathway of EGFR. The benefits of cetux in CLMs are well documented in clinical trials, and are acknowledged in the approval and licensing of this agent. Results from the CRYSTAL study showed that for patients with wild-type KRAS, the addition of cetux to FOLFIRI as first-line treatment for CLMs increases the response rate to 59% compared with 43% using FOLFIRI alone (p = 0.003). Further, a slight increase in PFS was found (9.9 vs 8.7 months; p = 0.017), but all these benefits were not seen among patients with KRAS mutations [70]. The OPUS study was constructed similarly and assessed the addition of cetux to FOLFOX, and it also showed benefits for the KRAS wild-type subgroup (61% vs 37%; p = 0.011) but not for patients with KRAS mutations [66]. In the last decade, the definition of “tumor shrinkage” was widespread, which refers to “contraction or shrinking” of a liver metastasis due to the chemotherapy effect. Early tumor shrinkage, namely 20% or more at the eighth week, was experienced by 64% of patients in the CRYSTAL study and 69% of patients in the OPUS study. In addition, early tumor shrinkage translated into a long-term clinical benefit of 12 months median PFS and 28 months median OS in the CRYSTAL study and 12 months median PFS and 26 months median OS in the OPUS study. In 2009, the FDA approved cetux for treatment of CLMs with KRAS wild-type, since it had little or no effect in CRC harboring a KRAS mutation [71]. The identification of biomarkers associated with disease control, including All RAS mutation status in patients treated with cetux, is changing the current management of CLMs. Based on the CRYSTAL and OPUS studies, the American Society of Clinical Oncology published in 2009 a consensus statement that patients who have CLMs with KRAS mutations in codon 12 or 13 were unaffected by EGFR inhibition, and they recommended that anti-EGFR drugs not be used as part of the treatment regimen for this patient population [72]. The CELIM study randomized patients with unresectable CLMs to receive FOLFOX plus cetux or FOLFIRI plus cetux in a non-blinded fashion. Response rates for the 53 patients in each arm were similar (68% and 57%; p = not significant). But, when analyzed for KRAS mutation, an impressive ORR of 70% was found for the wild-type group. Following a median of eight cycles, complete resection was achieved in 41 patients (34%), suggesting that this regimen was useful for converting unresectable cases to resectable ones [73]. In a secondary analysis, 68 patients were included in a retrospective reevaluation by seven experienced liver surgeons. Twenty-two patients (32%) were considered to be resectable based on initial staging imaging studies, which increased to 41 patients (60%) based on restaging of studies after chemotherapy (p = 0.001). This study concludes that chemotherapy plus cetux yields high ORR compared with historical controls, and leads to a significant increase in resectability [54]. It is interesting to compare the process and liver resection rates in cetux trials in liver- limited KRAS wild-type studies. An interesting observation of the design of four major trials about the evaluation of resectability rate is as follows: in the CRYSTAL and OPUS studies the decision of liver surgery was carried out by clinical oncologists with 13% and 16% of liver resection rate respectively, and in the CELIM and POCHER trials the decision of liver surgery was discussed by MDT with the participation of liver surgeons, with 34% and 60% of liver resection rate respectively. So, this is one of the main items of evidence concerning the interdisciplinary management of CLMs. Finally, KRAS mutational status was shown to be a highly predictive selection criterion in relation to the treatment decision regarding the addition of cetux to FOLFOX-4 for previously untreated patients with CLMs [66].
Panitumumab (panit) was developed by immunization of transgenic mice (Xeno mouse) that are able to produce human immunoglobulin light and heavy chains. After immunization of these animals, a specific clone of B cells that produced an antibody against EGFR was selected and immortalized in Chinese hamster ovary cells. These cells are then used for the full-scale manufacture of the 100% human antibody [67, 74]. Panit works by binding to the extracellular domain of the EGFR, and prevents its activation. This results in halting of the cascade of intracellular signals dependent on this receptor [67]. In 2006, the FDA approved panit for the treatment of patients with CLMs and KRAS wild-type with disease progression on or following chemotherapy regimens based on 5-FU, oxal, or irino. In 2007, it was approved by the European Medicines Agency (EMEA) for the treatment of refractory EGFR-expression of CLMs in patients with non-mutated KRAS [74]. In 2009, the FDA updated the labels of two anti-EGFR monoclonal antibody drugs (cetux and panit) indicated for the treatment of CLMs, to include information about KRAS mutations. This was the result of a study, which demonstrated lack of benefit with panit in patients who carried NRAS mutations [64]. Although they both target the EGFR, panit (IgG2) and cetux (IgG1) differ in their isotype, and they might differ in their mechanism of action. Monoclonal antibodies of the IgG1 isotype may activate the complement pathway and mediate antibody-dependent cellular cytotoxicity [65]. It is not clear at this time if one drug is superior to the other [66].
Adverse effects: Cetux and panit represent an effective treatment option for patients affected by CLMs; furthermore, they are relatively devoid of systemic toxicities, which are commonly observed with standard cytotoxic chemotherapy [75]. Both have been associated with skin toxicity/rash, fatigue, nausea and/or vomiting, diarrhea, renal toxicity and decreased magnesium levels [76, 77]. Often, the patients do not develop anemia or neutropenia and usually have no occurrence of hypersensitivity reactions [78, 79]. Lv, Z and Ning, J conducted a meta-analysis in 2014 to evaluate the efficacy and toxicity of adding cetux to oxal-based or irino-based chemotherapeutic regimens for the treatment of patients with CLMs with wild-type/mutated KRAS tumors. These authors conclude that the incidence of grade 3/4 adverse events, including skin toxicity/rash, diarrhea, hypertension, anorexia, and mucositis/stomatitis, was slightly higher in the combined therapy group than in the chemotherapy-only group [80]. A number of cancer therapy agents are cleared by the kidney and may affect renal function, including cytotoxic chemotherapy agents, molecular targeted therapies, analgesics, antibiotics, radiopharmaceuticals and radiation therapy, and bone-targeted therapies [23]. EGFR inhibitors cause electrolyte imbalances including hypomagnesaemia and hypokalaemia, due to the direct nephrotoxic effect of the drug on renal tubules. Cetux may also result in renal tubular acidosis [81]. Discerning the renal adverse effects resulting from these agents is essential for safe treatment strategies, particularly in those with pre-existing renal disease [81]. Clinical and molecular predictive markers of response are under active evaluation in order to better select patients who could benefit from anti-EGFR treatment, with the aim of both optimizing patient outcomes and avoiding unnecessary toxicities [30]. The majority of patients treated with EGFR inhibitors will experience dermatologic toxicities, most notably the papulo-pustular skin rash, which can impact quality-of-life and affect adherence to therapy. Often, this skin rash is mainly noted in the sun-exposed parts of the body, such as the face or chest [71, 75]. Skin toxicity was classified in 2014 according to the National Cancer Institute Common Toxicity Criteria for Adverse Events version 4.0. The most common skin toxicity was an acne-like skin rash (80% of patients) and paronychia (20%). Other side-effects were trichomegaly, hypertrichosis, and allergic reactions [82, 83]. In addition, the severe papulo-pustular rash and xerosis may be clinical predictors of good response to anti-EGFR therapy. Patients who develop the skin rash should be treated promptly because suboptimal treatment of these adverse effects can lead to delays in taking the prescribed dose of anti-EGFR or certainly to interruption of therapy [77, 78, 83]. A proper care of low-grade toxicities is well recommended in order to reduce progression to high-grade toxicities and the resulting risk of hospitalization, which really impacts on costs [84, 85]. At the start of treatment with EGFR inhibitors, proper patient education about the skin rash associated with these monoclonal antibodies, and the implementation of a pre-emptive, comprehensive skin toxicity program significantly contribute to improving adherence to therapy, optimizing anti-EGFR therapy, and maintaining quality-of-life [75, 83]. Previous experience from clinical trials shows that in some cases proper care and prevention can improve the quality of patients’ lives [82, 85]. Oral antibiotics may be needed for worsening skin rash, such as one accompanied with blisters and ulcers. Otherwise, topical steroid creams such as hydrocortisone may help [85]. Gibson, T and colleagues showed in 2006 the results of a phase III trial which compared panit as a single agent to provide supportive care in patients with previously treated CLMs, and they reported skin-rash toxicity in 90% of patients, with increased severity significantly correlated with improved medium OS [74]. Jaka, A and colleagues reported in 2015 that 81.9% of the patients developed a papulo-pustular rash. Patients who received the most cycles of treatment with the EGFR inhibitors were at the highest risk of developing the rash, and these patients also had the most severe rash reactions (p = 0.03) [77]. In addition, all of the patients who exhibited a complete tumor response had the rash, and the incidence of such rash was lower in patients with poor tumor response (p = 0.03) [77]. Many authors have shown that early acne-like rash predicts superior outcome among patients treated with anti-EGFRs [74, 77, 80, 82, 84].
Bevacizumab (Bev) is a monoclonal antibody which is an angiogenesis inhibitor that slows the growth of new blood vessels and, has shown promising preclinical and clinical activity against metastatic CRC, particularly in combination with chemotherapy [13]. Bev is a recombinant humanized monoclonal antibody, whose main action is the inhibition of the function of a natural protein called vascular endothelial growth factor A (VEGF-A) that stimulates new blood vessel formation [4]. VEGF-A is a chemical signal that stimulates angiogenesis in a variety of diseases, especially in cancer. So, this monoclonal antibody specifically blocks the angiogenesis by inhibition of VEGF-A [14]. Bev is produced in a mammalian Chinese hamster ovary cell expression system in a nutrient medium, and was the first commercially available angiogenesis inhibitor. Bev decreases micro-vascular density and integrity and reduces interstitial tumor pressure in vivo, potentially increasing local delivery of other concurrently administered cytotoxic drugs [86]. Because most malignant tumors are highly dependent on angiogenesis it is expected that bev could stop or delay growth of tumors [87]. Hurwitz, H and colleagues demonstrated in 2004 the benefits of adding bev to systemic chemotherapy for CLMs. In a randomized controlled double-blinded trial, they compared bolus 5-FU/LV plus irino in conjunction with either bev (n = 402) or placebo (n = 411). Patients in the bev group had an almost 5-month improvement in median survival compared with the placebo group (20.3 vs 15.6 months; p < .001), and they had an increase in median PFS (10.6 vs 6.5 months; p < .001) [88]. Bev was approved by the FDA for certain metastatic cancers. In 2004, it received its first approval, for treatment for metastatic CRC when used with standard chemotherapy (as first-line treatment) and with 5-FU-based therapy for second-line metastatic CRC [87]. In 2005, it was approved by the European Medicine Agency (EMA) for use in metastatic CRC. In patients having CLMs with KRAS, mutated tumors could be treated in the preoperative period with bev given intravenously every 14 days, in combination with the chemotherapy drugs 5-FU, LV and oxal or irino [89].
Adverse effects: Although studies have confirmed the survival benefit of bev, the toxic side-effects caused by this monoclonal antibody should also be considered [90]. Bev inhibits the growth of blood vessels, which is part of the body’s normal healing and maintenance. The body produces new blood vessels in wound-healing, and as collateral circulation around blocked or atherosclerotic blood vessels. One concern is that bev interferes with these normal processes, and worsens conditions such as coronary artery disease or peripheral artery disease. These effects are largely avoided in ophthalmological use since the drug is introduced directly into the eye, thus minimizing any effects on the rest of the body [90, 91]. The most frequent adverse reactions to bev are hypertension, renal toxicity, and proteinuria, heightened risk of bleeding and thrombosis, and wound-healing abnormalities [91–93]. Arterial hypertension is the most common bev-associated adverse event, which has been correlated with the biological inhibition of the vascular endothelial growth factor-related pathway, and may represent a possible clinical marker for treatment efficacy [91, 94]. Chun-Ying Qu and colleagues reported in 2015 that Grade 3 hypertension was higher in the bev plus irino, 5-FU, and LV arm than in the placebo arm (11% vs 2% respectively) [95]. Although in several studies the results showed that the incidence of hypertension (≥grade 3) in the treatment group was approximately five times higher than that of the control group, other published studies have confirmed that this side-effect can be well controlled by oral antihypertensive agents [95]. Scartozzi, E and colleagues showed in 2009 that bev-induced hypertension may represent an interesting prognostic factor for clinical outcome in advanced CRC patients receiving first-line bev [94]. Anti-VEGFs agents including bev, aflibercept (VEGF trap), and anti-VEGF receptor (VEGFR) tyrosine kinase inhibitors (TKIs) all cause hypertension, whereas some of them result in proteinuria [81]. The incidence, severity, and pattern of renal toxicities may vary according to the respective target of the drug. The early diagnosis and prompt treatment of these renal alterations are essential in daily practice where molecular targeted therapies have a definitive role in the armamentarium used in many cancers [23, 81]. The risk of thromboembolic events are the deep vein thrombosis, pulmonary embolism, transient ischemic attack, and acute mesenteric ischemia. However, incidences of overall thromboembolic events were not statistically different between the bev/irino and the control arms [95]. Bev could produce abnormalities of wound-healing. In this group are included wound dehiscence, ecchymosis, subcutaneous serum collection, bleeding, and wound infection [92]. Treatment with bev prior to surgery may be associated with increased wound infections and dehiscences, attributable to inhibition of neovascularization of the healing wound. Wound healing complications were increased in patients who had major surgery during bev therapy. In order to diminish or avoid abnormalities of wound-healing, the current recommendation is still to wait at least 28 days from cessation of bev to proceed with surgery, although many liver surgeons prefer to wait 6 weeks [96]. Gruenberger, T and colleagues showed in 2008 that bev can be safely administered until 5 weeks before liver resection in patients with CLMs without increasing the rate of surgical or wound healing complications or severity of bleeding. This is one of the early studies that showed that neoadjuvant bev does not affect liver regeneration after resection [97]. For the aforementioned reasons, it has been recommended that a period of 6 weeks should elapse following the cessation of bev administration before the hepatic resection [92]. Severe but very infrequent side-effects are gastrointestinal tract perforations. These risks include gastrointestinal free intra-abdominal perforation, fistula formation, intra-abdominal abscess, and free air under the diaphragm without identified source. All of them are rare, but are serious complications which may be fatal [95]. Nasal septum perforation and renal thrombotic microangiopathy have been also reported [98]. In December 2010, the FDA warned of the risk of developing perforations in the body, including in the nose, stomach, and intestines, in patients treated with bev. In 2013, F. Hoffmann-La Roche, the Swiss global health-care company that produces bev announced that the drug had been associated with 52 cases of necrotizing fasciitis between 1997 and 2012, of whom 17 patients died. Chun-Ying Qu and colleagues, have shown in 2015 that although the incidence of gastrointestinal perforation caused by bev increased by approximately threefold in the treatment group compared with that of the control group, the incidence in eight studies including one meta-analysis is relatively low (approximately 1–1.5%), suggesting that in this issue bev is safe and non-toxic [95]. The contraindications of bev use include hypersensitivity to its active components or to recombinant monoclonal antibodies, pregnancy, lactation, wound-healing risk complications, severe arterial hypertension, proteinuria, arterial thromboembolic episodes, congestive heart failure, cardiomyopathy [92]. Several meta-analyses suggest that cancer therapy with bev is associated with a slightly elevated risk for developing any serious adverse effects. The incidence of adverse reactions can be limited by effective monitoring and prevention during the course of treatment with this monoclonal antibody [93]. The addition of bev to standard chemotherapy before resection of CLMs does not seem to increase postoperative morbidity. Caution should be given to extended resections (more than three liver segments) and synchronous bowel anastomoses [99]. The higher risks for adverse effects in patients treated with bev should be weighed against its benefits, and be considered by the MDT approach [92, 93].
In conclusion: (1) consensus between many researchers affirm that anti-VEGFs and anti-EGFRs targeted antibodies have increased efficacy of chemotherapy in first-, second- and third-line treatment, (2) patients with liver limited metastatic disease who have response to chemotherapy in combination with targeted antibodies have more chance of curative resection, (3) widespread use of KRAS testing could permit oncologists and liver surgeons to be able to tailor SPC and increased efficacy of different chemotherapeutic regimens, and (4) for the near future, there is the need to have new prognostic markers (to provide information on outcome independent of the therapy that is used) and also new predictive markers (to provide information on outcome with regard to a specific therapy, such as KRAS testing) to increase the efficacy of treatment armamentarium and decrease the toxicity of the drugs. Further, some markers can have both predictive and prognostic value.
Criteria to Define Resectability in Colorectal Liver Metastases
Today, in some medical centers worldwide, patients diagnosed at a metastatic stage appear to be wrongly directed towards a palliative strategy, and thus they can lose a real chance for survival. This probably results from initial misguidance, lack of knowledge of the disease, and the absence of an initial assessment of the resectability of the metastases by a specialized surgeon capable of this evaluation. Progress in recent years in the multimodal management of these patients has led to a clear increase in the resectability rate of CLMs and has improved their prognosis. Planning the right therapeutic approaches for individual patients is becoming more complex, and it requires a close multidisciplinary collaboration between surgical and medical oncologists. A relevant message for the management of CLMs is the importance of a strong and interactive multidisciplinary team (MDT) to plan the care and cure for these patients [100, 101]. This interdisciplinary team (a so-called tumor committee or tumor board) is handled by a case-manager and integrates liver surgeons, clinical oncologists, pathologists, gastroenterologists, and specialists in image-study diagnosis, in order to discuss altogether the oncologic case studies with which they are presented at each meeting [102]. The treatment of disseminated CRC is no longer the domain of only one physician or of one medical group with the same specialty [11]. A proportion of diagnosis and treatment decisions in patients with CLMs could be changed by the discussion in the MDT [12–14]. In two studies with 699 people, working in groups of 2–5, converging evidence of the general collective intelligence factor that explains that a group’s performance is stronger than the maximum individual intelligence of such a group was found [15]. Treatment of CLMs must always be established in an MDT discussion with an analysis of prognostic factors and resectability [3]. The real paradigm shift is the approach to patients with CLMs by an interdisciplinary group where the backbones are the clinical oncologist and the surgeon specialized in liver surgery [5]. In our opinion, the MDT is essential in the assessment and decision-making concerning CLMs to ensure that patients receive optimal care and, further a regular follow-up and re discussion if is necessary should be established for each individual case. In conclusion, currently, the assessment and follow-up of individual cases of CLMs by MDT is mandatory.
No agreed definition of resectability has been reached by several panels of oncologists and liver surgeons considering the treatment of CLMs. The resectability very probably differs from one hospital to another, and depends on the available equipment and the level of surgical expertise. The definition also depends, understandably, on patient-specific data, such as general health, co-morbidities, nutritional status, and more specifically, the presence of a possible underlying liver disease [103]. Currently, with subsequent confirmation that a patient with CLMs is medically fit for general anesthesia and major abdominal surgery, determination of resectability falls into two domains: oncological and technical. From an oncological perspective, evaluation of extrahepatic disease and response to any delivered SPC are the main considerations. From a technical perspective, the ability to remove all viable tumors with negative margins and adequate functional liver remnant are prioritized [104]. With regard to oncological considerations, extrahepatic disease in patients with CRC who also have liver metastases should no longer be considered an absolute contraindication to hepatectomy [105]. The most common sites of extrahepatic disease include recurrent CRC involvement, intra-abdominal lymph node involvement, and lung metastases [106]. However, the presence of extrahepatic disease that is not durably controllable with chemotherapy and/or resection should contraindicate liver resection, although various groups of researchers have reported long-term post-hepatectomy survivals in highly selected patients with clinically apparent extrahepatic disease at all these sites [7]. Currently, patients who have responded to SPC and harbor extrahepatic disease that is amenable to surgical resection, for instance isolated portal lymphadenopathy, could be considered for hepatic resection; and in other patients expected to have long-term control with adjuvant therapies of the extrahepatic disease, for instance a small-volume lung disease, could be considered as well as liver resection. With regard to technical considerations, CLMs were historically divided into three or four groups in order to classify the liver metastases according to the possibility of surgical resection. In the 90s, there was a discussion about how to define whether CLMs were or not resectable, and two main issues were evaluated: when all macroscopic disease could be removed, and when enough healthy liver remnant could be left in place with adequate portal inflow, biliary drainage, and hepatic vein outflow [5, 95]. However, one of the first classifications of patients were published in 2007 by clinical oncologists, Schmoll, H and colleagues, and consisted of three groups as follows: Group 1; patients with metastases that might become resectable, Group 2; patients with unresectable metastases with high tumor burden and/or tumor-related symptoms, and Group 3; patients with unresectable asymptomatic metastases and low aggressive disease [107]. Later, the Group 0 was added by the same authors, which included patients who were initially resectable [89]. The definition of resectable CLMs has changed over the years, and now focuses on all visible liver metastases while preserving at least 20–25% liver remnant with adequate vascular supply and biliary drainage, with the expectation that a resection would render the patient able to stay free of macroscopic evidence of disease [108]. Some liver surgeons have defined the resectability in CLMs in four groups of patients that were differentiated as follows: Group 1; initially resectable, Group 2; not optimally resectable, Group 3; unresectable that could become resectable, and Group 4; unresectable that will never be likely to be resected [3, 108, 109]. The criteria of resectability included estimated residual liver volume, the number and localization of lesions, and the resection margins. Co-existing medical conditions need to be taken into account. Age per se is not a limiting factor [4]. Recently, an established practical approach is to subdivide patients into four clinically defined groups based on last ESMO Clinical practice guidelines published in 2014:
Group 0; primarily technically R0-resectable liver metastases. Upfront resection is an option, specifically when metastases are limited in number and size. The goal is to cure and/or to reduce relapse rate. Chemotherapy intensity is nothing or in some cases is moderate (FOLFOX)
Group 1; potentially resectable metastasis with curative intention but not upfront R0. The goal is objective response and tumor shrinkage. The most active induction chemotherapy should be selected upfront in this group
Group 2; multiple metastasis/sites by definition never or unlikely to be resectable, rapid progression and/or associated symptoms and/or co-morbidities allowing intensive treatment. The goal is disease control and symptom improvement. The treatment intention is upfront active combination of at least doublets
Group 3; never-resectable metastatic disease, without present or imminent mild symptoms and with limited risk for rapid deterioration. The goal is disease control and to preserve the quality of life. The treatment depends on performance status and patient preference. Single agent or doublet low toxicity or only palliative care [103, 110].
In our opinion, one definition with widespread consensus of resectability of CLMs is the ability to remove surgically, with clear microscopic margins (R0), all metastases, without compromising postoperative liver function because of the insufficiency of either the remaining liver volume or biliary and venous vascularization and drainage. Although a surgical margin of 5–10 mm is considered optimal currently, anticipation of at least a microscopically negative margin (1 mm) should be included in the definition of resectability [111, 112]. More recently, the ability to accurately predict the future liver remnant (FLR) volume and function have added to our ability to select resectable cases [97]. In addition, it is difficult to define an absolute value for remaining liver volume because this varies from one patient to another in a multifactorial manner [113]. Liver volumetry has allowed quantification of the anticipated FLR [114]. This has facilitated stratification of the risk of liver failure after major liver resection, and therefore the selection of candidates who may benefit from hypertrophy of liver remnant [113]. The liver volume values most frequently reported in the literature are 20% for a healthy liver, 30% in patients who underwent numerous cycles of SPC, and 40% in patients with an underlying liver disease that impairs their functional hepatic reserve [114]. Moreover, unresectability is defined with one or more of the following criteria: (1) no possibility of upfront R0/R1 resection of all lesions, (2) less than 30% residual liver volume after resection and, (3) metastases in contact with major vessels of the FLR [115]. For insufficient FLR volume, currently there are some surgical procedures that may improve or enhance the normal parenchyma, such as percutaneous portal vein embolization or intra-operative portal vein occlusion, two-stage liver resection, combined liver resection and contra-lateral tumor destruction, and associating liver partition and portal vein ligation for staged hepatectomy (ALPPS) [115]. Only 20% of patients with CLMs meet the principal criterion, that is to say resectability, for receiving surgical treatment. It is therefore important to optimize their management to increase surgical treatment and thereby improve the prognosis of this disease [104].
In conclusion, the technical feasibility of hepatic resection should be based on three criteria related to the remaining liver following resection: (1) the anticipated ability to conserve two contiguous segments, (2) the skill to keep adequate vascular inflow and outflow as well as biliary drainage, and (3) the awaited capacity to preserve adequate FLR.
Response Imaging Evaluation in Colorectal Liver Metastases
Imaging studies are a major component in the evaluation of patients for the screening, staging, and surveillance of CRC. Several modalities are available; these include ultrasound (US), abdominal computed tomography (CT), magnetic resonance imaging (MRI), 18-F-FDG positron emission tomography (FDG-PET), and FDG-PET-CT [116, 117]. US is operator-dependent, plays a limited role in the diagnosis of CLMs, and has generally low sensitivity for detection, especially for small lesions. When the primary endpoint of the study is objective response evaluation, US should not be used to measure tumor lesions [118]. MRI and CT are the best currently available and reproducible methods to measure target lesions selected for response assessment [7]. The choice of CT or MRI for preoperative assessment of CLMs depends on local expertise and availability, CT being limited by radiation dose and further, by limited accuracy for characterization of small lesions. MRI lacks ionizing radiation, offers higher contrast resolution, and provides the possibility of performing multi-parametric imaging, combining T1, T2 and diffusion-weighted imaging with dynamic multi-phases imaging. Currently, MRI is considered the most accurate non-invasive imaging technique for detection of CLMs, and is probably the best choice for a precise preoperative detection of CLMs, particularly for diagnosing small lesions under 10 mm in diameter [117, 119]. Related to this, FDG-PET and FDG-PET-CT in the preoperative imaging evaluation of CLMs have a role mostly for detection of extrahepatic metastatic disease [7]. While there are many sophisticated new methods to explore response to treatment such as perfusion studies, diffusion-weighted imaging with MRI, and texture evaluation which is still at the development stage, at present, in clinical practice, tumor response in CLMs can be evaluated from three different perspectives: (1) change in tumor size, (2) morphologic change unrelated to size, and (3) functional imaging, essentially FDG-PET. For the first perspective, the established methods for evaluation rely on changes in tumor size as defined by WHO and RECIST criteria. A situation is evolving with discrepancies between responses based on both methods, and advances in molecular imaging and image processing are opening new opportunities for response evaluation [104]. The WHO criteria, the first attempt at standardization, use bidimensional measurements and were supplanted in 2000 by the RECIST criteria. Such criteria were published in February 2000 by an international collaboration including the European Organization for Research and Treatment of Cancer (EORTC), the National Cancer Institute of the United States, and the National Cancer Institute of Canada Clinical Trials Group. RECIST, which means “Response Evaluation Criteria in Solid Tumors” is a set of published rules that define when cancer patients improve or respond, stay the same or stable, worsen or progress during treatments. Today, the majority of clinical trials evaluating cancer treatments for objective response in solid tumors use RECIST criteria [118]. Only patients with measurable disease at baseline should be included in protocols where objective tumor response is the primary endpoint. It is considered measurable disease, in the presence of at least one measurable lesion. The standard criteria used to evaluate tumor response by RECIST were developed to assess tumor shrinkage after cytotoxic chemotherapy, and may be limited in assessing response to biologic agents, which have a cytostatic mechanism of action [120]. Target lesions should be selected on the basis of their size, that is to say the lesions with the longest diameter. Since 2009, RECIST has undergone modifications in its rules, and the major changes include the number of lesions to be assessed, which has been reduced from a maximum of ten to five lesions. Thus, it is the sum of maximal transverse diameters of up to five target lesions [111]. RECIST evaluation of target lesions are defined as follows: (1) complete response (CR); disappearance of all target lesions, (2) partial response (PR); at least a 30% decrease in the sum of the longest diameter (LD) of target lesions, (3) stable disease (SD); neither sufficient shrinkage to qualify for partial response nor sufficient increase to qualify for progressive disease, taking as reference the smallest sum LD recorded since the treatment started, and (4) progressive disease (PD); at least a 20% increase in the sum of the LD of target lesions, taking as reference the smallest sum LD recorded since the treatment started or the appearance of one or more new lesions [118]. In summary, many authors have shown that change in tumor size is a strong indicator of response, but the problem is the arbitrary choice of cut-off values. The need for 30% decrease in tumor size derives from historical data collected at a time where precise measurement was impossible. Currently, the new imaging techniques available allow better estimation. Several studies have shown that an early decrease in size of 10% correlates better with outcome than the established 30% decreased by RECIST [104]. The second perspective of evaluating tumor response is morphologic change unrelated to size which is recognized as a valid indicator of response, particularly with target therapy. In this situation, modifications of the tumor texture, enhancement, and margins have been shown to be a reflection of response, regardless of change in tumor size. This was first observed with gastrointestinal stromal tumor (GIST); similar observations have been since made in CLMs treated with bev. Moreover, by evoking necrosis and cavitation, evaluation based on tumor size alone, as is done in the RECIST criteria, is no longer an adequate method. New molecular and functional imaging techniques are currently being developing [97]. Finally, in the last decade several authors have reported that tumor metabolic response to SPC could be quantified by FDG-PET as predictive of prognosis in patients undergoing resection of CLMs. Assessing metabolic response uniquely characterizes tumor biology, which may allow future optimization of patients and treatment selection [121]. In special cases, FDG-PET imaging is a useful tool for assessing treatment response and predicting clinical outcome in patients with CLMs who undergo chemotherapy before liver resection [122].
In conclusion: (1) MRI is currently the best choice for a precise preoperative mapping of CLMs, particularly for diagnosing small lesions, (2) RECIST criteria are not used currently in clinical practice to judge response to chemotherapy in CLMs and further, is imperfect particularly when bev is used, (3) an early decrease in size of 10% in CLMs is considered a radiologic response to chemotherapy, (4) modifications of the tumor texture, enhancement, and margins as well as necrosis and cavitation have been shown to be a reflection of tumor response to chemotherapy, (5) if an abdominal CT was carried out in order to detect CLMs, the diagnosis and assessment of resectability should be completed with MRI, and (6) the role of FDG-PET to evaluate treatment response in CLMs remains to be defined.
In our opinion, imaging studies are the cornerstone of response evaluation in oncology, and resectability or not must be evaluated by multidisciplinary review.
Disappearing Liver Metastases and Tumor Response
Disappearing liver metastases (DLMs) are also called by other authors missing or vanishing liver metastases. However, the verb disappear according to the Cambridge Advanced Learner’s Dictionary means “go where it is impossible to be seen or found” [123]. So, applying this word in relation to CLMs in the context of DLMs refers to a hepatic lesion that is impossible to see or find in the same anatomical liver site that was localized by imaging studies and/or surgical exploration. Therefore, in our opinion we definitely prefer to call these lesions DLMs. A dramatic response to SPC in some patients with multiple bilateral and initially unresectable CLMs sometimes leads to their partial or complete disappearance from imaging studies [98]. DLMs are likely to become in more frequent in the near future due to the widespread use of highly efficient chemotherapy. This creates a new set of issues for managing patients with CLMs following neoadjuvant or conversion chemotherapy. The question remains whether these lesions are undetectable, but still present and therefore likely to grow back [99]. During SPC, some CLMs disappear on serial imaging. This disappearance may represent either a complete radiological response (CRR) or a simple reduction in the sensitivity of imaging during chemotherapy that could lead to a misinterpretation of the assessment of the patient [124]. From the start of the third millennium, the reported concordance between a CRR with complete pathological response (CPR) has been variable, ranging from 20% to 100%. Early evidence have been reported by Elias, D and colleagues in 2004 on 11 patients in whom at least one of the CLMs disappeared on imaging and could not be localized at laparotomy. Local recurrence was seen in three of these patients (27%), with a median follow-up time of 31.1 months. It was concluded in this initial report with small sample size that disappearance of CLMs after SPC on high-quality imaging studies and after intraoperative liver exploration resulted in their definitive cure in approximately 70% of cases [125]. Subsequently, on the contrary, Benoist, S and colleagues in 2006 reported on 66 CLMs that disappeared radiographically following SPC, which represented 20% of all lesions in the cohort. At the time of exploration, 31 lesions (47%) could not be identified, even with intra-operative ultrasonography, and they were consequentially not removed. After 1 year of follow-up, 23 (74%) of these lesions recurred. Of the other 35 liver lesions (53%) identified and removed at surgery, 32 (91%) contained viable disease, suggesting that tumors are still present, even when they disappear on imaging. Thus, it was concluded that 55 of the original 66 lesions (83%) harbored residual viable disease [126]. In our opinion, based on this study, many liver surgeons began to manage these patients with a clear surgical exploration tendency. One year later, Rubbia-Brandt, L and colleagues published in 2007 a study in order to characterize histological response to chemotherapy of CLMs, evaluate efficacy of different chemotherapies schemas on histologic response, and determine whether tumor regression grading (TRG) of CLMs predicts clinical outcome [127]. The TRG scoring system is as following: TRG1; absence of residual cancer and large amount of fibrosis that is CPR, TGR2; rare residual cancer cells scattered throughout the fibrosis, TRG3; more residual tumor cells but fibrosis predominates, TRG4; residual cancer cells predominate over fibrosis, and TRG5; only tumor cells that usually correspond to the cases without chemotherapy treatment [117]. Histological tumor regression of CLMs to chemotherapy corresponds to fibrosis overgrowth that replaces the malignant cells, and not to the increase of intra-tumoral necrosis [127]. In a study published by Blazer, D and colleagues in 2008, related to 305 patients who received a median of five cycles of oxal and irino-based chemotherapy, with or without bev, prior to hepatic resection, a pathological response with no residual histological evidence of tumor or TRG1 was observed in 9% of cases, a major response (1–49% residual cells) was seen in 36%, and a minor response (up to 50% residual cancer cells) was seen in 55% of tumors in the resection specimen. The 5-year survival rates for these three groups were 75%, 56%, and 33% respectively (p < .05) [128]. So, CPR to SPC was independently associated with improved survival on multivariate analysis [128]. In addition, Adam, R and colleagues reported in 2008 that only 4% of patients had a CPR in a series of 767 patients who underwent liver resection after SPC for CLMs [129]. However, this study had the problem that the cohort involved a heterogeneous group of patients with initially resectable and unresectable disease, including some patients with extrahepatic disease. Further, in this study it was observed that a CPR may occur in almost one-third of objective chemo-responders with an age up to 60 years, with metastases up to 3 cm in diameter, and with low CEA values [129]. As with the Blazer study, patients with a CPR (n = 29) had an excellent 5-year survival, which reached 76% compared with 45% for patients without this kind of response (p = .004) [128, 129]. Other report, by Chun, Y and colleagues in 2009 represents an early assessment of the correlation between radiographic and histologic findings, and how they correlate with patient outcome [110]. In this study, they assessed the association of morphologic radiologic response with pathologic response. A total of 234 lesions were examined from 50 patients who underwent hepatic resection after treatment with bev. In the cohort that had an “optimal” morphologic radiologic response, the median number of residual tumor cells was 20%. In contrast, patients who had an “incomplete or absent” response had 50–70% residual tumor cells on pathologic assessment and had a shorter median survival than the responder group [110]. Further, an interesting study by Auer, R and colleagues reported in 2010 a CPR of 65% [124]. Many authors have shown that after SPC, a pathological response of CLMs increasingly occurs, but such responses are not frequently CPR [126, 130]. It is mandatory that DLMs on preoperative CT-scan should be systematically confirmed by a second imaging modality, ideally MRI. With increasing efficacy of SPC or CLMs, more patients will present with one or more DLMs on preoperative cross-sectional imaging [131]. Currently, it is well demonstrated that MRI is more accurate than CT for the evaluation of liver metastases [116]. Among patients with DLMs, an extensive search of the lesions, including full mobilization of the liver, palpation, and intra-operative ultrasound, should be conducted at the time of surgery. The variability in the reported ability to detect DLMs at the time of surgery is undoubtedly multifactorial, but may be related to the quality of preoperative imaging, since some of the CLMs may disappear or may be hardly detectable with surgical exploration and intra-operative ultrasound. Zalinski, S and colleagues reported in 2009 a new technique to mark small lesions with coils before chemotherapy. This technique facilitates the resection of small lesions likely to disappear after SPC [132]. Further, Takahashi, M and colleagues reported in 2012 about the use of lipid-stabilized perfluorobutane microbubbles as an intra-operative ultrasound contrast agent, in order to improve the identification of DLMs and mainly the detection of isoechoic ultrasound lesions [133]. The incidence of DLMs ranges from 5 to 38% in most reported series [124–126, 134–136]. However, incidence is dependent on the extent and sensitivity of preoperative imaging. In addition, disappearance is associated with high tumor number, longer duration of chemotherapy, and small lesion size [107]. It should be noted that among groups such as those at the Gustave Roussy Institute of Paris and The Memorial Sloan Kettering Cancer Center of New York which employ hepatic arterial infusion therapy, the incidence of CPR is much higher [124, 125, 134]. Specifically, Elias, D and colleagues reported a CPR rate of 86% among patients treated with hepatic arterial infusion therapy prior to surgery, versus 22% for those treated with SPC alone [125, 134]. Recently, a new definition was reported of “true complete response”, as either a CPR (no tumor detected in the resection specimen) or a durable complete clinical response (CCR), which is defined as the DLMs not recurring on follow-up imaging studies for a period of time (usually 1 year) [114]. Gruenberger, T and colleagues reported in 2012 that the pathologic tumor response was further defined as the “objective measurement of residual cancer cells in resected tissue”, which has been identified as a reliable prognostic factor in patients with CRC receiving SPC and has been shown to correlate with improved OS after resection of CLMs [7]. Expanded surgical intervention in CLMs and improved chemotherapy led to an increasing problem of DLMs. Treatment of those continues to evolve, and poses a real challenge for liver surgeons [136]. Currently, studies are appearing with an improvement in the CPR rates, such as the one published by Zendel, A and colleagues in 2014 about the influence of CPR on CLMs who received SPC on long-term survival after hepatectomy. Of 472 CLMs evaluated, 86 were no longer visible from images after SPC (14 out of 86 metastases are not included because were treated with local ablation). Of the remaining 72 metastases, only 22 (30.6%) were microscopically persistent metastases or recurrences in situ [109]. Most studies have noted a higher rate of intrahepatic recurrence among patients with DLMs left untreated, compared with patients in whom all initial sites of disease were resected. In several series, recurrent DLMs have been reported to occur in more than one-half of patients in whom the DLMs were not resected [104]. With regard to OS, patients with DLMs have a reported 5-year survival ranging from 40 to 80%. Several studies have noted no statically significant difference in OS among patients with some untreated DLMs versus those in whom all original DLMs sites were treated [131, 135]. The management of patients with DLMs, that is, CLMs that disappear under chemotherapy and are undetectable intra-operatively and finally are “left in place”, continues to be controversial [106]. Moreover, up to the 2010s the collected data suggested that all original sites of CLMs should be addressed surgically or with ablative therapy if possible, even if they are no longer present on re-staging imaging, as they are likely to harbor viable malignant cells that may progress at some point. But today, these statements are a matter of debate and currently, the MDT has a great challenge when all original liver metastatic sites are not detected and some questions emerge about how patients should be managed. One of the therapeutic attitudes could be to go forward and operate without preoperative evidence of residual disease. On the contrary, the current attitude of the majority of the authors is to wait to see some evidence of recurrent liver disease before surgical approach. A complex clinical situation is a patient that has had mixed response characterized by some DLMs but also other areas of residual macroscopic disease, where the clinical approach is more controversial. Further, there is no evidence about what could be the role of a “chemotherapy break” as a provocative test to determine a durable complete response.
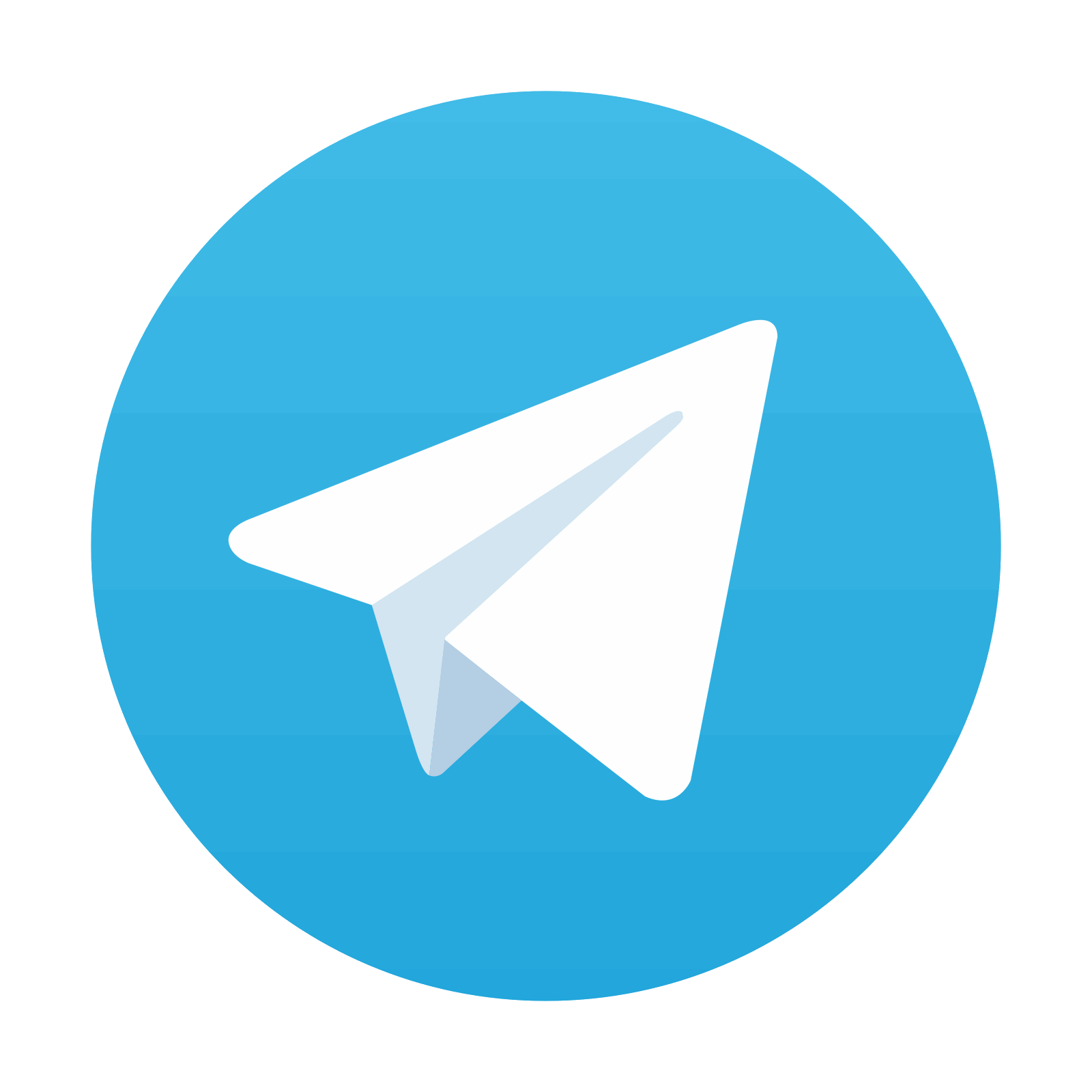
Stay updated, free articles. Join our Telegram channel

Full access? Get Clinical Tree
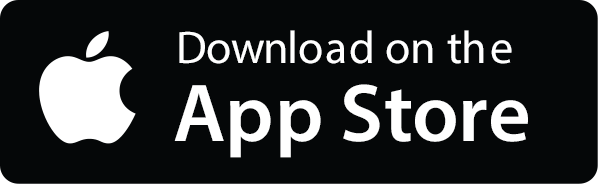
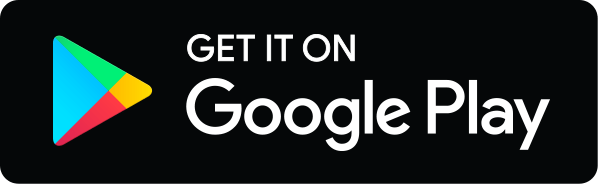