(8.1)
Where [Creat]P is the plasma concentration of creatinine (in μmol/ml) and G the creatinine generation rate in μmol/min.
Thus at steady state a lower GFR will be associated with an higher plasma creatinine following the relationship: GFR α 1/[Creat]P – so that, assuming a steady state has been achieved and that G is constant, a halving of GFR will be accompanied by a doubling of plasma creatinine. This relationship forms the basis of the use of fold increase in creatinine from baseline to define severity of AKI in consensus definitions based on the original RIFLE criteria as this would reflect fold decrease in GFR.
While changes in plasma creatinine define AKI there are significant limitations to its use, particularly in the critically ill [4, 5]. Firstly, use of plasma creatinine as an indirect measure of the GFR is unreliable outside the steady-state, after an acute change in GFR creatinine will rise or fall until achieving a new steady-state where plasma creatinine reflects the new GFR, this process will take a period of time that is dependent on both the magnitude of change in GFR and the underlying creatinine generation rate. With large falls in GFR many days may pass before steady-state is achieved and until then creatinine will underestimate severity of renal dysfunction. Secondly, changes in creatinine production can alter measured plasma creatinine concentration as much as changes in excretion (GFR). For example, creatinine production will fall if there is a reduction in lean body mass, if there is a fall in the dietary intake of creatine, or in the presence of liver disease [6]. As these are all common scenario’s in the intensive care unit and the degree of renal dysfunction may be underestimated in the critically ill if one is solely guided by the creatinine concentration and, similarly, renal recovery after AKI may be significantly overestimated [7, 8]. Importantly, sepsis is associated with reduced creatinine production which may account for the seemingly slow rise in creatinine often observed in patients with septic AKI [4]. However, despite these limitations creatinine is still almost universally employed given the fact that assay is cheap, relatively easy and quick.
8.2.2 Clearance Measurements
Despite the limitations of plasma creatinine, acutely, direct measurement of GFR is not normally performed. GFR can be estimated through the calculation of the clearance of a molecule such as creatinine that is freely filtered from the plasma in the glomerulus and excreted unchanged into the urine (Eq. 8.2)
![$$ \mathrm{G}\mathrm{F}\mathrm{R}\left(\mathrm{ml}/ \min \right)\cong \frac{{\left[\mathrm{Creat}\right]}_{\mathrm{U}}}{{\left[\mathrm{Creat}\right]}_{\mathrm{P}}}\times {Q}_{\mathrm{U}} $$](/wp-content/uploads/2016/07/A313311_1_En_8_Chapter_Equ2.gif)
Where [Creat]U & [Creat]P are the urinary and plasma concentrations of creatinine respectively and Q u is the urine flow rate in ml/min.
![$$ \mathrm{G}\mathrm{F}\mathrm{R}\left(\mathrm{ml}/ \min \right)\cong \frac{{\left[\mathrm{Creat}\right]}_{\mathrm{U}}}{{\left[\mathrm{Creat}\right]}_{\mathrm{P}}}\times {Q}_{\mathrm{U}} $$](/wp-content/uploads/2016/07/A313311_1_En_8_Chapter_Equ2.gif)
(8.2)
Although creatinine clearance is often used to estimate GFR, creatinine is by no means an ideal marker for this purpose. The ideal marker would not only be sensitive and specific in detecting small, early, changes in GFR, but would also not be secreted, metabolised or reabsorbed by tubular cells. Furthermore, it would be easily measured and would not be influenced by exogenous compounds. Tubular secretion of plasma creatinine can cause creatinine clearance to over-estimate GFR by 10–20 % or more, however competing substances for tubular secretion including some drugs can abolish this effect. The difference between Creatinine Clearance and true GFR has become more apparent since the adoption of more accurate Isotope-Dilution Mass-Spectroscopy (IDMS)-traceable laboratory standards and more accurate and precise enzymatic creatinine assays, as previous measurements un-standardised colorimetric assays tended to over-estimate plasma, but not urinary creatinine by detection of non-creatinine plasma chromogens. As an alternative to creatinine exogenous substances without tubular secretion such as inulin, EDTA (ethylenediaminetetraacetic acid) and iohexol are used to measure GFR occasionally, however these are impractical in the everyday acute clinical arena.
8.2.3 Alternatives to Creatinine: Cystatin C and Urea
Urea is a water-soluble low molecular weight by product of protein metabolism, which, like creatinine, exhibits a reciprocal relationship with the GFR. However, as a measure of GFR urea clearance has been superseded principally due to the greater variety of factors which influence both its renal clearance and endogenous production [9]. The main drawback with using urea as a GFR marker is that the rate of renal clearance is not constant. Under steady-state conditions approximately 50 % of urea is reabsorbed by proximal renal tubular cells so that the urea clearance is around 50 % of GFR, however, in hypovolaemic states, enhanced tubular reabsorption of sodium and water together accompanied by urea may decrease urea clearance as a proportion of GFR giving rise to a misleading disproportionate rise in the observed urea concentration. Conversely in advanced chronic or acute kidney disease, or in the presence of diuretic agents, urea clearance may rise as a proportion of GFR, so that increase in urea concentration could somewhat blunted. Urea production has also highly variable rates as these may be increased such as in high protein intake, catabolic states and gastrointestinal haemorrhage, but may also be reduced in acute or chronic malnutrition and liver disease. Therefore, plasma urea and urea clearance is not recommended for GFR estimation particularly under non-steady state conditions.
Cystatin C is a low molecular weight cysteine proteinase inhibitor synthesised at a relatively constant rate by all nucleated cells and released into plasma [10]. The main catabolic site of the Cystatin C are the proximal renal tubular cells following the almost complete (>99 %) filtration by the glomerulus [11]. Therefore, little or no Cystatin C is present in the urine. As a consequence, the urinary clearance of Cystatin C cannot be determined but any fall in GFR correlates well with a rise in serum Cystatin C concentration and excellent correlation with radionuclide derived measurements of GFR [12]. However the lack of a standardised method for measurement has prevented widespread adoption into clinical practice. This is coupled with the observation that the accuracy of measurement is affected by older age, sex, smoking status and raised CRP levels as well as abnormal thyroid function and the use of corticosteroids. Nevertheless, confounders of Cystatin C are likely to be less marked than those of creatinine during acute illness and availability of a standardised assay at an acceptable cost may lead to more widespread uptake of Cystatin c measurement in the future.
8.2.4 Mathematical Estimation of GFR
Several equations have been developed and validated for the estimation of the GFR or Creatinine Clearance. These include the Cockcroft-Gault equation, the four variable MDRD (Modification of Diet in Renal Disease Study Group equations Study Equation), the CKD-EPI Creatinine Equation, the CKD-EPI Cystatin C Equation and the CKD-EPI Creatinine-Cystatin C Equation. Many laboratories now quote an eGFR value together with serum creatinine. Although useful it must be remembered that, these estimated GFRs are derived values and not measured variables. At heart these equations are dependent on the reciprocal relationship between GFR and plasma creatinine at steady state transforming this into a direct GFR estimate by providing what is essentially an estimate of creatinine generation normalised to body surface area for individuals of a given age, sex and racial background. They are thus dependent on a patient firstly, being in steady state between GFR and plasma creatinine and, secondly, having a typical creatinine production for the outpatient populations used to generate these estimates. As neither of these are the case in most of critically ill patients, these formulae are not recommended for use in the acute setting, but rather as a tool for managing chronic kidney disease.
Key Messages
The basis of use of creatinine for assessment of renal function relies on its rate of excretion being approximately proportional to GFR.
Creatinine levels will, initially, significantly underestimate the severity of renal dysfunction following a significant fall in GFR until steady-state is achieved.
Changes in creatinine production can alter measured plasma creatinine concentration as much as changes in excretion and this is of particular relevance in the critically ill.
Cystatin C, a low molecular weight cysteine proteinase inhibitor is synthesised at a relatively constant rate by all nucleated cells and almost exclusively filtered at the glomerulus.
Although confounders of Cystatin measurement are probably less than creatinine, there is at present a lack of a standardised Cystatin C method of measurement.
8.3 Urinalysis in AKI
8.3.1 Urine Analysis
Standard urine analysis involves assessment of urine colour, pH, specific gravity and the presence of glycosuria and/or proteinuria. Further information may be determined from microscopy of the urine. Under normal conditions urine colour is dependant on concentration however under certain pathological states urine colour may aid in diagnosis. For example, a red supernatant may point to myoglobulinaemia or haemoglobinuria and hence lead to further focused investigation. With regard to the intensive care unit, green urine may be observed as a consequence of intravenous propofolol infusion. Although pH and specific gravity may be of use in stable patients, they add little to diagnosis within the ICU. However, the presence of haematuria particularly in the presence of proteinuria should alert the clinician to the possibility of parenchymal renal disease. Indeed the presence of proteinuria may complicate AKI particularly in the presence of sepsis although this is often tubular in origin reflecting incomplete reabsorption of low molecular weight proteins by proximal tubular cells. Glomerular proteinuria reflects leakage of larger molecular weight proteins such as albumin across the glomerular capillary wall and this may reflect acute injury such as glomerulonephritis but may also have been present prior to admission [13, 14]. The presence of premorbid proteinuria has significant prognostic implications. For all these reasons, a simple urinary dipstick analysis should be undertaken in all patients and where necessary proteinuria may be quantified either by timed collection or through a urinary protein: creatinine ratio.
8.3.2 Urine Microscopy
The assessment of the urinary sediment is often overlooked in the intensive care unit but can yield important information regarding the cause of the AKI. For example, frank haematuria may suggest underlying renal tract pathology whereas the presence of dysmorphic red cells imply glomerular injury. Similarly, casts, which appear cylindrical in nature due to the development within the renal tubule, may signify significant injury. Cellular casts consisting of either epithelial cells, erythrocytes or leukocytes are associated with significant renal damage. White cell casts are seen both in infection and with tubulointerstitial damage whereas red cell casts are seen in glomerulonephritis in the presence of vasculitis. Epithelial cell casts reflect cell necrosis and desquamation and classically are thought to reflect acute tubular cell necrosis. Although these findings have been described, they are not routinely employed due to the lack of consistency between the findings seen on urinary microscopy and correlation with biochemical values. Several attempts have been made to correlate findings with diagnosis and prediction of outcome but so far these have proved far from perfect and are rarely employed in clinical practice [15]. Crystals may also be seen in the urine, though are rarely of significance in the critically ill.
Key Messages
Simple urinary dipstick analysis should be undertaken in all patients where possible.
Proteinuria may complicate AKI particularly in the presence of sepsis.
The presence of premorbid proteinuria has significant prognostic implications.
Haematuria particularly in the presence of proteinuria should alert the clinician to the possibility of parenchymal renal disease.
8.4 Urine Chemistry
There are many potential tests which may be performed on the urine but in practice few are applied to the patient with AKI. Principally these involve the fractional excretion of sodium and urea as well as urinary estimation of creatinine. Although historically measures such as the urine:plasma creatinine ratio and the serum urea:creatinine ratio have been used to try to differentiate between AKI secondary to volume deplete states and intrinsic disease results are inconsistent and these techniques are now rarely employed. In fact while elevated urea proportional to creatinine could reflect dehydration and reversible renal dysfunction, in critical illness, reduction in creatinine generation and increase in urea generation during active muscle wasting may lead to elevated urea:creatinine ratios that are in fact associated with more severe illness and adverse outcomes [16], illustrating the difficulty in meaningfully interpreting these measurements.
8.4.1 Urinary Sodium
The urinary sodium is used by some as an indicator of a ‘pre-renal’ aetiology for renal dysfunction given the avid sodium reabsorption by the renal tubules in volume deplete states. Thus a urinary sodium value of 10–20 mmol/l is suggestive of a haemodynamically reversible cause of renal dysfunction whereas a value of >40 mmol/l is classically referred to as being indicative of established, not rapidly reversible, tubular injury (Table 8.1). However, despite the dogma that such biochemical values can translate directly into a diagnostic test for a pathological diagnosis, there is little to substantiate this in the literature particularly within the critically ill. Indeed, the currently available data suggests that measurement of the urinary sodium has little or no diagnostic or prognostic utility within this population [17].
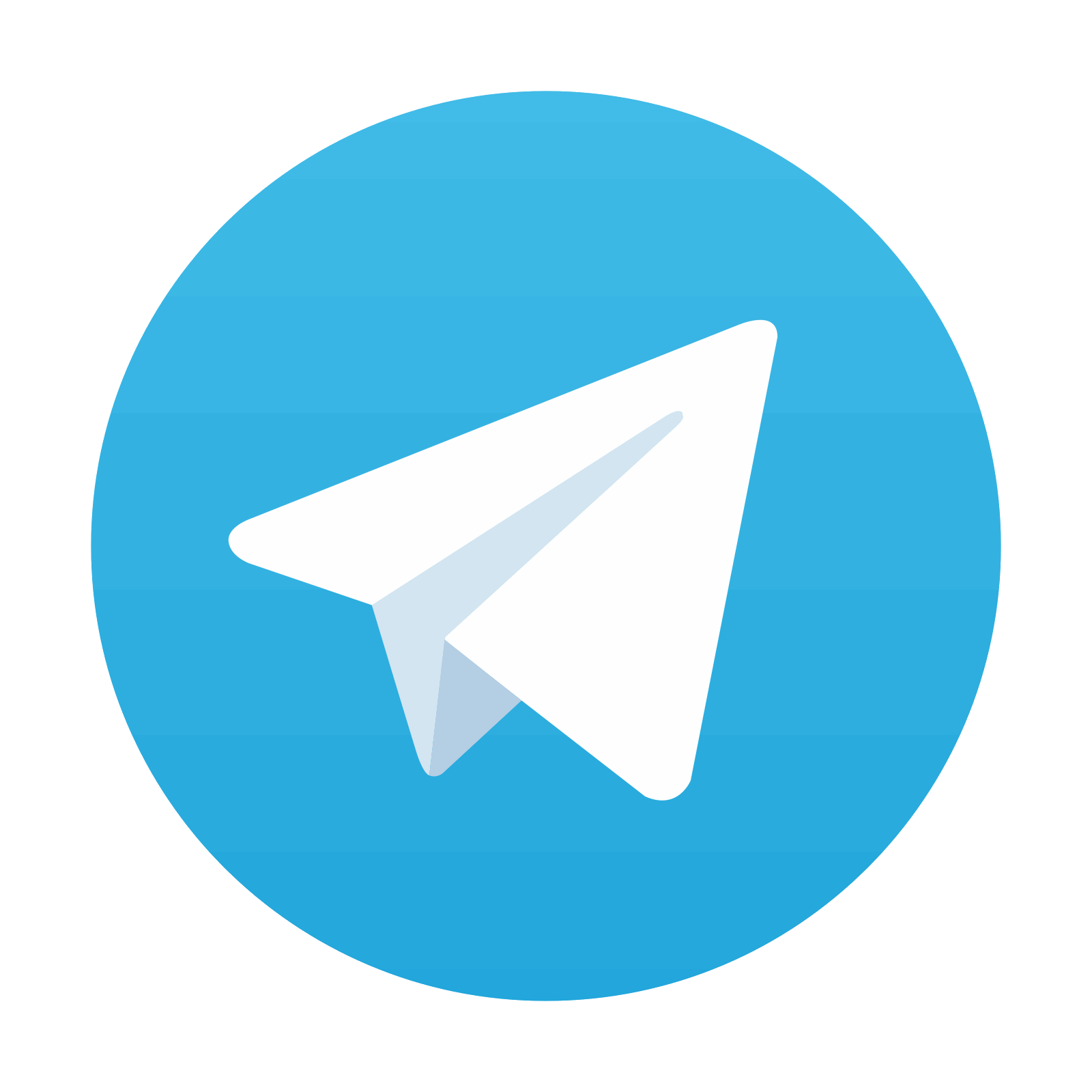
Table 8.1
Classical urinary indices in AKI due to pre-renal causes and intrinsic disease
< div class='tao-gold-member'>
Only gold members can continue reading. Log In or Register a > to continue
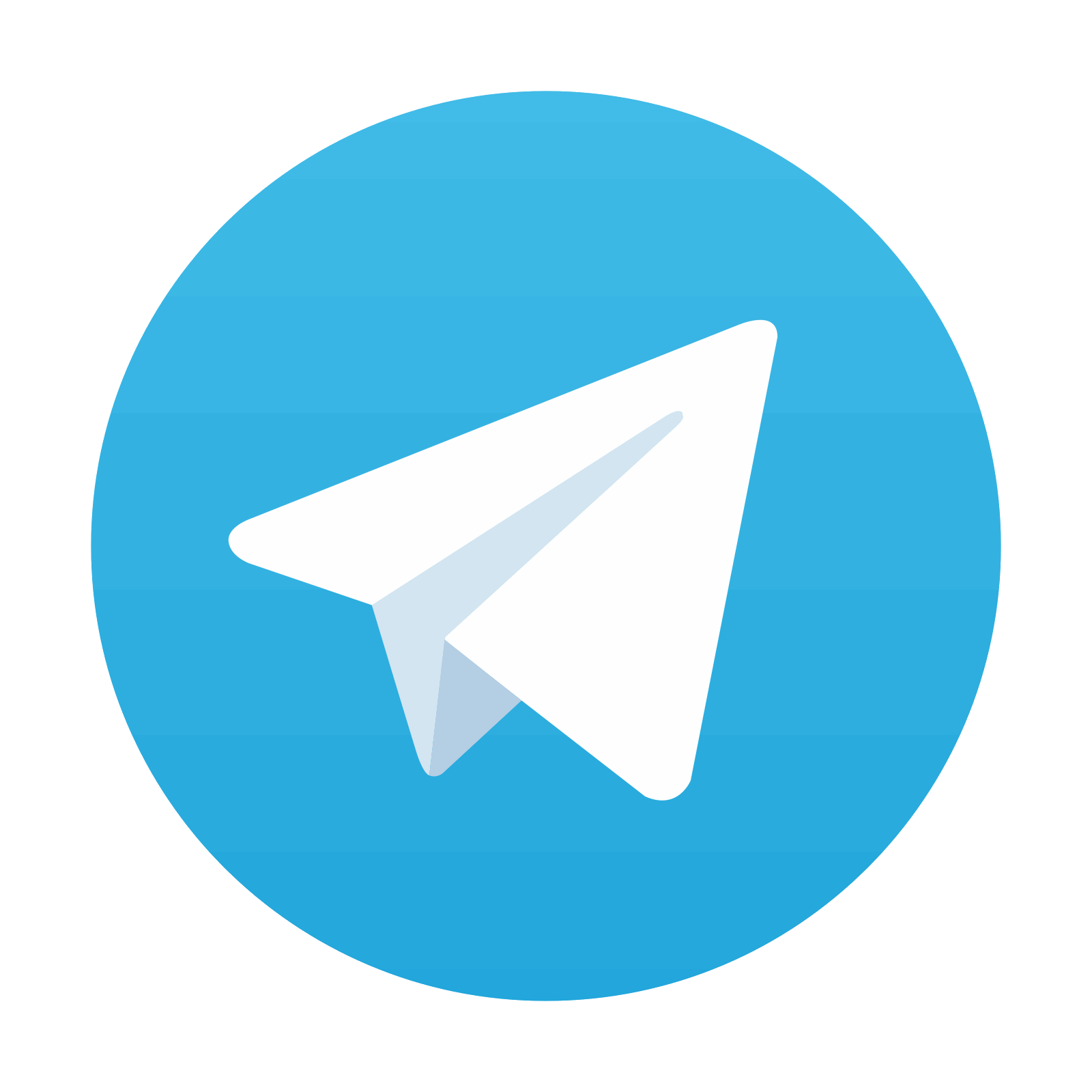
Stay updated, free articles. Join our Telegram channel

Full access? Get Clinical Tree
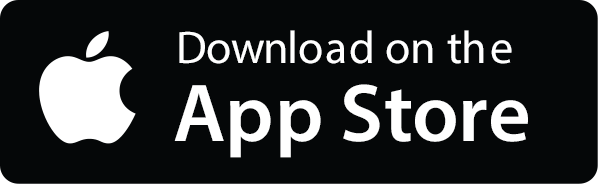
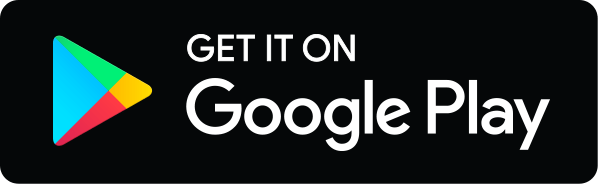
