Fig. 18.1
Kidney function analysis by black box method
By comparing these modified kidney input and output proteomes, this work first aims to find which proteins are blocked or permitted to pass through and which proteins are secreted or shed from the kidney. Because these different protein handling pathways in the kidney are closely related to the form and size of individual proteins, the experimental molecular weights (MWs) of proteins are therefore important for understanding the mechanisms of protein handling by the kidney. Then, the experimental MWs of the proteins in plasma and urine are compared. For different proteins, the associations between the quantitative changes from plasma proteome to urine proteome and their MWs were also investigated.
18.2 Kidney Input Proteome and Output Proteome
The human plasma proteins were collected from the plasma proteome project (PPP) initiated by HUPO, who published a high confidence (FDR 1 %) list of 1,929 identified proteins (http://www.mcponline.org/content/suppl/2011/06/01/mcp.M110.006353.DC1/mcp.M110.006353-4.xls) including Phase I and II data, in June 2011 [Farrah et al. (http://www.mcponline.org/content/early/2011/06/01/mcp.M110.006353.long)]. The human urine proteins were collected from three large-scale datasets of the previous studies [1, 6, 7] and one large-scale dataset identified in our institution (data not published). There are only a limited number of proteins with experimental MWs information published so far in plasma and urine. In this study, the experimental MWs of the proteins were collected from the previous study [4, 8, 10].
The prostate is a secretory gland downstream of the kidney that might secrete prostatic fluid into urine, which would complicate the kidney output proteome. Human prostatic secretion proteins identified by proteomic methods were hence removed from the urine proteome and plasma proteome. A total of 114 human prostatic secretion proteins were acquired from Lin et al. [5]. Other proteins that are possibly incorporated in urine downstream of kidney were temporarily ignored due to limited knowledge at this time.
For easier comparison, the protein identifiers in different datasets were standardized. The UniProt Reference Clusters (UniRef) provide clustered sets of sequences from the UniProt Knowledgebase (http://www.uniprot.org/help/uniprotkb) [including isoforms (http://www.uniprot.org/help/canonical_and_isoforms)] while hiding redundant sequences. UniRef90 was selected as the reference database. All the protein identifiers of different datasets were mapped to UniRef90 cluster using ID mapping tool from UniProt Web site. After protein ID standardization and removal of prostatic secretion proteins, kidney input and output proteome were generated. This resulted in 1,778 non-redundant UniRef90 sequences in the plasma as the kidney input proteome and 6,093 non-redundant UniRef90 sequences in the urine as the kidney output proteome.
18.3 Kidney Function Described in Proteomic Language
The plasma-only subproteome, the plasma-and-urine subproteome, and the urine-only subproteome were generated after comparing the kidney input and output proteomes with the kidney considered as a black box. The function of the kidney can be described in itemized proteomic language as whether a particular protein is blocked, permitted to pass, or secreted/shed from the kidney. These three groups of proteins correspond to the three subproteomes.
18.3.1 Plasma-Only Subproteome
There were 351 non-redundant protein sequences in the plasma-only subproteome. Currently, there was no evidence that they were present in urine based on available proteomic data. Due to the profiling depth of the urine proteome is significantly exceeded that of the plasma proteome, this group of proteins is more reliable. These proteins are supposed to be difficult to pass through the kidney black box. There were only 4 proteins with experimental MWs, which were relatively small proteins. They were P11226 (26.3 kDa), P02745 (26.3 kDa), P20742 (51.6 kDa), and Q14289 (65.1 kDa). The theoretical MWs were in a range from 3 kDa to more than 400 kDa, while the theoretical PIs were in a range from 4 to 11.6. According to estimated concentration of the proteins in plasma provided by plasma proteome dataset, there were many moderate- or even high-abundant proteins that were present only in plasma but not in urine. Particularly, there were some high-abundant Ig proteins present only in plasma. This group included proteins that could not be filtered at the glomerular capillaries or filtered but reabsorbed completely back into the blood from the tubules.
18.3.2 Plasma-and-Urine Subproteome
There were 1,424 non-redundant protein sequences that existed in both plasma and urine. They may pass the kidney black box in various forms. There were only 15 proteins with experimental MW information available from both plasma and urine, which range from 11 to 133 kDa in plasma and 11 to 77 kDa in urine. Comparing their MWs in plasma and in urine, six proteins were within 20 % variation, suggesting that they may pass through the kidney in an intact form; four had MWs 20 % higher in plasma than in urine, and five had MWs 20 % lower in plasma than in urine. These differences reflect functions of the kidney. In addition to one protein whose theoretical MW was 3,816 kDa, the theoretical MWs of the proteins were in a range from 3 kDa to more than 600 kDa, while the theoretical PIs were in a range from 3.6 to 12.
For the kidney considered as a black box, the quantitative changes of different proteins from plasma proteome to urine proteome reflect the kidney protein handling function. Therefore, the ranking order of the plasma and urine proteins sorted by their concentrations in plasma was compared with that in urine. The estimated concentrations of the proteins in plasma were provided by plasma proteome dataset, which were used to generate their ranking order in plasma. Many urine proteins were collected from a large-scale dataset identified in our institution using MASCOT search engine (data not published). The MASCOT search engine has been incorporated the exponentially modified protein abundance index (emPAI), which offers approximate, label-free, relative quantitation of the proteins in a mixture based on protein coverage by peptide matches [2]. Each identified urine protein had an emPAI value, which can be used to approximately estimate the absolute protein contents in urine. The emPAI values of these plasma and urine proteins were extracted from the large-scale dataset. These proteins were sorted from most to least abundant. Proteins not identified in the large-scale dataset were at the end. The ranking order of these proteins approximately represents their abundance in human urine. After comparing their ranking order in plasma with that in urine, we found that the two ranking orders did not correspond well with each other. Many proteins have a significantly changed ranking order when they passed through the kidney. This suggested that the kidney performed different handling functions for the different proteins.
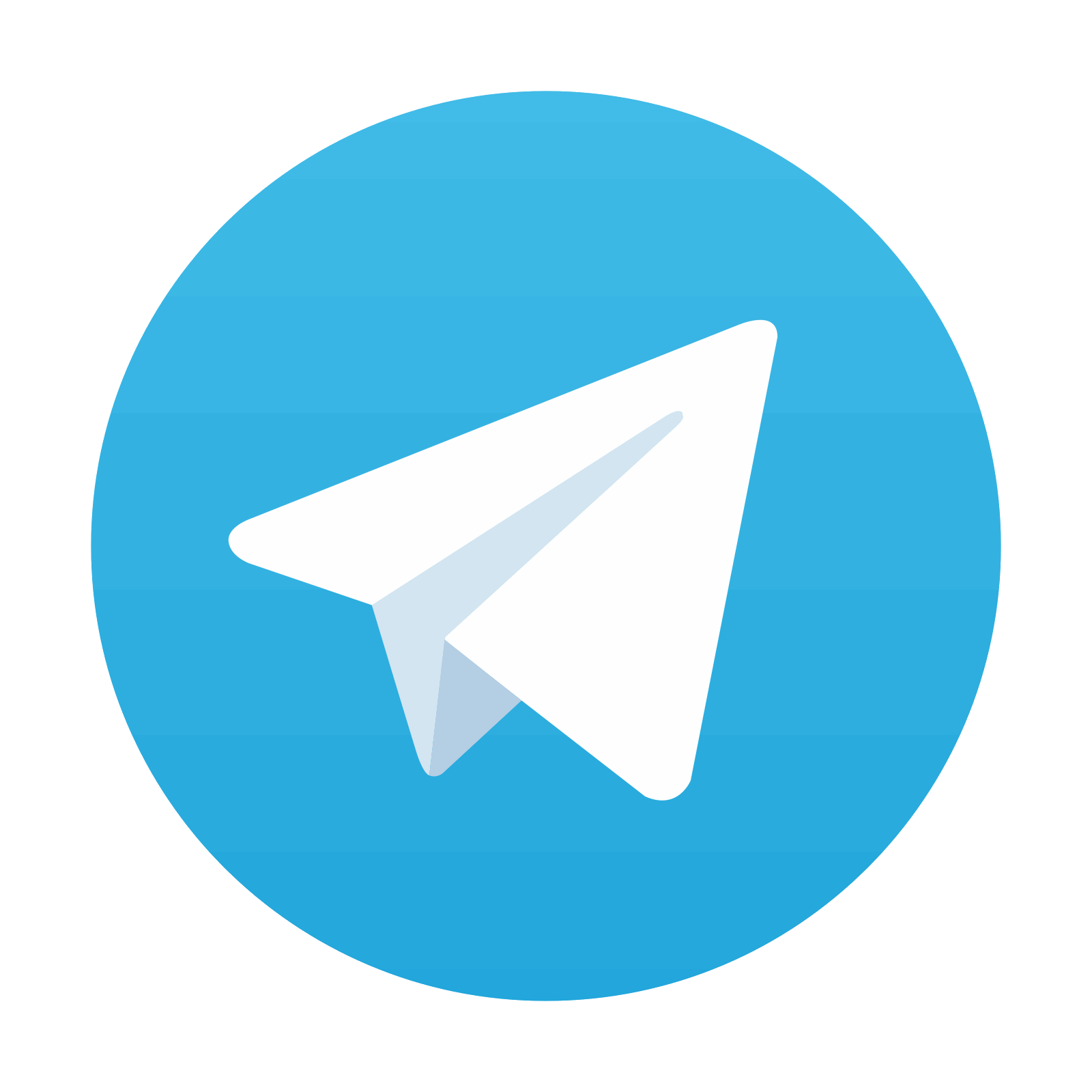
Stay updated, free articles. Join our Telegram channel

Full access? Get Clinical Tree
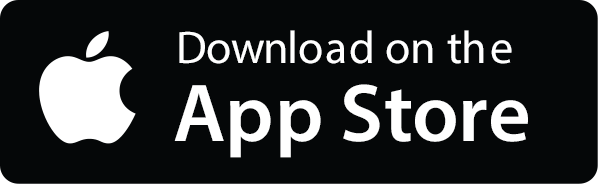
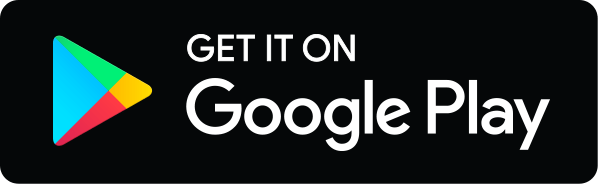