Drug/therapy
Dosage
Efficacy proven in controlled trials
Cholestyramine
4–16 g/day (PO)
Rifampicin
300–600 mg/day (PO) adult
10 mg/kg/day (PO) child
Naltrexone
25–50 mg/day (PO)
Naloxone
0.2 μg/kg/min (IV)
Sertraline
75–100 mg/day (PO)
Contradictory efficacy observed in controlled trials
Ondansetron
4–24 mg/day (PO)
4–8 mg/day (IV)
Ursodeoxycholic acid
10–15 mg/kg/day (PO)
Efficacy shown in case series or case reports
Propofol
10–15 mg (IV bolus)
Lidocaine
100 mg/day (IV)
Dronabinol
15 mg/day (PO)
Butorphanol
1–2 mg/day (intranasal)
Phenobarbitol
2–5 mg/kg/day (PO)
Phototherapy
Plasmapheresis, extracorporeal albumin dialysis (e.g., MARS), plasma separation, anion absorption, nasobiliary drainage, biliary diversion
Liver transplantation
The second-line therapy for pruritus is the antibiotic rifampin (RMP) [16, 19, 20]. It is a (PXR) agonist in addition to inducing the P450 system. Recent in vitro studies have shown that RMP inhibits ATX expression in human HepG2 hepatoma cells and hepatoma cells overexpressing the PXR, but not in the hepatoma cells in which PXR was knocked down [3]. Hence, the beneficial antipruritic action of RMP may be explained, at least partly, by the PXR dependent transcriptional inhibition of ATX expression. Other mechanisms through which RMP might exert its antipruritic actions include induction of the phase I (P450 system), phase II (1A1) biotransformation enzymes and phase III export pumps, such as canalicular conjugate export pump MRP2 [21]. This enables RMP to accelerate detoxification and excretion of numerous compounds, such as bilirubin, bile acids, steroids and drugs. These mechanisms could lead to enhanced metabolism and/or increased secretion of direct or indirect pruritogens [22, 23]. Phenobarbital, an equally potent inducer of the P450 system, does not decrease pruritus as much as rifampin, suggesting that the observed clinical effect of rifampin may not be entirely due to induction of the P450 system [24]. Rifampin has also been shown to be both safe and effective in children with cholestatic disease, with a greater effect in those with intrahepatic cholestasis compared to those with extrahepatic causes [25]. The typical dose is 300–600 mg/day in divided doses in adults, and 10 mg/kg/day in children. Meta-analysis of prospective, randomized, controlled trials has shown RMP to be an effective and safe short-term treatment of pruritus. About 13 % of patients develop hepatotoxicity which can occur as early 3 months after starting therapy [16]. Hence, serum aminotransferases should be monitored at regular intervals when taking RMP [26]. In addition, patients should be informed that RMP changes the color of urine and tears to an orange-red color, a benign adverse effect.
Based on experimental evidence that opioids are important mediators of itch [27], opioid antagonists have been studied in the treatment of cholestatic pruritus and may be recommended as third-line agents for the treatment of cholestatic pruritus. Several clinical trials have proven the beneficial effects of opioid receptor antagonists on pruritus in patients with cholestasis. They significantly reduced itch and/or scratching behavior. Nalmefene, an oral opioid antagonist, improves pruritus as well as plasma bile acid concentrations in patients with cholestatic liver disease. The starting dose is usually 4–10 mg/day, which is then gradually titrated up every 2 days to achieve symptomatic control; the maximum studied dose is 240 mg/day [28, 29]; however, it is not available in most countries. A double blinded, randomized controlled cross over trial of 29 cholestatic patients given naloxone (0.4 mg intravenous bolus, followed by 0.2 μg/kg per minute), showed significant improvement in pruritus over 4 days [30]. Another double blinded, randomized control trial of naltrexone, (50 mg/day orally) in 16 cholestatic patients showed a significant improvement in pruritus within 4 weeks [31]. Parenterally administered naloxone should be reserved for emergency treatment. Naltrexone was proven to be more effective than placebo in reducing pruritus as well as in improving fatigue and depression [32]. Varying degrees of severity of a withdrawal-like reaction, characterized by anorexia, nausea, colicky abdominal pain, pallor, cool skin, and increased blood pressure have been reported in cholestatic patients treated with opioid antagonists. The reaction may begin within hours of administration, but the effect is temporary and usually subsides within 2–3 days, despite continued treatment. It may be as a result of an enhanced opioidogeric tone in cholestatic patients [14]. Therefore, opioid receptor antagonists should be started at very low doses. A breakthrough phenomenon, the sustained exacerbation of pruritus in the early weeks of treatment after an initial decrease, has been seen in 1 of 16 patients treated with naltrexone and 5 of 14 patients treated with nalmefene [13, 31]. This effect may be due to the reversal of the downregulated opiate receptors in the brain during therapy. Unmasking of chronic pain can also occur with prolonged treatment [33].
The serotonin antagonists and selective reuptake inhibitors (sertraline and paroxetine) have been studied in patients with chronic pruritus. Sertraline, a selective serotonin reuptake inhibitor, was associated with improvement in cholestatic pruritus in one retrospective study [34], and a pilot study of sertraline (75–100 mg/day) demonstrated a significant improvement in visual analog scores, scratching activity, duration and distribution of itch in those treated with sertraline as compared to placebo. Initial studies of ondansetron, a 5-HT-3 antagonist, showed a small, but significant positive effect on pruritus [35], but a subsequent double blinded, randomized control trial showed that there was no difference in effect [36]. The mechanism by which serotonin modulates the perception of itch is still not known. Further studies are needed to further evaluate the role and efficacy of serotonergic agents in the treatment of pruritus before they can be routinely recommended. The pharmacobiology of the apparently paradoxical effect of sertraline (increased serotonin) and ondansetron (decreased serotonin) on itch is unclear, but could be due to dichotomous effects of serotonin on the central nervous system versus the peripheral nervous system, downregulation of excitatory 5-HT3 receptors, or disparate effects on various norepinephrine, serotonin, or opioid receptor subtypes.
Other proposed therapies include grapefruit juice, dronabinol, plasmapheresis, and molecular adsorbent recirculating system (MARS). Small studies of PBC patients treated with 600 cc of daily grapefruit juice show some improvement in pruritic symptoms [37]. However, subsequent studies have been unable to produce similar results [38]. Patients with refractory pruritus treated with plasmapheresis had prompt relief of their symptoms, but the duration of this effect was variable from 1 day to 5 months [39]. Patients with liver failure and cholestasis treated with MARS show an associated improvement in hepatic function and disappearance of pruritus [40]. The duration of effect is highly variable lasting from a few days to several months, even disappearing completely in several case reports and series [41–43]. A small case series of patients treated with dronabinol showed a brief anti-pruritic effect, lasting approximately 4–6 h [44]. Ursodeoxycholic acid and prednisone are associated with little to no relief in pruritus [45] of chronic cholestatic liver diseases, even though UDCA is quite effective in cholestasis of pregnancy. A 2002 Cochrane database systematic review evaluated the beneficial and adverse effects of per oral UDCA in PBC. Pruritus as an outcome was also studied. It identified a total of 16 randomized trials evaluating UDCA against placebo or no intervention in 1,422 patients. The conclusion was that UDCA given in doses of 8-15 mg/kg/day for 3 months to 5 years had no significant effect on pruritus [45]. The pruritus of PBC is almost always cured by liver transplantation, which is a viable option for patients with severe intractable pruritus.
Bone Disease
Osteopenic bone disease with a predisposition to spontaneous fracturing is a common complication of chronic cholestatic liver disease. In North America, osteodystrophy in cholestasis is mainly a result of osteoporosis and less that of osteomalacia. Osteoporosis is defined as defective bone formation, whereas osteomalacia is defective bone mineralization resulting from vitamin D deficiency. Cirrhosis has been linked to increased risk of fracture by approximately twofold higher than non-cirrhotic liver disease including PBC [46–48].
Prevalence
Osteoporosis occurs in chronic liver disease of all etiologies, not just cholestatic liver disease [49]. The severity of osteoporosis varies according to the type, severity, and progression of the underlying liver disease. It has been shown that osteoporosis seems to be more striking in patients with cholestatic liver diseases than other patients with chronic liver disease with increased risk of fracture. The prevalence of osteoporosis in PBC patients as defined by the WHO is between 14.2 and 51.5 %. The difference between studies is probably due to differences in patient selection, liver disease severity or hypogonadism. In the largest controlled study of PBC to date [50], lumbar Z-score was reduced and correlated significantly with a calculated risk score based on age, bilirubin level, prothrombin time, serum albumin level, and edema. Women with PBC lose bone mass at a rate approximately twice that seen in age-matched controls, and this accelerated bone loss is the result of mostly decreased formation rather than increased resorption of bone. An important cofactor for the development of osteoporosis in patients with PBC is the menopausal status. Bone mineral density (BMD) is strongly affected by estrogen status, being normal in premenopausal women but deficient in premature menopause [51]. For many years this notion of menopausal patients with PBC being at higher risk of osteoporosis was considered as a controversial subject [46, 49, 52], but two studies confirmed a fourfold increased risk of osteoporosis and a twofold increased risk of fractures in this group of patients compared with the age-matched controls [51]. The reported cumulative frequency of fragility fractures in patients with PBC ranges from 10 to 26 %, with a cumulative frequency of vertebral fractures of 10 % to 20 %. The prevalence of osteoporosis in end-stage PBC patients is 41 % with fractures occurring in 21 % of these patients [28, 48, 53]. BMD in patients diagnosed with PBC before development of cirrhotic state is similar to healthy controls [54]. Similarly, the prevalence of osteoporosis in PSC is 32 % with fractures occurring in 16 % of these patients [28, 55]. The prevalence of osteoporosis is higher in both PBC and PSC patients with stage III and IV disease than stage I and II disease [28, 29, 56]. Children with chronic cholestasis also develop osteopenia proportional to disease severity and progression [57].
One half of patients with PBC who undergo liver transplantation have severe bone disease. Following liver transplantation, there is a rapid loss of bone during the first 3–6 months, which is believed to be related to the immunosuppressive medications and catabolic state in the peri-transplantation period [58]. As a result, one half of patients with PBC experience a pathologic fracture during the first months after liver transplantation. Sometime during the first 4 months, bone formation rates begin to increase; but they do not overtake the increased rate of bone resorption [56] until approximately 12–24 months post-transplantation, when a gradual increase in total bone mass is noted [58]. The incidence of post-transplant fractures is as high as 30-40 % in cholestatic patients [59]. Most of the fractures occur in the first year and fewer beyond 3 years after liver transplantation [60]. Patients at highest risk for post-transplant fracture are those with a low pre-transplant bone mineral density (BMD) and a history of pre-transplant vertebral fracture [61, 62]. Thus, it is imperative to identify and aggressively manage osteopenia and osteoporosis in pre-transplant patients with chronic cholestatic liver disease.
Pathophysiology
Mechanisms of osteoporosis in cholestatic liver disease remain poorly understood but are believed to be multifactorial (Table 9.2). Older age, higher Mayo risk score, lower body mass index, postmenopausal status, and advanced histological stage are all independent risk factors for osteoporosis in PBC patients [51]. Higher serum bilirubin levels, which may be suggestive of more advanced PBC, correlate significantly with a higher rate of bone loss [28]. In PBC, other factors that appear to either directly or indirectly alter bone mass include insulin growth factor-1 (IGF-1) deficiency/gene polymorphisms [63], hypogonadism, and excess alcohol intake. Other factors implicated are subnormal vitamin D levels, vitamin D receptor gene polymorphisms/genotype [59, 64], osteoprotegerin (OPG) deficiency, collagen type Ia1 gene polymorphisms [65], and immunosuppressive therapy before and after transplantation.
Table 9.2
Factors that alter bone mass in patients with PBC
Older age |
Higher Mayo risk score |
Lower body mass index |
Postmenopausal status |
Advanced histological stage |
Insulin growth factor-1 deficiency |
Hyperbilirubinemia |
Hypogonadism |
Alcohol intake |
Subnormal vitamin D levels |
Vitamin D receptor gene polymorphisms/genotype |
Osteoprotegerin (OPG) deficiency |
Collagen type Ia1 gene polymorphisms |
Immunosuppressive therapy |
IGF-1 plays an important role in the process of bone remodeling and maintenance of bone mass. Reduced bone formation in patients with cirrhosis and advanced liver disease is thought to be related to low levels of IGF-1, but no direct relationship has been established between IGF-1 and osteoporosis. OPG secreted by osteoblasts is a member of the TNF receptor super family. It has been found to regulate bone turnover and inhibit osteoclast differentiation in vitro and in vivo, but its role in hepatic osteodystrophy, and particularly in PBC, is still not clear [54, 66].
Studies in PBC have suggested that the receptor activation of NF-kB (RANK) and the receptor activation of NF-kB ligand (RANKL) in addition to OPG are involved in osteoclastic bone resorption [66, 67]; but the exact role of RANK/RANKL in the pathogenesis of low bone turnover in chronic liver disease remains unclear, and there are conflicting findings on the levels of these proteins in the serum. Another study of PBC patients showed that vitamin D receptor genotype correlated with lumbar spine BMD with an allele dose effect. In this study, the risk of vertebral fractures increased from two to threefold with the presence of t-allele [64].
Despite the fact that genetic susceptibility for the development of osteoporosis in PBC has been suggested, including vitamin D receptor gene polymorphisms, collagen type Ia1 gene polymorphisms, and insulin-like growth factor-1 gene polymorphisms, contradictory results have been reported and no definitive genetic susceptibility to bone disease in PBC has been confirmed.
Unconjugated bilirubin inhibits osteoblast activity and function in vitro and in animal models [51], but there is no correlation between unconjugated, conjugated, and total bilirubin levels and bone mineral density (BMD) in patients undergoing liver transplantation. One study confirmed that elevated serum bilirubin alone is not a major contributory factor to hepatic osteodystrophy [68].
Hypogonadism is an established risk factor for osteoporosis in patients with PBC. In postmenopausal women, reduced trabecular bone volume correlates with decreased calcium absorption, which is directly related to longer duration of the disease. Male patients with hypogonadism have decreased levels of estrogen which adversely affects their skeletal health [69, 70].
Metabolism of vitamin D is normal in patients with cholestatic liver disease but calcium and vitamin D malabsorption can occur and lead to bone disease. Diamond et al. [71] compared the largest cohort of 107 patients with chronic liver disease (CLD) including primary cholestatic disorders with 40 age-matched controls, showing normal levels of vitamin D metabolites in the noncirrhotic patients. Cirrhotic patients showed a significant decrease in 25-OH vitamin D and 1, 25-OH2 without histological features of osetomalacia. Hence, these findings point towards a cause of osteoblast dysfunction in CLD that cannot be explained by abnormalities in vitamin D metabolites.
Corticosteroid use in patients with overlap syndrome and in the post-transplant setting has been shown to cause accelerated trabecular bone loss during the first 12 months with doses >7.5 mg/day [49, 72, 73]. Corticosteroids increase osteoclast differentiation and activity by production of interleukins, especially IL-1 and IL-6, and decrease osteoblast differentiation by suppressing differentiation, recruitment, and indirectly reducing the collagen synthesis. As a result, the lowest effective dose of corticosteroid should be used to minimize the deleterious effect on bone metabolism.
Metabolic bone disease, particularly osteoporosis, is becoming a major cause of morbidity in PBC patients post-transplant [49, 74]. Besides corticosteroids, other factors that also contribute include immunosuppressive therapy such a cyclosporine A and tacrolimus (FK506), immobility, and poor nutrition. All these factors contribute to accelerated bone loss after the OLT. Early accelerated bone turnover seen after OLT is not only because of the corticosteroids but has also been attributed to immunosuppressive agents such as calcineurin inhibitors, although their role in bone turnover after OLT is still controversial.
Diagnosis and Monitoring
The AASLD Guidelines [53] suggest that BMD testing should be considered in all patients with PBC at diagnosis and repeated every 2–3 years. There is a clear consensus that BMD should be assessed in all patients with cirrhosis, those receiving long-term corticosteroids (>3 months) or those who have experienced a fragility fracture and before liver transplantation. The severity and progression of bone disease can be assessed by measurement of BMD in different sites. Dual-energy X-ray absorptiometry (DEXA) and dual-photon absorptiometry are noninvasive techniques that quantify bone mass accurately. Common sites used are the lumbar spine (L2–L3) and the superior segment of the femur. This test is quick, precise, reproducible, and minimally invasive. At the time of referral for/diagnosis of PBC, approximately 20 % of patients have osteoporosis, as defined by a T-score below −2.5 in either the lumbar spine or the femoral neck, and approximately 10 % have severe bone disease, as defined by a Z-score below −2 [28, 64]. (The T–score is the number of standard deviations below the mean peak value in young gender-matched normal subjects, whereas the Z–score is the number of standard deviations below mean normal values corrected for age and gender). The risk of osteoporosis (T-score below −2.5) is eight times higher in patients with PBC than in a gender-matched population, whereas the risk of severe bone disease (Z-score below −2) is four times higher in patients with PBC. In addition to DEXA assessment, serum levels of calcium, phosphate, 25-OH vitamin D, and parathyroid hormone (PTH) need to be assessed when monitoring osteoporosis. These are obtained at the beginning of the assessment and at subsequent intervals (6 months to 3 years) according to the severity of cholestasis [52, 53, 75, 76].
Therapy
Therapy of osteoporosis in patients with chronic cholestasis is based on trial results in patients with postmenopausal osteoporosis. A few small studies were conducted in PBC patients with the primary outcome of improving BMD rather than the more clinically important endpoint of fracture rates [63].
Nonpharmacological measures should be taken into account in addition to pharmacological therapies for the management of osteoporosis in patients with cholestatic liver disease. Reversible factors that accelerate bone loss should be eliminated such as alcohol intake, tobacco, caffeine ingestion, and corticosteroids. Patients should be encouraged to do regular weight bearing exercise and to incorporate lifestyle changes that maintain both muscle and bone mass.
In patients with PBC, 2 studies [77, 78] showed calcium supplementation improved bone mass in patients who were vitamin D deficient. High-dose supplemental vitamin D, or 25-hydroxy vitamin D, increased bone mass [79, 80] and reversed some of the osteomalacic changes of bone mass in patients with hepatic osteodystrophy. Further studies of calcium and vitamin D supplements are warranted in patients with cholestatic diseases.
The National Institute of Health guidelines suggest prophylaxis with calcium supplementation of 1,000 mg/day for all adults and 1,500 mg/day for those at risk for osteoporosis, and vitamin D supplementation of 800 IU/day for all adults and 50,000 IU given 2 to 3 times per week for those found to be deficient or at risk for osteoporosis [81]. Treatment can be initiated if T score ≤−2.5, Z score ≤−1.5, or as clinically indicated.
Therapies can be divided into anti-resorptive and hormone replacement. Bisphosphonates, which are used for treating postmenopausal osteoporosis, are the main therapy for treating cholestatic patients with osteoporosis and are usually given with calcium and vitamin D. This class of drugs was studied in a small number of patients with CLD and especially patients with PBC [80]. Although etidronate was no better than placebo in one randomized controlled study [82], alendronate (70 mg/week) was found to improve bone mass significantly after 2 years of treatment in 13 patients with PBC when compared with etidronate [83] and in 15 patients with PBC when compared with 13 patients treated with placebo [84]. Alendronate was not able to show an effect on fracture rate [84]. After OLT, PBC patients are usually treated with bisphosphonates, which have been studied in seven studies in an attempt to reduce the high fracture rate observed in these patients. Intravenous pamidronate has shown no effect on fracture risk in five studies. With respect to the BMD, 3 studies showed an increase in the bone mass but it was mainly limited to trabecular bone (lumbar spine) [85, 86]. Zoledronic acid given within 7 days of transplantation and then 1, 3, 6, and 9 months reduced bone loss in the first 3 months. Lack of effect on the fracture rate is probably related to small size of the studies [86].
Hormone replacement therapy is considered the second-line therapy after bisphosphonates for the treatment of osteoporosis in women with cholestatic liver disease. This is in part because of the risk of thromboembolic disease and malignancy, as well as heart disease in late postmenopausal women. Treatment with estrogens significantly prevents loss of bone mass in postmenopausal patients with PBC [62] and was not associated with worsening cholestasis in a series of 46 patients with PBC who received estrogen treatment for a mean period of almost 5 years [87]. Estrogens were studied in two small-randomized controlled trials of patients with PBC. They were shown to improve BMD after 2 years but no effect on the fracture rate was seen in the first trial [88]. In another study of 18 patients with PBC, only one patient had to stop the transdermal estrogen because of rising aminotransferases [89]. Because of the carcinogenic properties of estrogens, their lack of protective cardiovascular effects, a possible increased risk of dementia, and resumption of menses, however, many postmenopausal women are not enthusiastic about taking estrogens.
Raloxifene, a selective estrogen receptor modulator, has shown some promise as an alternative to estrogen replacement therapy for postmenopausal osteoporosis. In the MORE trial in non-cholestatic, postmenopausal women with osteoporosis, raloxifene (a selective estrogen receptor modulator) in combination with calcium and cholecalciferol, increased BMD in the spine and femoral neck and reduced the risk of vertebral fracture [90]. Raloxifene was evaluated in a pilot study of nine postmenopausal women with PBC who showed a significant increase in bone mass after 1 year of treatment; this improvement in bone mass was not seen in an age and menopausal status-matched control group [91]. Raloxifene and other selective estrogen receptor modulating agents may eventually have a role in cholestatic patients with osteoporosis, but further studies targeting these patients are needed.
Testosterone is used in hypogonadal male patients to stabilize hormone levels. Transdermal testosterone is preferred to prevent any liver side effects from surges in testosterone levels seen with oral or depot preparations.
Other treatments that have been used are sodium fluoride, strontium ranelate, vitamin K derivatives, and recombinant parathyroid hormone.
Sodium fluoride stimulates bone formation, and it prevented bone loss in a 2-year, prospective, double-blind trial of PBC patients [92]. Though very effective at preventing bone loss and reducing the risk of fractures, side effects of severe dyspepsia limit its use. One randomized trial compared cyclical etidronate to fluoride and showed etidronate to be better tolerated and more effective than fluoride at preventing bone loss in PBC [93]. However, a subsequent randomized controlled trial of etidronate in PBC subjects with established osteopenia (T-score <−2.0) found that etidronate was no more effective than placebo [82].
Strontium ranelate has been approved in Europe since 2004 for the treatment of osteoporosis to reduce the risk for vertebral and hip fractures in postmenopausal women [94]. In 2012 the indication was expanded to include men at increased risk for fracture. Recently the European Medicines Agency has restricted the use of this drug because of concerns about its risk for cardiac adverse events. It is not currently approved in the USA, and there have been no studies on its effect in cholestatic patients with osteoporosis.
Vitamin K derivatives, phytonadione and menaquinone may be effective for the prevention and treatment of osteoporosis due to cholestasis [95]. A randomized, control trial showed a higher BMD in patients receiving vitamin K when compared to placebo [96]. Given the low incidence of side effects and demonstrated efficacy, vitamin K should be considered as part of osteoporosis treatment in cholestatic liver disease.
There are several medications available for the treatment of postmenopausal osteoporosis which have not shown efficacy in trials of cholestatic patients. For example, parenterally administered calcitonin did not stop the progression of bone disease in a cohort of PBC subjects [77]. Another study evaluating subcutaneous calcitonin therapy given during the first 6 months after liver transplantation showed that calcitonin did not prevent or reduce accelerated bone loss or spontaneous fractures [97]. Though there are trials showing benefit from PTH in patients with postmenopausal osteoporosis [98], there are no trials with PTH in patients with cholestatic liver disease. Recombinant parathyroid hormone is reserved for patients intolerant of bisphosphonates to prevent fragility fractures.
Dyslipidemia
Cholestatic disorders manifest a distinct pattern of dyslipoproteinemia due to the retention of cholesterol, phospholipids, and bile salts that are normally secreted in bile [99].
Prevalence
Lipid abnormalities are found in 85 % of patients with PBC. At the time of the first visit, approximately 75 % of PBC patients have a total cholesterol above 200 mg/dl. A cross-sectional study in patients with PBC showed that patients with early and intermediate histologic stages have mild elevations of very low density lipoproteins and low density lipoproteins, and marked increases in high density lipoproteins. Within the LDL fraction of the serum of cholestatic patients, three distinct lipoproteins can be identified: β2-lipoprotein (triglyceride rich), also known as lipoprotein Y (LP-Y); lipoprotein X (LP-X), and normal LDL. LP-Y appears to be a remnant of a triglyceride-rich lipoprotein that is distinct from IDL. LP-X is a complex composed of equimolar amounts of excess phospholipid and unesterified cholesterol. The main protein component is albumin within the core and apolipoprotein C upon the surface. In contrast, patients with advanced disease have marked elevations in LDLs with the presence of lipoprotein-X, and a significant decrease in HDL [100]. Cholestatic patients with elevated triglyceride levels often have clear (not lipemic) serum because most of the triglycerides are contained in LP-Y and LDL.
Pathophysiology
Lp-X is about 50 nm in diameter and its major component bile acid is lithocholic acid. Early studies reported the presence of Lp-X in the serum of patients with cholestasis [101, 102]. Cholestatic patients have elevated levels of lipoprotein X, which may be protective against atherogenesis [103]. However, lipoprotein X may also contribute to hypercholesterolemia in cholestatic patients by not effectively downregulating hepatic hydroxymethylglutaryl coenzyme A reductase [104]. The presence of serum Lp-X does not facilitate the distinction between intrahepatic and extrahepatic cholestasis. Bile lipoprotein is a precursor of Lp-X and in cholestasis this refluxes into the plasma pool and binds to albumin to form Lp-X. Serum Lp-X usually disappears soon after the cholestasis is relieved. Lp-X is not significantly taken up by macrophages but is taken up by hepatocytes. Sometimes severe and prolonged cholestasis can lead to very high serum Lp-X concentrations, resulting in severe hypercholesterolemia and tendon xanthomata [105]. LP-X is also found in patients with lecithin-cholesterol acyltransferase (LCAT) deficiency, an enzyme responsible for cholesterol esterification in plasma. In addition to cholestasis and LCAT deficiency, Lp-X may also be found in the plasma after intravenous infusion of intralipid emulsion such as occurs with total parenteral nutrition. Pseudohyponatremia has also been described in some patients with severe hypercholesterolemia secondary to raised Lp-X. Thus the presence of Lp-X should be considered in pseudohyponatremia associated with cholestasis [106]. Lp-X possesses strong aggregatory properties and can bind to alkaline phosphatase (AlkPhos) producing an Lp-X-AlkPhos complex. This complex may be found in cholestasis; typically that evoked by hepatic malignancy.
Clinical Significance
Usually in patients without cholestasis, the risk of atheroma formation is raised if the level of HDL cholesterol is low and the LDL cholesterol is high. Xanthoma formation can occur in patients with PBC as a result of prolonged increase in total serum cholesterol and lipid levels. The implications of this finding with respect to coronary artery disease, cerebrovascular disease, and overall morbidity and mortality are poorly understood. Despite marked hypercholesterolemia, excess mortality from cardiovascular disease has not been associated with PBC [107]. Studies investigating the risk of dyslipidemia in PBC [108] have shown that hypercholesterolemia in chronic cholestasis is not associated with increased clinical or subclinical atherosclerosis. A prospective observational study of 312 PBC patients followed for 7.4 years showed no statistical difference in the incidence of atherosclerotic death when compared to age and sex matched controls [109]. The reason for the observed lack of cardiovascular events in patients with cholestatic dyslipidemia may be related to this difference in composition of the low density lipoproteins. The three factors with protective effects regarding the risk of atheroma formation in hyperlipidemia are: LP-X, HDL cholesterol, and adiponectin, all of which are raised in patients with chronic cholestasis.
Therapy
As is evident from the above discussion, only patients with chronic cholestasis having other risk factors for atheroma formation are likely to benefit from therapy [100, 108, 110]. Treatment includes lifestyle changes and medical therapy (Table 9.3). A small study of 6 PBC patients treated with simvastatin, a 3-hydroxy-3-methylglutaryl coenzyme A reductase inhibitor, showed a significant reduction in total cholesterol, LDL cholesterol, alkaline phosphatase, gamma-glutamyltransferase, and immunoglobulin M [111]. Pravastatin, another HMG-coA reductase inhibitor, at 20 mg/day showed marked decrease in cholesterol and total bile acid levels, with pronounced decreases in cholic acid and chenodeoxycholic acid [112]. The use of reductase inhibitors is increasing in patients with cholestatic liver disease without subsequent reports of hepatotoxicity. However, further studies regarding clinical benefit and risk are needed before HMG-coA reductase inhibitors can be routinely recommended in cholestatic patients. Therapy with UDCA has been shown to lower the LDL levels in patients with PBC and has been useful in some patients with xanthelasmas. Fibrates have not been shown to be efficacious in dyslipidemias seen in chronic cholestasis [107]. Ezetimibe, which inhibits the intestinal absorption of cholesterol (Neiman Pick C1 like protein), was investigated in patients with PBC [104]. Alone or in combination with a statin, ezetemibe reduces LDL cholesterol in cholestasis [103, 104, 109]. Statin therapy is relatively ineffective in lowering Lp-X [113]. Complete regression of xanthomata has been described by treatment with apheresis, which has been suggested by some as the first-choice treatment for patients with massive Lp-X accumulation due to cholestasis [113, 114].
Table 9.3
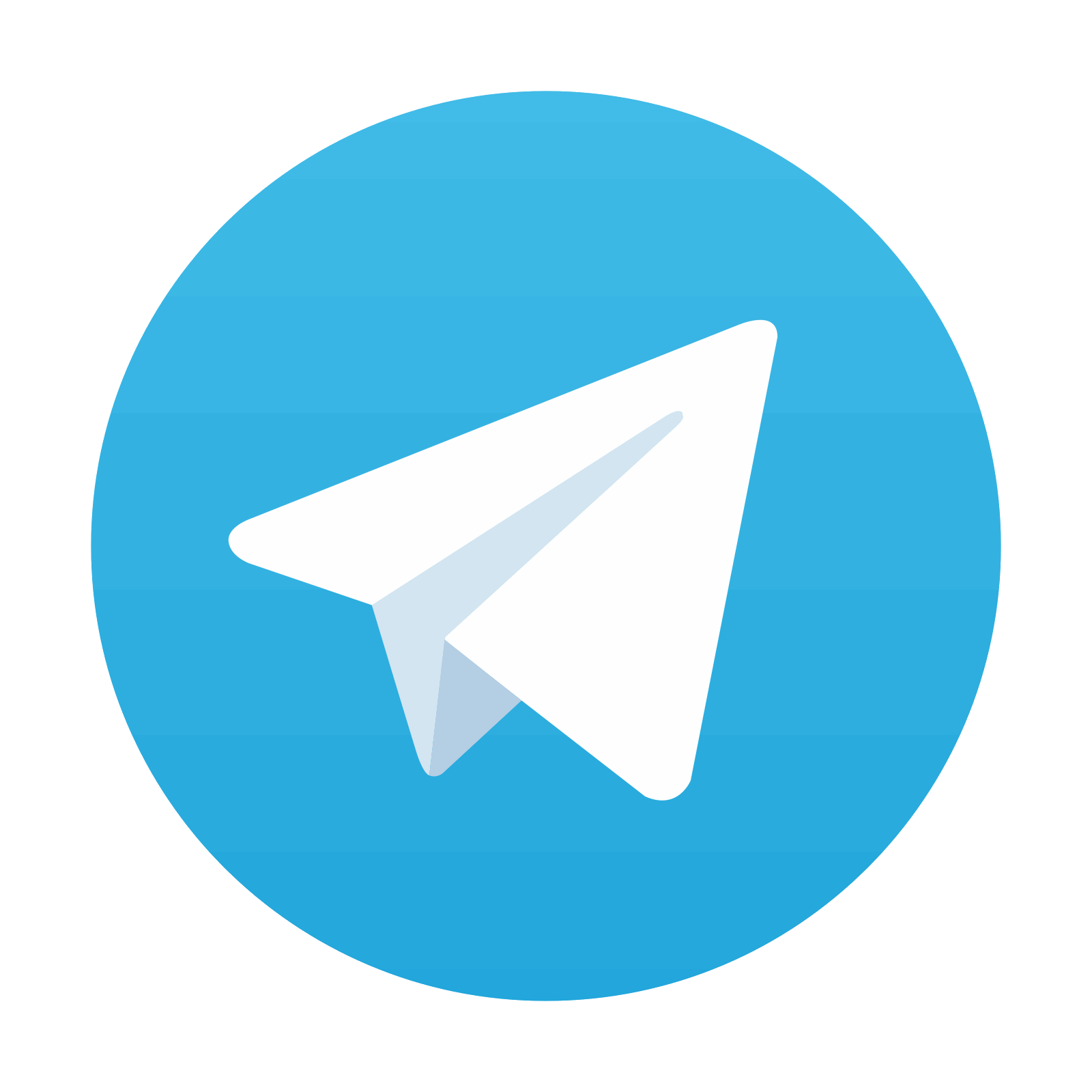
Treatment options for osteoporosis
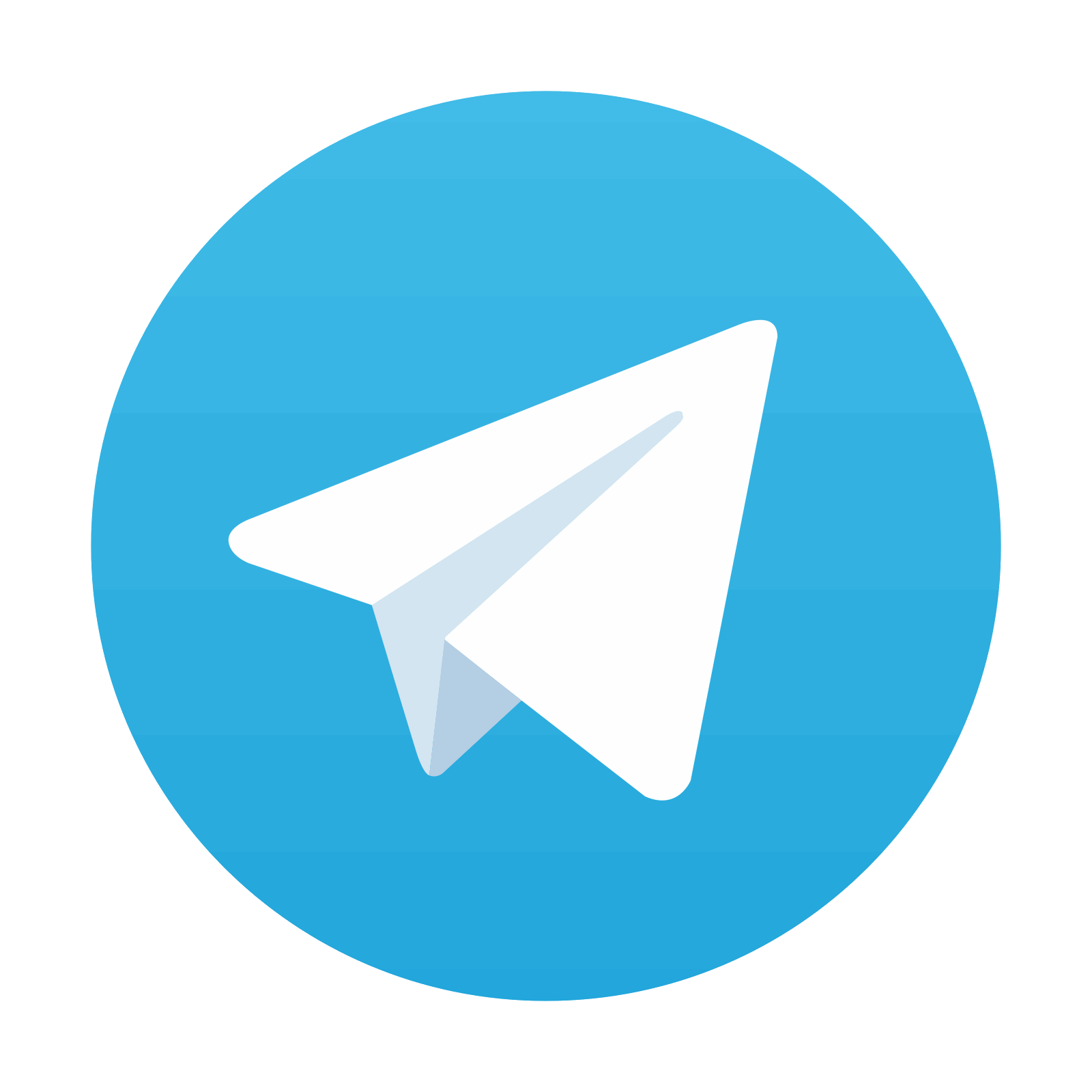
Stay updated, free articles. Join our Telegram channel

Full access? Get Clinical Tree
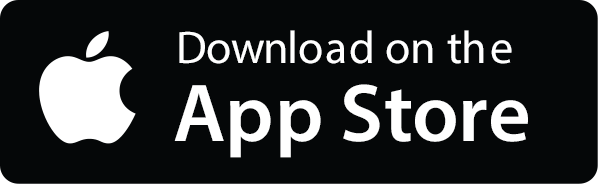
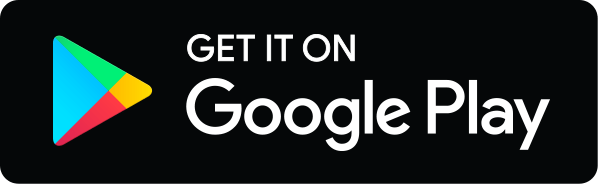
