Type of genetic aberration
Gene
iCCA
eCCA
Copy number alterations
MYC
MDM2
EGFR
KRAS
CCND1/CCND3
AKT3
FGFR1/3
Deletions
CDKN2A
UTY
KDM5D
Fusion genes
FGFR2
6–45%
0%
ATP1B
2.5%
0%
Mutations
KRAS
3–18%
12–21%
PIK3CA/PIK3CA/
PIK3C2G/PIK3C2A/
PTEN
22%
IDH1/IDH2
6–22%
0%
ERBB2
TP53
6–7%
7–14% dCCA > pCCA
ARID1A/ARID2
4–14%
5–7%
PBMR1
8–13%
0%
BAP1
12–22%
0–3%
SMAD4
0%
21%
TGFBR2
3%
FGFR2
8–13%
0%
CDKN2A
3%
ARAF
11%
EGFR
4%
1%
Epigenetic regulators
(total 29%)
IDH1/IDH2
BAP1
TET1/2/3
MLL2/3
10.3 Pathology and Cells of Origin
Based upon their macroscopic growth pattern, CCA may be classified as mass-forming, periductal-infiltrating, or intraductal-papillary. iCCA is predominantly mass-forming, while pCCA is typically periductal-infiltrating. Histopathologically, 90–95% of CCAs are adenocarcinomas of moderate to poor differentiation, with characteristic mucin expression and highly desmoplastic stroma [9].
CCA is thought to originate from transformed cholangiocytes. This concept received recently support by a cholangiocyte-lineage tracing model in which p53-knockout mice were treated with thioacetamide resulting in labeled-cholangiocyte-positive, NOTCH1-overexpressing CCA tumors [12]. Interestingly, certain primary liver cancers such as cholangiocellular carcinoma, cholangiocarcinoma-like hepatocellular carcinoma (HCC), and mixed CCA-HCC are characterized by features of both tumor types possibly suggestive of common cells of origin [1, 18, 31]. Indeed, there is also evidence that CCA might originate from stem cells, hepatic progenitor cells, or transdifferentiated hepatocytes. Hepatic progenitor cells have been described in the intrahepatic biliary ductules, the canals of Hering, and peribiliary glands (PBGs) (Fig. 10.1a, b) ([5, 6] #153). These cells have the potential to differentiate in hepatocytes or cholangiocytes, and their differentiation into cholangiocytes underlies regulation by EGFR-induced NOTCH1 [16]. Cells with stem cell features have been described in extramural PBGs of large intrahepatic bile ducts and extrahepatic ducts. In PSC, PBG areas are increased, and they contain inflammatory infiltrates and increased numbers of cells with stem cell features, and cell proliferation is markedly increased [6]. Biliary dysplastic lesions in PSC are found in intrahepatic large ducts, extrahepatic ducts, and PBGs, and the number of cells with stem cell features is significantly increased in these dysplastic lesions [6].


Fig. 10.1
Peribiliary glands. (a) Schematic of peribiliary glands (PBGs) including intramural (arrow heads) and extramural (arrows); L bile duct lumen (Nakanuma et al. 1994). (b) Immunohistochemistry for CK7 of normal hepatic parenchyma (left) and hepatic parenchyma in primary sclerosing cholangitis (PSC). Arrows indicate areas with PBGs (Carpino et al. [6])
The concept of transdifferentiation is supported by a recent study in which H-Ras and SV40 large T-antigen-transformed hepatic stem cells, hepatoblasts, and hepatocytes gave rise to primary liver cancers with features of HCC and CCA. However, CCA formation was predominantly observed with transformed hepatoblasts [13]. Further, a recent study reported cholangiocarcinogenesis after intrahepatic NOTCH1 and AKT overexpression in a mouse model [11].
In summary, the controversy about the cellular origin remains. However, important insights in the carcinogenesis were gained from studies evaluating the cellular origin of CCA. Interestingly, a common finding of these studies was the upregulation of EGFR and NOTCH1 signaling independent of the cellular origin. These findings provide the rationale for an EGFR- or NOTCH1-targeted chemoprevention strategy in patients at risk for cholangiocarcinogenesis. Indeed, Mayo Clinic recently completed a clinical phase 1 trial evaluating erlotinib in PSC patients with biliary epithelial trisomy 7 (clinicaltrials.gov, NCT00955149).
10.4 Pathogenesis
Inflammation and cholestasis are key factors in cholangiocarcinogenesis. Proinflammatory cytokines (i.e., IL-6) activate inducible nitric oxide synthase (iNOS) resulting in excess nitric oxide that mediates oxidative DNA damage, inhibition of DNA repair enzymes, and expression of cyclooxygenase-2 (COX-2). Further, iNOS has been shown to upregulate NOTCH1 in cholangiocytes [14]. Proinflammatory pathways downregulate hepatobiliary transporters, thereby contributing to cholestasis [19]. Bile acids and oxysterols activate EGFR and enhance COX-2 expression [33]. COX-2 dysregulates CCA growth and apoptosis resistance and positively regulates pro-oncogenic signaling pathways such as HGF, IL-6, and EGFR.
Next-generation sequencing identified somatic mutations in oncogenes (i.e., KRAS), tumor suppressor genes (i.e., TP53, SMAD4), and chromatin-modifying genes (i.e., ARID1A, BAP1, PBMR1) in CCA [5, 8, 15]. These studies have also shown the distinct mutational landscape of different etiologies and anatomic locations [2, 5, 8, 15]. KRAS mutations are more common in pCCA (22–53%) than iCCA (9–17%), while IDH1/2 mutations are more characteristic of iCCA [2, 8]. Mutant IDH1/2 (isocitrate dehydrogenase) inhibits hepatocyte but not biliary differentiation and causes expansion of hepatic progenitor cells, resulting in iCCA formation in genetic mouse models [24]. Liver fluke-associated CCA is more commonly associated with mutations of TP53 and SMAD4, while BAP1 and IDH1/2 mutations are more frequent in non-liver fluke-associated CCA [5, 8]. Mutations of genes coding for components of oncogenic pathways such as PI3KCA and MET have been described in iCCA and pCCA [28]. Recently, gene rearrangements resulting in oncogenic fibroblast growth factor 2 (FGFR2) fusion proteins were identified in up to 45% of iCCA patients [6, 32]. Also non-genomic upregulation of EGFR, HER2, and MET was found, especially in patients with poor outcomes [2]. Whole-genome expression profiling confirmed activation of pathway driving proliferation (i.e., EGF, RAS, AKT, MET), angiogenesis (i.e., VEGFR, PDGFR), and inflammation (i.e., IL-6) [4].
Receptor tyrosine kinases such as IL-6 receptor, c-MET, and the EGFR family members ERBB2 and ERBB1 are key signaling pathways in cholangiocarcinogenesis. CCA cells and cancer-associated fibroblasts (CAFs) express and secrete cytokines and other mitogenic growth factors (i.e., IL-6, hepatocyte growth factor [HGF]) with subsequent auto- and paracrine stimulation of their cognate receptors. Receptor overexpression (i.e., IL-6R, c-MET, and EGFR), inactivation of negative feedback mechanisms, and transactivation between receptors (i.e., c-MET/EGFR, COX-2/IL-6) further contribute to constitutive pathway activation. Aberrant activation of these receptor tyrosine kinases causes constitutive activation of downstream signaling cascades (i.e., JAK/STAT3, PI3K/Akt, ERK1/2, and p38MAPK) resulting in dysregulation of cell senescence, cell cycle regulation and proliferation, and apoptosis.
10.5 Future Treatment Directions
Traditionally considered chemotherapy resistant, the phase III randomized controlled ABC-02 trial reported a 6-month survival benefit in CCA patients treated with gemcitabine/cisplatin combination therapy versus gemcitabine monotherapy [27]. A recent phase III randomized controlled trial showed higher objective response rates (31% vs. 14%, p = 0.004) and longer progression-free survival (5.9 months vs. 3.0 months, p = 0.049) through the addition of the EGFR inhibitor erlotinib to gemcitabine/cisplatin [20]. The data indicate the potential of molecular targeting to improve outcomes in CCA.
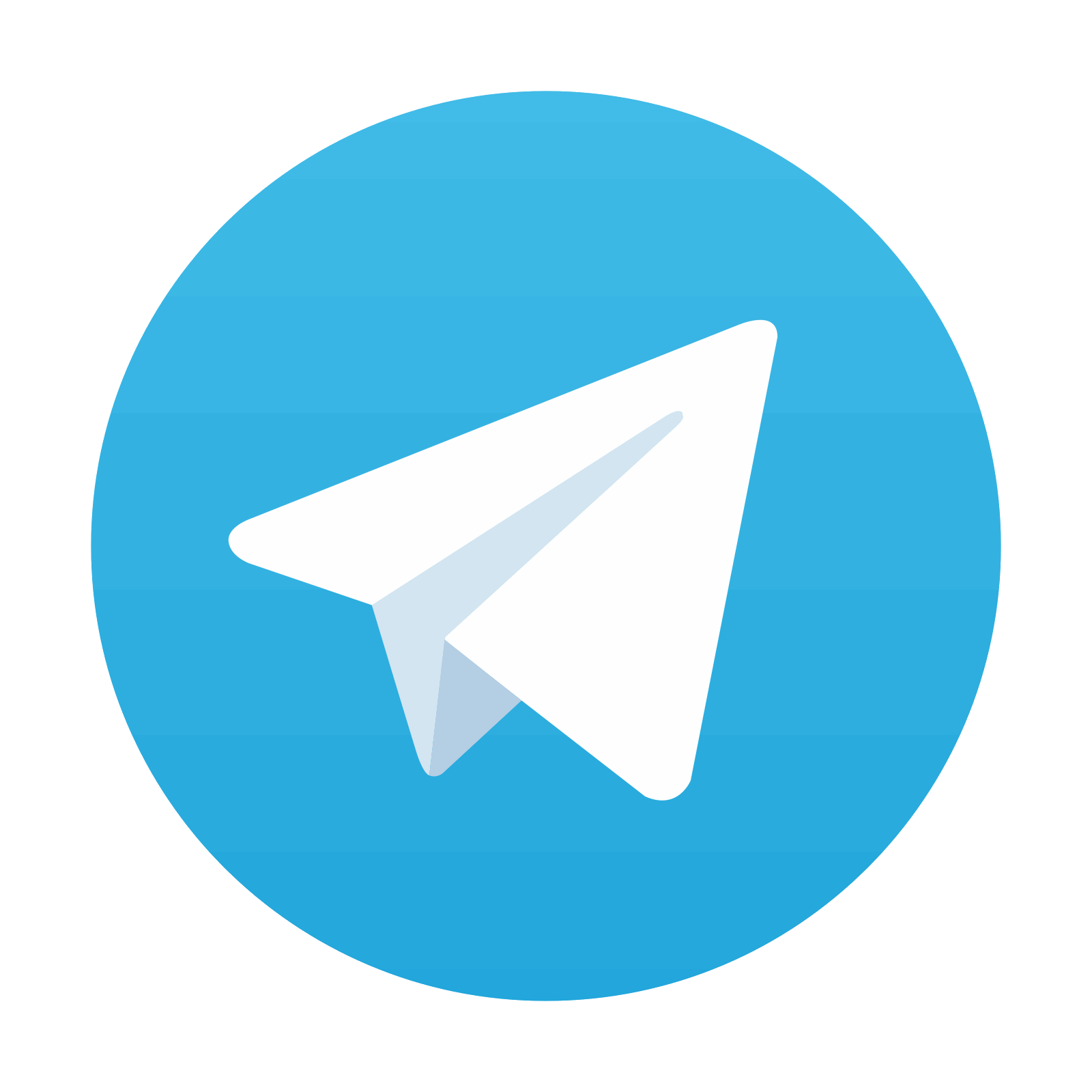
Stay updated, free articles. Join our Telegram channel

Full access? Get Clinical Tree
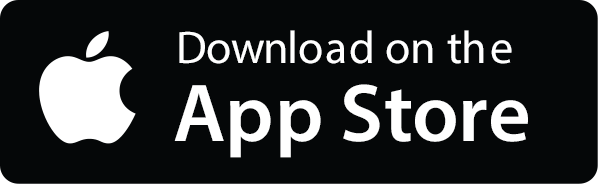
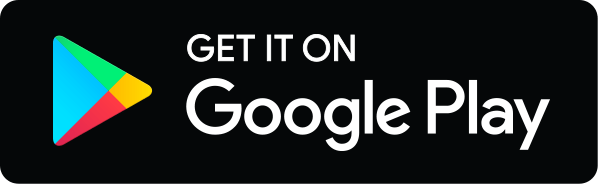