Abstract
It has been known for centuries that ingestion of certain foods and for decades that alteration of the gut microbiome by antibiotics or probiotics can have profound effects on physiology and pathophysiology. In the past decade, the mechanisms underlying these observations has become apparent with the discovery of G protein-coupled receptors for components of the gut lumen, including exogenously ingested and endogenously produced substances and bacterial metabolites. Examples of these substances include short- and long-chain fatty acids, bile acids, polyamines, protons, bitter tastants, terpinoids, and bacterial endotoxin. Each of these compounds or class of compounds have conjugate receptors expressed on key signal transducing cells such as enteroendocrine cells or enteric nerves that release hormones or activate neural functions in response to receptor activation by these luminal ligands.
In this chapter, we will describe each class of receptor, describing its ligands and importantly how each contributes to normal and abnormal physiology such as the regulation of insulin relapse, gut motility, gut mucosal proliferation, and mucosal anion secretion. Understanding the function of these receptors should inform the design of molecules that mimic the response to luminal bioactive molecules which in turn regulate neurohormonal mechanisms, with the aim of discovering new therapies for metabolic disease, inflammatory activation, and diarrhea as examples.
Keywords
Bacterial metabolites, G protein-coupled receptors, Short-chain fatty acids, Bile acids, Enteroendocrine cells, Enteric nerves, De-orphanized receptors, Bitter tastants, Lipopolysaccharide, Guanylyl cyclase, TRP channels
28.1
Introduction
For centuries, it has been known that ingestion of foods rich in indigestible carbohydrates, sugars, bitter substances, and fats elicits specific cellular responses, such as releasing hormones that alter gastrointestinal (GI) function. It has been only in the past few years that the mechanisms underlying these host responses to ingested, endogenous, and microbially produced substances have been unraveled. In this chapter, we will describe the known biologic sensors for these substances termed “chemosensors,” providing data regarding their possible biologic functions, in an effort to gain further understanding of how the host responds to these biologic signals.
We have focused on the colon since the colon appears to be the main locus of the gut microbiota and luminal chemosensors. The human colon, comparable with the rodent cecum or ruminant forestomach, is the principal site in which commensal bacteria ferment luminal compounds, absorbing calories, and essential nutrients downstream of their usual small intestinal absorption sites. The colonic epithelium is also densely populated with enteroendocrine cells (EECs) that transduce luminal bioactive compounds into neurohormonal signals. Interestingly, the rectal mucosa has the densest EEC population with a predominance of glucagon-like peptide (GLP)-1, GLP-2, peptide-tyrosine-tyrosine (PYY), and 5-hyroxytryptamine (5-HT) containing cells that contribute to GI physiology and metabolic control through neurohormonal pathways. In this chapter, we will review the significance of colonic region-specific luminal bioactive compounds, their receptors, and how receptor activation affects GI function.
28.2
Gut Microbiota
The mammalian intestine is home to trillions of microbes, including more than 1000 species of bacteria, archaea, protozoa, fungi, and viruses. The gut microflora is an ecosystem that is recognized as alternative organ interacting with the host digestive and immune systems through signaling pathways. From a genetic viewpoint, the intestinal lumen includes microbial genes that are estimated to exceed the number of host genes by 100- to 400-fold, indicating that a large variety of known and unknown metabolites are produced. Microbial composition and diversity is determined by species richness and other measures, with “symbiosis” used to denote the microbial composition associated with health, and “dysbiosis” used when the diversity is altered. Dysbiosis, present in a variety of diseases, can be modified with supplementation of specific beneficial bacteria termed probiotics or by nonabsorbable antibiotics or fecal microbiota transplantation from healthy subjects. Alteration of gut microflora in this fashion significantly improves the course of inflammatory bowel disease (IBD), infectious diarrhea due to Clostridium difficile , altered insulin sensitivity, and possibly irritable bowel syndrome (IBS). In addition to GI diseases, dysbiosis has been recently implicated in the pathogenesis of obesity and central nervous disorders, such as autism and Parkinson’s disease.
Human fecal production is ~ 128 g/day, increased by high dietary fiber intake. The chemical composition and pH of the fecal output are influenced by diet, with the major organic component (25%–54% of dry solid) of feces derived from bacterial biomass. Gas chromatography-mass spectrometry analysis detected more than 700 volatile organic compounds (VOCs) from human feces, which are derived from the diet, shed epithelial cells, and microbial metabolism of undigested foodstuffs. The composition of luminal VOCs is altered in diseases such as IBS, IBD, colorectal cancer, and autism, implicating that the pathogenesis of these diseases is associated with dysbiosis. Matsumoto et al. developed a novel technique for quantifying colonic luminal metabolites (metabolome) using capillary electrophoresis with time-of-flight mass spectrometry (CE-TOFMS) to compare germ-free (GF) and conventionalized GF mice, which were intragastrically inoculated with fecal suspension obtained from specific pathogen free (SPF) mice. Their method identified 179 metabolites including 77 metabolites that predominate in conventionalized mice compared with GF mice. The prominent anions are short-chain fatty acids (SCFAs) such as acetic, propionic, lactic, and butyric acids at total ~ 100 mM, and cations are polyamines (PAs) such as putrescine, spermine, and spermidine in sub-mM concentrations. The luminal metabolites include signaling and bioactive molecules such as γ-amino butyric acid (GABA), prostaglandin (PG) E 2 , β-alanine, cadaverine, putrescine, 5-HT, tryptamine, tyramine, ornithine, and xanthine. These bacterial metabolites could influence host physiology, although some could be released from the colonic mucosa in response to microbial activity. Clarifying colonic chemosensing mechanisms for detecting and responding to luminal bioactive molecules emphasizes the importance of dysbiosis in the pathogenesis of disease.
28.3
Sensor Cells in the Colonic Mucosa
A variety of chemosensors such as G protein-coupled receptors (GPCRs) and transient receptor potential channels (TRPs) are expressed in the colonic epithelial cells, including enteroendocrine, tuft, goblet, and columnar cells ( Fig. 28.1 ). Although GPCRs are primarily cell membrane receptors usually present on the apical membrane, many are detected in the cytosol by immunohistochemistry, either an artifact or due to receptor internalization after stimulation. Overall, most colonic chemosensing GPCRs reported are “promiscuous” sensors, activated by ligands at μM-mM concentrations, differing from hormone receptors tuned at nM concentrations of very selective ligands. The expression level of some chemosensing GPCRs and their downstream transmitters is altered within a few weeks by luminal environmental changes such as altered dietary components and microbial activity. This dynamism suggests a potential value of GPCRs and sensory cells as diagnostic markers and as therapeutic targets. In addition to the epithelial cells, subepithelial tissues that include immune cells and nerve fibers possess the same sensor molecules, contributing to the communication between host and microbiome ( Fig. 28.1 ). Since those cells have no direct contact with lumen, detecting bioactive molecules that are transported across the gut epithelium, the mechanism, by which these molecules are transported across the gut, is of importance.

All types of epithelial cells differentiate from common stem cells, which are located at the bottom of the crypts, and most differentiated cells migrate to the surface epithelium. The turnover time of epithelial cells in the colon differs between cell types. For example, the turnover time of the two major types, columnar and goblet cells, is 4.6 days, while that of tuft cells is 8.2 days, and EECs is 23.3 days in mice. Columnar epithelial cells (enterocytes) and mucus-secreting goblet cells almost fill the colonic epithelium. Colonic crypts consist primarily of goblet cells, whereas the surface epithelium is composed mostly of columnar cells, indicating the possibility that goblet cells transform columnar cells, the latter which are responsible for most of the transcellular transport of ions with accompanying fluid secretion and absorption that occurs in crypts and in the surface epithelium.
Membranous epithelial or microfold (M) cells mostly occurring in solitary and aggregated lymphoid follicles throughout the large intestine are specialized in transporting luminal macromolecules and bacteria. Luminal antigens are taken up by M cells through phagocytosis and are caught by dendritic cells and lymphocytes wandering in subepithelial area; this sampling is an important function of mucosal immune system. M cell morphology and lectin binding property in the cecal patch differ from those in Peyer’s patches, indicating that the surface glycoconjugate pattern of M cells differs between the small and large intestine; their target and function might vary according to intestinal regions.
The EECs are “intestinal taste cells,” a type of “paraneuron” proposed by Fujita, due to their morphological and biological features that are similar to neurons including secretory granules and transmitter release in response to distinct stimuli. The density of EECs differs among intestinal regions (1%–5%), the highest concentrations are present in the human duodenum and rectum, suggesting that even in the rectum, the “tail end” of the intestine, chemosensing is important. The morphology and gene expression profile of colonic EEC differ from those in the small intestine: colonic EEC usually possess a long basolateral rootlet, and the transmitters are less varied than small intestinal EEC. One of the major populations of colonic EEC termed L cells due to their large secretory granules, which store the hormones PYY, GLP-1, and GLP-2 ( Fig. 28.2 ). These hormones enhance energy absorption and storage via distinct pathways: PYY slows GI transit, GLP-1 potentiates glucose-induced insulin secretion, and GLP-2 increases mucosal growth. Since total colonic resection reduces postprandial plasma concentrations of GLP-2, colonic EEC contributes to systemic energy metabolism as an endocrine organ. Colonic L cells express a variety of chemosensors, including gustducin, taste receptor subunits T1R2 and T1R3, sodium-dependent glucose transporter (SGLT)-1, bitter receptor T2R38, bile acid receptor GPBA, and free fatty acid (FFA) receptors FFA1, FFA2, FFA3, FFA4, and GPR119. PYY-containing L cells express pre- and postsynaptic markers and directly link with nerve fibers, implicated in the specific efferent and afferent communication between EEC and the enteric nervous system. Another major class of EEC is 5-HT-producing enterochromaffin (EC) cells. Martin et al. demonstrated that EC cells isolated from murine colon express a variety of sensors for sugars (SGLT1, SGLT3, GLUT1, GLUT2, GLUT5, T1R3), amino acids (GPR92/93, also known as LPA5), and fatty acids (FFA1-4, GPR84, GPR119). The antisecretory hormone somatostatin is produced by distinct small EEC population, which also express FFA3. Exogenous factors regulate the individual expression of EEC; for example, EC cell numbers are decreased by the depletion of dietary fiber, whereas they are increased by the luminal microbiome or by dextran sodium sulfate-treated mucosal inflammation. As a specific example, GLP-1-producing L cells are upregulated by the presence of luminal fermentable dietary fibers. These reports suggest that there are distinct regulatory mechanisms in the differentiation of each EEC type.

Tuft cells (also called as brush, caveolated, multivesicular, and fibrillovesicular cell) are rarely present epithelial cells, marked by doublecortin-like protein kinase 1 (DCLK1) and cytokelatin-18. Although the colonic tuft cell is not well characterized, these cell types are candidate intestinal sensory cells based on the co-expression of taste transduction molecules such as gustducin and TRPM5. Immunohistochemical studies demonstrated that gastroduodenal tuft cells express neuronal nitric oxide synthase (NOS), choline acetyltransferase, cyclooxygenase (COX)-1 and -2, opioids, and uroguanylin, but not chromogranin A, suggestive of a unique transmitter releasing mechanism. Nerves that stain for the pan neuronal marker PGP9.5 + are connect to some tuft cells, suggesting a direct signal transduction pathway from tuft cells to neurons similar to that typical of other EEC. Since the depletion of epithelial DCLK1 decreases epithelial cell proliferation and survival rate after mucosal injury, DCLK1 + tuft cells may maintain epithelial homeostasis and restitution.
Antibacterial proteins, namely α-defensins, are exclusively produced in Paneth cells in the small intestine, and present in the colonic lumen in mice. Defensin-producing Paneth cells are not present in the normal colon; however, the mucosa of colonic adenomas synthesizes α-defensins, decreasing the population of commensal bacteria in patients. This change of α-defensins production sites to adenomatous epithelium may have impact on the composition of the microbiome.
28.4
Short-Chain Fatty Acids
Although the health benefits of dietary fiber intake and fermented foods have been well recognized for decades, the mechanism by which these food components benefit the host has only recently been elucidated, mostly due to the recent de-orphanization of SCFA receptors. In particular, soluble fiber improves bowel habits and improves glycemic control and energy metabolism through gut hormone release, including GLP-1, GLP-2, and PYY. These effects are not present in GF animals that lack luminal microbiota, indicating that microbial metabolites derived from dietary fiber rather than the fiber itself is beneficial.
SCFAs, such as acetate, propionate, and butyrate are among the most important microbial metabolites that interact with host cells. Up to 100 mM SCFAs are produced in the colonic lumen of non-ruminants by bacterial fermentation of dietary components that resist foregut digestion and absorption. Infant feces already contain significant amounts of acetate and lactate, but rarely propionate and butyrate, produced by bacterial fermentation from oligosaccharides contained in breast milk. Human amniotic fluid contains acetate and lactate at concentration 6.9 and 9.7 mM, respectively. These reports suggest that the human intestine is exposed to high concentrations of SCFAs throughout life and even prenatally, and SCFA sensing may be important for the development of the GI tract. Since luminal SCFAs are absorbed by colonic epithelial cells into the submucosa and the systemic circulation, a variety of SCFA signaling pathways are likely involved in acute and long-term physiological responses to luminal bacterial activity.
Luminal SCFAs acutely increase motility and transmural ion transport in rat colon in vivo and in vitro. From studies of isolated colonic segments, the contractile and secretory effects of SCFAs are primarily mediated by neural and epithelia-derived cholinergic pathways. Although isolated colonic EC cells are not directly activated by SCFAs, SCFAs induce peristalsis is accompanied with 5-HT release into the lumen and abolished by de-afferentation in vivo . SCFAs likely influence many physiological functions via local and neuronal pathways. On the other hand, repeated luminal infusion of SCFAs inhibits colonic motility and fluid secretion through PYY release. Furthermore, SCFAs reduce electrical stimulation-evoked neurally mediated contractions of isolated rat colon, which are independent of the presence of mucosa, indicating that SCFA can inversely regulate enteric neural activity. Thus, luminal SCFA sensing pathway acutely accelerates and secondarily reduces colonic motility and secretion, facilitating fermentation of the luminal content and the absorption of luminal SCFA as an additional energy source.
FFA2 and FFA3 were de-orphanized as SCFA selective receptors. Other FFA family members, FFA1 and FFA4 are long-chain fatty acid receptors not activated by SCFA. SCFAs are transported by the monocarboxylate transporter (MCT) and sodium-dependent MCT (SMCT) families, particularly basolateral MCT1 and apical SMCT1 in the large intestinal mucosa.
FFA2 (also known as GPR43) is abundantly expressed in neutrophils and in adipocytes in the GI tract, and is also present in PYY- and GLP-1-producing colonic L cells in human and rodents. Increased luminal SCFA concentrations induced by dietary fiber supplementation upregulates FFA2 expression and GLP-1-containing L cell density. In vivo and organoid studies using FFA2 deficient mice demonstrated that FFA2 activation is selectively involved in PYY, but not GLP-1 production and release into the circulation. Although colonic L cells express FFA2 and FFA3, both selective agonists have no effect on GLP-1 positive cell density in organoids, suggesting that GLP-1 upregulation requires signals different from these generated by epithelial cells. To date, ion secretion or colonic contraction induced by SCFAs has not been accomplished by the use of FFA2 selective agonists.
Experiments with FFA3- (known as GPR41) deficient and reporter protein-expressing mice indicate that GLP-1 and PYY release is mediated by FFA3 ; consistent with the FFA3 localization to L cells. FFA3 is distributed in enteric neurons, autonomic ganglia, and extrinsic afferent neurons, which contribute to GI physiological functions. The activation of FFA3, expressed on cholinergic nerves located in the mucosal and submucosal plexus of rat proximal colon, suppresses nicotinic acetylcholine receptor (nAChR)-mediated anion secretion, possibly through the inhibition of neural ACh release via the G i/o pathway. Myenteric neurons abundantly express FFA3, and intestinal transit in FFA3 deficient mice is accelerated likely due to the lack of neuronal and PYY-mediated inhibition, suggesting that FFA3 significantly contributes to colonic motility regulation.
Microbial-derived SCFAs may influence the development of myenteric neurons, which predominantly influence intestinal motility control. A diet enriched in the fermentable carbohydrate resistant starch increases luminal SCFA production and cholinergic, but not nitrergic, neuron populations in rat distal colon. In contrast, GF mice have less nitrergic neurons but similar cholinergic neuron populations compared with conventional controls. IBS patients with motility dysfunction have variable colonic SCFA content compared with healthy controls, independent of the predominant symptoms of constipation or diarrhea. These reports suggest that dietary therapy or pre- and probiotics may improve intestinal motility disorders through alteration of the myenteric neural population via increased luminal SCFA production.
28.5
Polyamines and the Ca ++ Sensing Receptor
PAs, such as putrescine, spermine, and spermidine, are necessary for intestinal cell proliferation, differentiation, and restitution after injury. Spermine and spermidine alter the activity of astrocytes and mast cells that express the selective vesicular PA transporter termed VPAT (SLC18B1). Although PAs are biosynthesized through ornithine decarboxylase, the predominant source is diet. Dietary PAs are rapidly absorbed by the upper intestine, whereas PAs and their precursor ornithine are present in sub-mM concentrations in the colonic lumen due to microbial production, especially by prokaryotes ; the active uptake of PAs has been identified in colonic epithelial cell lines and in cancer cells. The proliferative effect of PAs is mediated by Ca ++ sensing receptor (CaSR) activation, which is probably expressed in L cells present in the small intestine.
CaSR is a polymodal chemosensor, possessing a large extracellular domain. Extracellular Ca ++ , Mg ++ , and spermine are agonists of CaSR ; also l -amino acids and poly- and oligo-peptides allosterically activate CaSR in the mM range. Expression of CaSR was detected on apical and basolateral membrane of rat colonic epithelia, as well as on normal and malignant human colon. Extracellular PAs and Ca ++ synergistically and inversely modulate cAMP- dependent colonic fluid secretion through CaSR activation, suggesting that CaSR mediates luminal ligand- induced ion transport regulation. Since a CaSR antagonist inhibited oligopeptide-stimulated GLP-1 secretion from murine primary colonic cultures, CaSR may function as a sensor also in the colonic L cells.
28.6
Proton Sensing GPCRs
Extracellular pH is sensed by a GPCR subfamily that includes GPR4, ovarian cancer G protein-coupled receptor 1 (OGR1, also known as GPR68), T-cell death associated gene 8 (TDAG8, also known as GPR65), and G2A. Although G2A activation by protons is controversial, the acid-sensing function of GPR4, OGR1, and TDAG8 are revealed with a set point of regulation precisely in the physiological range (pH 6.8–7.8). The topology and physiological functions of these receptors in the colonic epithelial cells is unknown. Upregulation of these pH sensors in the inflamed colonic mucosa is implicated in pathogenesis of inflammatory diseases, which are associated with low luminal and tissue pH.
OGR1 is inactive at pH 7.8 and fully activated at pH 6.8 with half-maximal activation at pH 7.48 and pH 7.44 in OGR1-transfected CCL39 and HEK293 cells, respectively. Some essential metals, such as Fe ++ , Zn ++ , Co ++ , Ni ++ , and Mn ++ , but not Cu ++ , were identified as OGR1 agonists that activate G q -coupling inositol phosphate signals in neutral pH at 10–100 μM in an HEK293 expression system. Micromolar (1–10 μM) concentrations of Zn ++ and Cu ++ ions inhibit OGR1 activation stimulated at pH 6.9. OGR1 increases G q -coupled inositol phosphate accumulation stimulated by low pH, whereas the close relatives GPR4 and TDAG8 increase cAMP formation elicited by similar range of extra cellular pH, consistent with G s -coupled receptor activity. These receptors likely serve distinct functions in the maintenance of pH homeostasis.
Since inflammation is frequently associated with acidic tissue microenvironments, extracellular pH sensor expression has been investigated in inflammatory diseases. The physiological range of luminal pH is 6.5–7.5 in the ileum compared with 5.5–7.5 in the cecum, and 6.1–7.5 in the colon in healthy volunteers. The apical surface of the colonic epithelia is maintained between 7.1–7.5 in humans due to mucus and bicarbonate secretion. In rats with a large cecum as a fermentation site, the surface pH is 7.5 in the cecum, decreased to 6.2 in the early proximal colon, and maintained pH ∼ 7.5 throughout the mid- and distal colon. This pH regulation is clearly correlated with segmental differences in the expression of the anion exchanger downregulated in adenoma (DRA). Luminal and tissue pH in active IBD patients are significantly lower than that in normal subjects. In some active ulcerative colitis patients, the lowest pH value in proximal colonic lumen was reported as 2.3–3.4.
OGR1 expression is increased twofold in isolated crypts obtained from the large intestine of IBD patients compared to that from control subjects. Tumor necrosis factor (TNF)-α treatment upregulates OGR1 expression via nuclear factor (NF)-kB signal in a human macrophage cell line and in primary monocytes in vitro; spontaneous inflammation in the Il-10 deficient female mice model is protected by cross knock-out of OGR1, suggesting that OGR1 is involved in inflammation development. The same group further showed that activation of OGR1 by a slightly acidic pH in the medium bathing Caco-2 epithelial cells acutely enhanced barrier function over several hours, reduced cell motility, and induced proteins involved in cell junction assembly and cell/matrix interaction as well as genes associated with tissue inflammation. Whereas stabilization of the epithelial barrier has beneficial effects during acute infections, epithelial cell motility is important for the normal homeostasis of the intestinal mucosa. The proton pump inhibitor omeprazole upregulates OGR1 protein expression approximately two-fold in the colonic cancer cell line Caco-2 and increased Mg ++ transport in an OGR1-dependent manner, suggesting that OGR1 may function as a luminal pH sensor for maintaining mucosal barrier integrity in colonic epithelial cells.
GPR4 mRNA is expressed in normal human small intestine, but is scarcely detected in the colon. GPR4 mRNA and protein levels are upregulated in IBD patients and in the dextran sulfate sodium (DSS)-induced colitis murine model. GPR4-deficient mice have less inflammation after DSS treatment, suggesting a pro-inflammatory function of GPR4. Contrariwise, TDAG8, expressed in peritoneal macrophages, when activated by extracellular acidification inhibits cytokine production, such as TNF-α and IL-6. Each family member may thus serve distinct functions depending on the cell type and stage of the inflammation process.
28.7
Bitter Taste Receptors
Since the taste-specific G protein gustducin transduces taste receptor signals, its α-subunit (Gα gust ) is used as a taste cell marker. The localization of Gα gust in the rat GI tract was first demonstrated in tuft cells, with species and segmental variations subsequently identified. These findings suggest that taste receptors are also involved in intestinal chemical sensing, with functions varying according to species and segment of the GI tract that have distinct luminal environments.
The G protein-coupled bitter taste receptor (T2R) family includes about 30 members in humans and rodents. Each member of the T2R family consists of a short extracellular domain, with relatively divergent amino acid sequences (25%–90% identity), and functions as a monomer. Some bitter compounds activate the same T2R member, whereas some T2Rs respond to the same compound, consistent with low receptor ligand selectivity. This variability is thought to correspond to an ability to detect diverse ligands present in the external environment. Expression of mRNA from most members of the T2R family has been detected in human and rat colonic mucosa. A human T2R38 (T2R138 in mice) ligand, the bitter tastant 6- n -propyl-2-thiouracil (6-PTU) induces electrogenic anion (Cl − and HCO 3 − ) secretion in the human and rat colon through a tetrodotoxin (TTX)-insensitive pathway. Exogenous PGE 2 enhances 6-PTU-induced secretion in a PGE 2 -concentration dependent manner, suggesting that high concentrations of tissue PGE 2 induced by inflammation potentiate the T2R-activated secretory response, causing diarrhea. In mice, T2R138 is predominantly expressed in the colon more than in small intestine and stomach, whereas T2R108 expression is limited to the proximal colon and stomach. A bitter tastant-rich, low-cholesterol diet upregulates T2R138 expression in the upper intestine, whereas a high fat diet upregulates T2R138 expression in the colon, suggesting that certain T2R members act as chemosensors that alter gut functions according to the luminal environmental.
28.8
Bile Acid Receptor GPBA
The G protein-coupled bile acid receptor GPBA (also known as GpBAR1, TGR5, GPR131, BG37) activates isolated L cells of murine and human colon and EEC cell lines such as STC-1, GLUTag, and NCI-H716. The second messenger of GPBA coupling to Gα s is cyclic adenosine monophosphate (cAMP) that potentiates GLP-1 release from L cells upon Ca ++ -mediated nutrient sensing. GPBA expression is upregulated in murine models of colitis and Crohn’s disease, and GPBA deficient mice have increased dextran permeability caused by tight junction abnormalities. Oral supplementation of the GPBA agonist betulinic acid prevents inflammation in murine colitis model via release of the trophic peptide GLP-2, implicating that GPBA is important to the preservation of colonic mucosal barrier integrity through L cell activation. The GPBA selective agonist with no nuclear bile acid receptor activity, INT-777 improves pancreatic function and glucose tolerance in obese mice by GLP-1 release, suggesting that GPBA-mediated L cell activation has therapeutic relevance in obese and type 2 diabetes treatments.
GPBA is also present in colonic epithelial cells, EC cells, and enteric neurons that regulate peristalsis and transmural ion and water transport. Consistent with predominant GPBA colocalization with nNOS in myenteric neurons, bile acids inhibit the contractions of isolated proximal colonic smooth muscle through a neurogenic NO pathway in mice. Basolateral, but not apical application of INT-777 decreases anion secretion via suppression of neuronal and direct epithelial pathways in rat colon. Since 95% of bile acids, which are secreted into the duodenal lumen via the common bile duct, are absorbed in the ileum and 5% are metabolized by the intestinal microbiota, supraphysiological concentrations of luminal bile acids in the colon may signal the presence of malabsorption. GPBA activation is thought to slow intestinal transit via neuronal and hormonal pathways and thus accelerate absorption of luminal solutes and encourage microbial fermentation.
28.9
Olfactory Receptors
The olfactory receptor (OR) family comprises the largest share of GPCR genes, suggesting that ORs serve important functions as sensors of numerous ambient molecules. Although a variety of OR members are expressed in EC cells isolated from the upper intestine, colonic OR function has rarely been investigated. Odorants, including terpenoids derived from five-carbon isoprene units, are produced by many plants, insects, and gut commensal bacteria. OR ligands synthesized in the colonic lumen may be detected by mucosal chemosensors. Indeed, OR1G1 is present in isolated colonic mucosa; its ligand thymol increases the rate of anion secretion and transmucosal permeability in rat distal colon. Recently, Pluznick et al. de-orphanized murine Olfr78 (human homolog is OR51E2) as a SCFA receptor, particularly for acetate and propionate, acting on renal vascular smooth muscle. Since many microbial metabolites are VOC, additional functional ORs could be present in the colon.
28.10
Guanylyl Cyclase-C
Guanylyl cyclase (GC)-C (as known as GUCY2C) was discovered as the heat-stable enterotoxin (ST a )-binding protein that induces vast fluid secretion resulting in diarrhea through cGMP accumulation. GC-C localization in the apical membrane of the gut epithelium was demonstrated by 125 I-ST a binding in the mammalian intestine including humans, rats and pigs, with greater density in the upper half of crypts in the colon. While its downstream, cGMP- dependent protein kinase (cGK) localized to the apical membrane of intestinal epithelial cells is cGK II, cGK I is localized to smooth muscle and villus lamina propria. cGK II mRNA and protein are predominantly expressed in the proximal colon, but rarely in the distal colon of rats, consistent with segmental differences in cGMP-mediated anion secretion. Epithelial cGMP is released into the lumen of small intestinal loops, whereas cultured CaCo-2 cells release cGMP into the subepithelial space. Extracellular cGMP inhibits colonic nociceptor activation whereas epithelial GC-C activation stimulates transepithelial ion and water secretion; both pathways are activated by the 14-amino acid ST a peptide mimetic linaclotide, which is beneficial for improving chronic constipation in constipation- predominant IBS.
Consistent with GC-C and cGK II localization, the endogenous ligand of GC-C, guanylin, is produced by the columnar cells in the colon, particularly in the surface epithelial cells in humans and in rats. The muscarinic ACh receptor agonist, forskolin, and vasoactive intestinal peptide (VIP) stimulate guanylin release into the lumen. In isolated tissues, luminal, but not basolateral administration of guanylin induces secretion in the human colon and rodents. In vivo studies confirmed that anion and water secretion are induced by luminal guanylin, though less potently than ST a . Although no known luminal molecule stimulates guanylin release, guanylin is likely secreted as an autocrine mediator and participates in the physiological regulation of epithelial chloride and bicarbonate secretion.
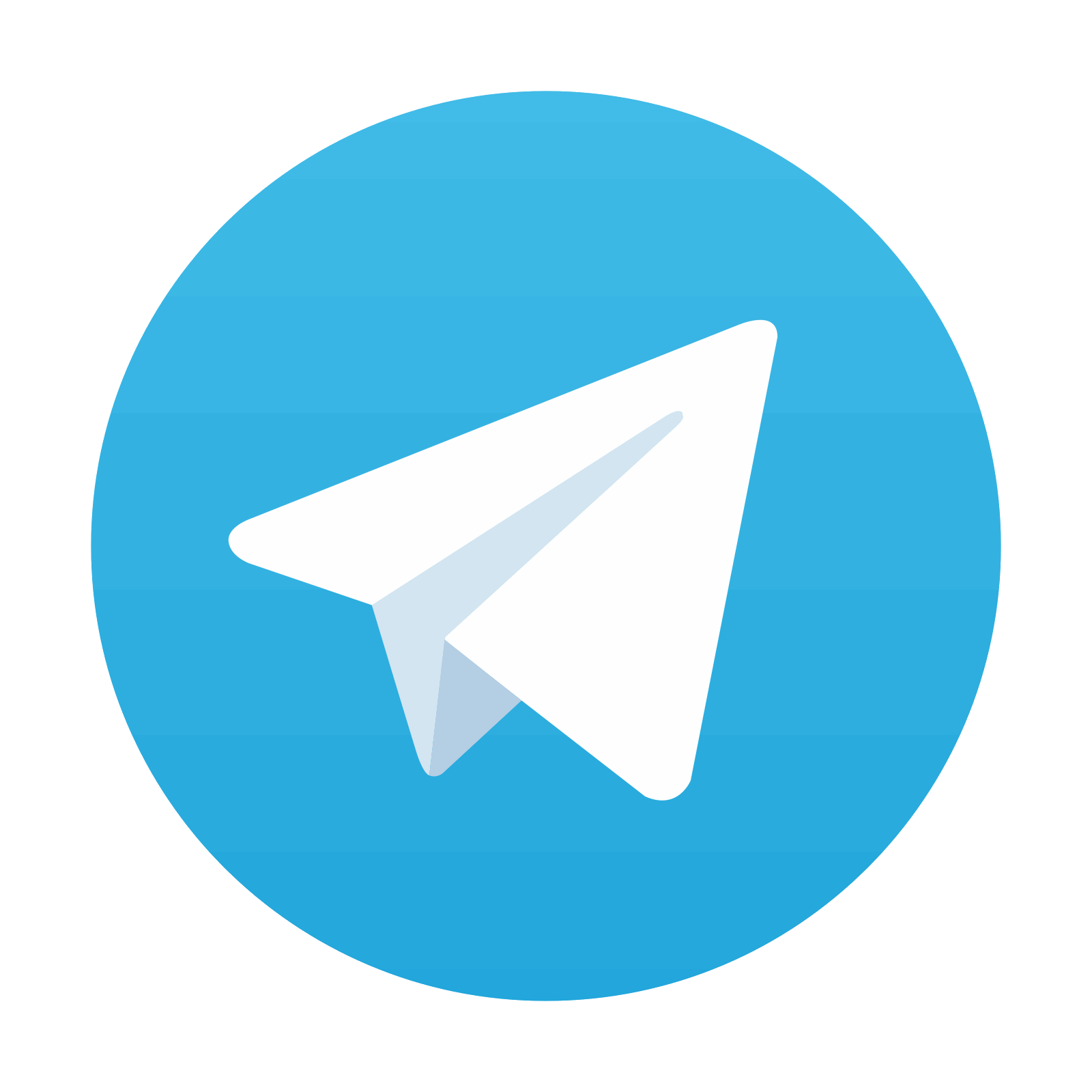
Stay updated, free articles. Join our Telegram channel

Full access? Get Clinical Tree
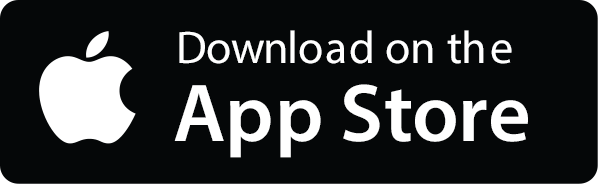
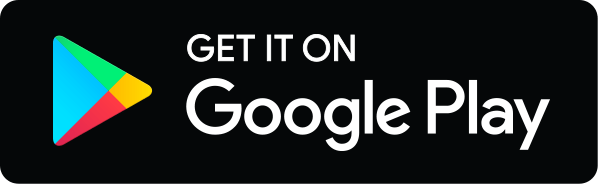