(1)
Department of Plastic Surgery, The University of Texas MD Anderson Cancer Center, Houston, 77030, TX, USA
Keywords
Abdominal wall reconstructionVentral herniaComponent separationMinimally invasive component separationBioprosthetic meshPedicled flapFree flapSurgical meshIntroduction
The goals of abdominal wall reconstruction are to re-establish the integrity of the musculofascial layer and provide stable external cutaneous coverage. Surgical planning for abdominal wall reconstruction must include the potential for loss of skin and musculofascial tissue. These tissue defects can be caused by tissue necrosis, infection, resection, incisional hernia with loss of domain, denervation of adjacent abdominal musculature, and/or scarred, retracted tissues that limit the ability to advance skin and fascia. Local wound conditions including bacterial contamination, previous surgeries, and previous radiotherapy can contribute to an increased risk of compromised wound healing, surgical site infection, and failure of the reconstruction. Patient factors such as advanced age, comorbidities, obesity, immunosuppression, poor nutritional status, tobacco use, and pulmonary disease also increase the risk of complications and thus must be considered in the perioperative planning and management [1–5]. Abdominal wall reconstructive procedures themselves can result in significant complications including mesh infection, seroma, dehiscence, recurrent hernia, abdominal compartment syndrome, and visceral injury. These may require complex reoperative surgeries, prolonged periods of wound care, and/or staged salvage procedures.
Direct suture repair of small ventral hernias can be performed with relatively low complication rates; however, hernia recurrences after direct suture repair are common, occurring in 10–60% of patients [3, 6]. When subsequent recurrent hernia repairs are performed, the recurrence rate increases each time, with progressively shorter intervals between repair and re-herniation [7]. The use of mesh reinforcement reduces hernia recurrence rates compared with primary suture repair alone [3, 8]. However, the addition of mesh does not prevent all recurrences. For example, in one long-term follow-up study, synthetic mesh reinforcement during elective repair of small (<6 cm), uncontaminated ventral hernias was associated with a 32% 10-year cumulative recurrence rate. On the other end of the spectrum, our group reported a 7.7% hernia recurrence rate in patients undergoing ventral hernia repair with inlay mesh and primary fascial closure after mean follow-up of 31 months [9]. Furthermore, despite advances in surgical technique and implantable mesh materials, other long-term outcomes of ventral hernia repair, including length of hospital stay and need for reoperation, have not significantly improved over time [2]. In addition, the incidences of surgical site occurrences, wound dehiscence, wound infection, seroma, and fascial separation in elective ventral hernia repair are higher than in other “clean” general surgery procedures [2, 3, 5]. In patients undergoing repairs of incisional hernias with previously documented wound infections, up to 41% will develop another wound infection, whereas only 12% of patients without a history of infection will develop a wound infection after hernia repair [2]. Clearly there are ongoing difficulties with hernia repair particularly wound complications and infection, and further improvements are needed.
Various meshes have been developed to improve results in hernia repair. Commonly used implantable meshes include macroporous (monofilament and double-filament polypropylene), microporous (extended polytetrafluoroethylene [ePTFE] ), composite (anti-adhesive layer laminated to macroporous mesh), and bioprosthetic (decellularized, processed human, or animal tissue) meshes [10, 11]. Macroporous meshes have large pore sizes that allow for ingrowth of scar tissue. When placed in contact with abdominal viscera, macroporous meshes are associated with the formation of bowel adhesions, bowel obstructions, and enterocutaneous fistulae [12, 13]. Therefore, these materials should be avoided or used in combination with omental coverage or anti-adhesive barriers when placed in contact with bowel. Microporous meshes have a smaller pore size that does not allow for significant tissue ingrowth but may lead to encapsulation, periprosthetic fluid collection, and bacterial overgrowth. Therefore, microporous mesh has a lower affinity for visceral adhesions but may be more susceptible to infection. A wide variety of composite materials are now available that combine various qualities, such as having macroporous mesh on one side to promote tissue ingrowth and microporous mesh on the other to reduce the risk for adhesions to the mesh (polypropylene/ePTFE). In an attempt to take advantage of macroporous and anti-adhesive characteristics , anti-adhesive bilaminar mesh (such as Sepramesh [polypropylene/carboxymethylcellulose and hyaluronic acid, Bard, Inc., Murray Hill, NJ]) was developed to induce fibrovascular incorporation in the sublay plane and minimize visceral adhesions with the microporous component or bioresorbable component when placed intraabdominally. Clinical evidence suggests a reduced risk of adhesions for composite and coated synthetic meshes compared with traditional synthetic meshes [14–18]. The reported relative benefits of these different prostheses with regard to adhesion formation and risk for infection vary [12, 16, 19–22].
Bioprosthetic meshes are an equally diverse and expanding class of mesh materials. Certain characteristics are thought to contribute to the successful use of particular biologic repair materials in the setting of wound contamination or low-grade infection. These mesh properties include an intact extracellular matrix and the ability to support tissue regeneration through revascularization and cell repopulation. It has been hypothesized that resistance to infection for some biologic repair materials may be related to the ingrowth of cells and vasculature structures [23]. The neovascularization demonstrated in studies of some biologic repair materials may allow these materials to better resist infection when placed in a potentially contaminated field [23]. Data on the ability of some bioprosthetic meshes to support regeneration come from studies in animal models that describe the immunologic response of the host to the prostheses [24]. It should be emphasized that no prospective clinical trials have been completed to date directly comparing different bioprosthetic meshes in incisional hernia repair , and the few clinical data suggesting benefits of one product over another come from small retrospective studies of a limited number of the available bioprosthetic mesh materials. Our group compared porcine and bovine bioprosthetic meshes in 120 patients undergoing abdominal wall reconstruction and found no difference in hernia recurrence 2.9% vs. 3.9% or bulge 7.2% vs. 0% [25]. Data from animal and clinical studies are awaited for the majority of bioprosthetic mesh materials.
Current Indications for Utilization of Bioprosthetic Mesh
Indications for the use of bioprosthetic mesh are based mostly on animal data and short-term low level evidence from clinical studies and case reports. Based on our clinical experience and inferences from The Ventral Hernia Working Group we use the following indications: [24]
- 1.
Contaminated wound (existing wound infection, adjacent ostomy, planned or inadvertent disruption of the gastrointestinal tract’s continuity, enterocutaneous fistula).
- 2.
Complex repair in a patient at high risk for the development of wound healing problems, subcutaneous infection, and/or need for reoperation.
- 3.
Planned exposed bioprosthetic mesh or high likelihood of cutaneous exposure (open abdominal wound closure with a bridging repair or unreliable skin coverage in a patient with multiple comorbidities).
- 4.
Unavoidable direct placement of mesh over bowel and other abdominal viscera.
Surgeon preference and the variables of any given clinical scenario, including patient comorbidities, wound contamination, prior radiation, availability of omentum, and/or posterior sheaths (retrorectus repair), and the quality of the overlying soft tissue, will determine whether bioprosthetic mesh or synthetic mesh is implanted. Regardless of mesh type, the expectations are that the mesh will maintain the abdominal wall contour and not become attenuated, leading to a hernia or bulge. In addition, the mesh should be able to interface with the underlying viscera without forming extensive adhesions that can lead to bowel obstruction or erosion that can lead to fistulization . Both synthetic and bioprosthetic meshes can meet these expectation and the decision to use either is based on patient comorbidities, wound characteristics, quality of the overlying soft tissue, and surgeon preference.
Abdominal wall defects require both reconstruction of the musculofascia and closure of the overlying skin. Musculofascial reconstruction is generally performed with component separation and/or implantable mesh. The use of component separation in complex abdominal wall repair has been described by many surgeons with numerous variations, including reinforcement with mesh [20, 26–27], and is now considered by many experienced hernia surgeons to be the standard of care [20, 28, 29]. Component separation provides an enhanced fascial closure technique and a dynamic repair of the abdominal wall without compromising motor innervation to the abdominal wall muscles.
Patient Selection
In elective hernia repair, patients should be “optimized” before surgery by improving controllable medical and surgical site comorbidities (see Chap. 8: Getting Ready: Preoperative Patient Optimization). Factors such as serum glucose levels and nutritional status should be brought under control, and tobacco use should be eliminated for at least 3 weeks before surgery to decrease perioperative morbidity [5, 30]. Compelling data from the National Surgical Quality Improvement Program highlight the risk of morbid obesity, which increases perioperative complications and deaths [31]. Obese patients undergoing elective hernia repairs should be screened by a nutritional counselor and enrolled in a diet and exercise program to reduce the risk of complications. Patients who are unable to lose weight on a personalized plan should be considered and evaluated for surgical laparoscopic bariatric procedures prior to elective hernia repair.
In non-elective or emergent abdominal wall reconstruction , contaminated wounds should be appropriately debrided and prepared to reduce the bacterial bioburden [32]. Severely contaminated wounds may require a staged approach consisting of serial debridement, dressing changes, negative pressure wound therapy, and delayed fascial closure with bioprosthetic mesh and/or component separation. Systemically ill patients with numerous comorbidities and contaminated fascial defects may benefit from early musculofascial reconstruction with bioprosthetic mesh. Early fascial closure preserves the musculofascial domain, improves ventilatory support, reduces fluid loss, reduces the risk of enterocutaneous fistula, and may reduce the acuity of subsequent wound management.
Abdominal Wall Reconstruction Principles
The general principles of abdominal wall reconstruction include optimization of the patient, preparation of the wound, centralization and reapproximation of the rectus abdominis muscles in the midline, and the use of appropriate synthetic or bioprosthetic material to reinforce the closure.
A key element of the inset of bioprosthetic mesh is to place the mesh in an inlay (intraperitoneal), preperitoneal, or retrorectus position under appropriate physiologic tension; this is in contradistinction to a tension-free repair. Mesh inset under physiologic tension facilitates and stimulates appropriate collagen remodeling to optimize mechanical strength and thus reduce the risk of bulge and laxity. The edges of the bioprosthetic mesh should overlap the undersurface of the musculofascial edge by at least 3–5 cm to allow for remodeling and fibrovascular incorporation. This method of bioprosthetic mesh inlay takes advantage of the mesh’s remodeling mechanism, rather than simple scarring mechanisms, and increases the tensile strength of the junction between the mesh and the musculofascia [24, 33, 34].
The anatomic plane of the bioprosthetic mesh inlay has direct implications for the degree of incorporation at the mesh-musculofascia interface (Fig. 12.1). When possible, it is preferable to avoid insetting the bioprosthetic mesh in direct contact with the peritoneum or preperitoneal fat. To avoid this, the preperitoneal fat pad is dissected away from the posterior rectus sheath and the mesh is placed in direct continuity with the posterior sheath fascia. This improves the fibrovascular infiltration and mechanical strength at the mesh-musculofascia interface better than suturing the mesh to the preperitoneal fat/transversalis fascial layer would. Alternatively, a retrorectus repair can be used, whereby, mesh can be sutured to the semilunar line between the rectus muscle and the posterior rectus sheath.


Fig. 12.1
Anatomic planes of mesh inset. (A) Onlay. (B) Retrorectus. (C) Preperitoneal. (D) Intraperitoneal. (Copyright © 2012 The University of Texas MD Anderson Cancer Center)
Onlay mesh placement is technically easier but has several significant drawbacks and is not often recommended. If a reinforced repair is going to be performed, one generally must be able to close the fascia first, which is not possible before mesh placement in many cases of large defects; the inset of the mesh as an inlay actually helps reduce the tension needed to close the fascial defect. Thus, an inlay mesh placement facilitates primary fascial closure, whereas an onlay mesh placement can be performed only after primary fascial closure is achieved. However, there may be some situations in which an onlay repair is the only safe option, such as when it is impractical to re-enter a hostile abdomen. Although onlay reinforcement avoids placement of mesh directly against intraperitoneal viscera, its positioning in the subcutaneous space may increase the risk of seroma formation and/or cutaneous mesh exposure if wound dehiscence occurs.
When technically feasible, a bioprosthetic mesh inlay repair should be reinforced with a second layer of primary fascial closure over the mesh (Fig. 12.2). This dual-layer repair is preferred to a bridging interposition repair. Centralization of the rectus abdominis muscle complexes reduces the fascial defect and facilitates primary fascial closure. Primary fascial coverage also allows for complete apposition of the bioprosthetic mesh and the overlying musculofascial defect edge. Every attempt should be made to re-approximate the fascia over the inlay mesh as this has a significant impact on outcomes. Our group evaluated the difference in hernia recurrence outcomes between patients reconstructed with primary fascial closure repairs versus those who required bridging repairs. There was a statistically significant higher incidence in hernia recurrence in patients undergoing bridged versus reinforced repairs, 55.6% vs. 7.7%, respectively [9]. Bridging the fascial defect with mesh was an independent predictive factor for hernia recurrence using logistic regression analysis. Bridging the fascial defect was more predictive for recurrence than fascial defect size itself. When primary fascial closure is not attainable, a bridging repair is performed (Fig. 12.3). This is done using a dual-circumferential inlay technique, which allows two concentric suture lines to affix the bioprosthetic mesh directly to the musculofascia [34–37]. Creating direct apposition of the bioprosthetic mesh and the undersurface of the fascial defect itself prevents the collection of fluid between the two layers, which could prevent or delay fibrovascular incorporation and remodeling. To prevent the collection of fluid at the mesh-musculofascia interface, closed-suction drains are placed between the musculofascia and the bioprosthetic mesh.



Fig. 12.2
Reinforced mesh repair with primary fascial closure and Minimally Invasive Component Separation. (Copyright © 2012 The University of Texas MD Anderson Cancer Center)

Fig. 12.3
Bridged mesh repair and Minimally Invasive Component Separation. (Copyright © 2012 The University of Texas MD Anderson Cancer Center)
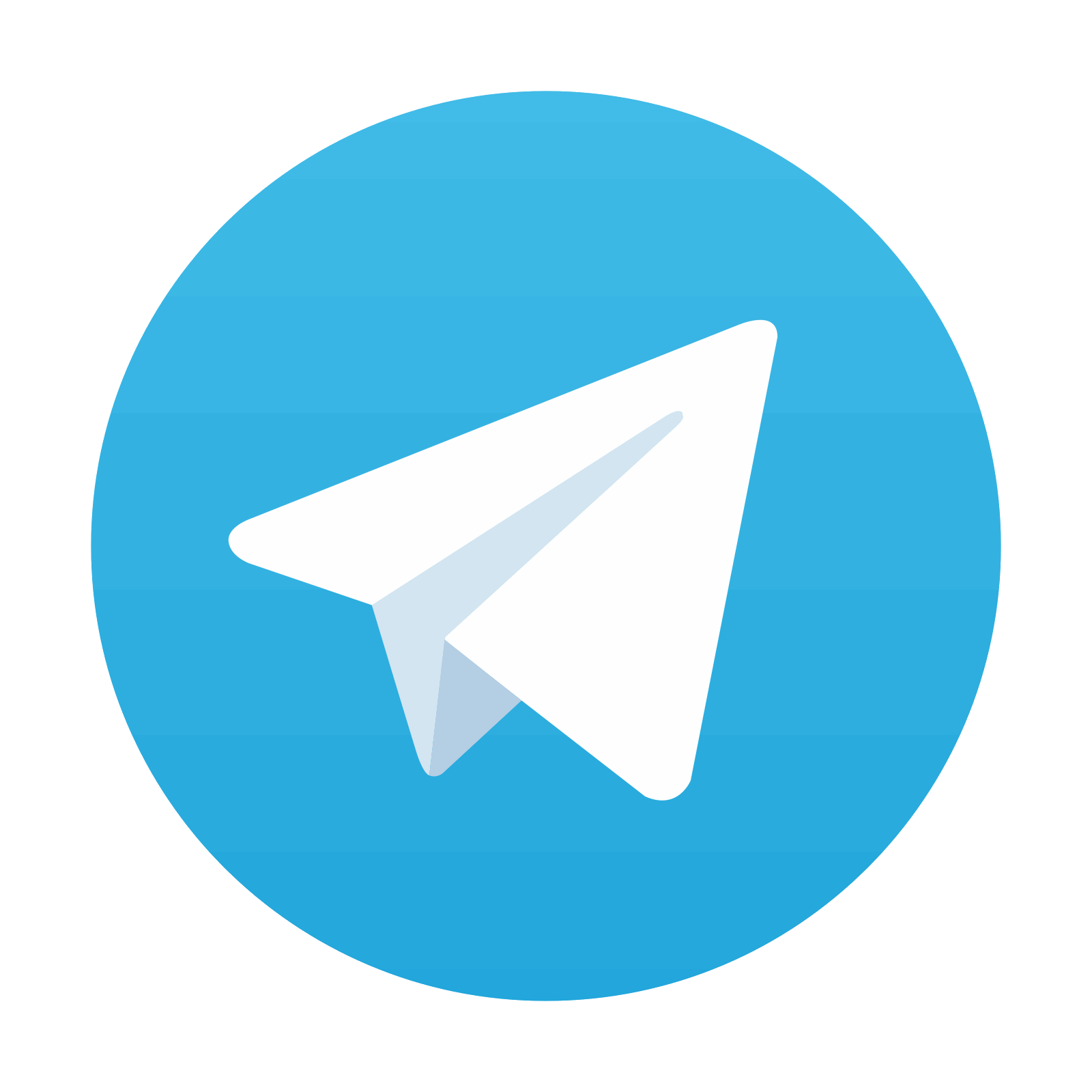
Stay updated, free articles. Join our Telegram channel

Full access? Get Clinical Tree
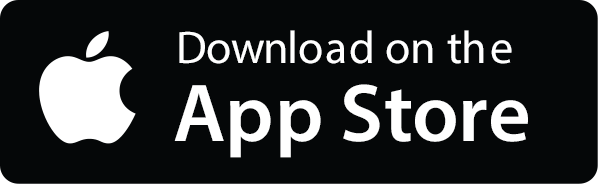
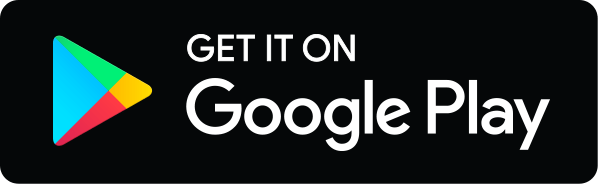