Fig. 10.1
Pedigree for Simon Jones and Heather Simpson
Heather proceeds to have DNA analysis for the same 50 common CFTR mutations, and no mutations are found. The couple are reassured that their risk of having a child with classical CF is low, and Simon undergoes successful microsurgical epididymal sperm aspiration (MESA). In vitro fertilization (IVF) with intra-cytoplasmic spermatozoa injection (ICSI) is performed, and the couple progress to a clinical and biochemical pregnancy. Simon’s parents and siblings are advised to seek CF carrier testing via their general practitioners and the couple go on to have a healthy male child born at term. The baby boy undergoes neonatal heel prick screening for conditions including CF, which reveals normal immunoreactive trypsinogen (IRT) levels. Heather and Simon are advised to inform their son that he may wish to access genetic carrier testing for CF before starting his own family in the future.
What Is CFTR?
Cystic fibrosis transmembrane conductance regulator (CFTR) is the protein product of the CFTR gene that was first isolated in 1989, after a long international research effort to find the causative gene for the human genetic disorder cystic fibrosis [1–3]. The CFTR gene is located on chromosome 7 at band 7q31.2, and Riordan et al. identified 24 exons in the CFTR gene [2]. The sections of DNA within a gene that will encode the final protein molecule (in this case CFTR) reside within “exons” and the intervening DNA segments are termed “introns.” During transcription of the DNA sequence into RNA, all the exons and introns are transcribed but then the RNA molecule is modified, removing the intron sequences via a process known as “splicing.” The molecule also has other modifications made to it (a methyl cap—a methylated guanosine molecule—is added to the 5′ end and a polyA tail to the 3′ end of the gene) before a mature messenger RNA (mRNA) molecule is produced. Pathogenic mutations are usually found within the exons of a gene or at the exon-intron boundaries. These boundaries are the sites at which splicing occurs and genetic variation at these points could result in either the failure to insert exon sequences (known as “exon skipping”) or incorrect insertion of intronic sequences into the final mRNA molecule.
The CFTR protein functions as a cyclic adenosine monophosphate (cAMP)-regulated chloride- and bicarbonate-conducting channel [4]. CFTR mutations cause abnormal flow through this channel and lead to defective fluid and electrolyte transport. This mechanism is thought to be a major contributor to the respiratory, pancreatic, and nasopharyngeal symptoms in CF [5].
Every person carries 2 copies of the CFTR gene, and common nomenclature refers to any mutations on the same chromosome (or allele) as in cis and mutations on opposite alleles as in trans. Any person with a CFTR-related phenotype will have pathogenic mutations in both copies of their CFTR gene (i.e., the mutations will be in trans) and will have inherited one mutation from each parent (that parent being a “heterozygote” or healthy carrier). Sometimes, the mutation will be the same in both alleles, and under these circumstances, the patient is described as being a “homozygote” for the mutation. In other cases, the 2 mutations are different and the patient is then described as a “compound heterozygote.”
Mutations in the CFTR gene can result in a spectrum of clinical phenotypes, which can include CF, CBAVD, bronchiectasis, idiopathic pancreatitis, and sweat chloride elevation without CF. A simple subdivision would be into classical CF on the one hand and the milder CFTR-related disorders (CFTR-RD) on the other.
CF is the most common lethal genetic disorder in Caucasians, with an incidence reported at between one in 3500 [6] and one in 2500 [7, 8], giving an estimated carrier frequency of one in 25 [9]. Patients with classical CF manifest multi-organ dysfunction, chronic lung infection and inflammation, pancreatic insufficiency and infertility. CFTR-RD include patients with biallelic mutations (i.e., mutations in both alleles) of the CFTR gene who present with milder, atypical and late-presenting forms of CF—including isolated CBAVD or CBAVD with mild or atypical CF features, such as CBAVD with bronchiectasis.
How Common Is CFTR-Related Male Infertility?
Infertility is seen in around 15% of couples worldwide, and 40% of cases have a male cause [10]. Azoospermia (the absence of spermatozoa in the ejaculate) can be classified as non-obstructive or obstructive, and CBAVD accounts for 6% of cases of obstructive azoospermia (OA) and 1–2% of all cases of male infertility [11]. In male infertility secondary to OA, cases diagnosed with CBAVD using strict criteria are commonly caused by pathogenic CFTR mutations [12, 13]. Indeed, Dequeker et al. suggest that 80% of patients with isolated CBAVD have 2 identifiable pathogenic CFTR mutations, usually in a compound heterozygous state [12].
How Does CFTR-Related Male Infertility Present?
Male patients with CFTR-related infertility may present in a variety of ways: a patient with classical CF diagnosed in childhood who is referred for fertility discussions when transitioning to adult services; a male patient diagnosed with mild or atypical CF in early adulthood who is found to be azoospermic; a patient with azoospermia who has no palpable vas deferens on examination; or a patient who is diagnosed with OA secondary to CBAVD found on imaging. The latter examples of a male patients with OA secondary to CBAVD who are subsequently shown to have 2 mutations in CBAVD are the most common presentation of CFTR-related male infertility.
How Do CFTR Mutations Cause Male Infertility?
Of men with biallelic CFTR mutations, 95–97% are infertile due to a combination of viscous semen and absence of Wolffian duct structures, commonly presenting as CBAVD [14]. A fetal anomaly in the seventh week of gestation results in the characteristic anatomic pathology of CBAVD, including absent vasa and complete or partial absence of epididymis [15]. No female equivalent of CBAVD exists, due to the different embryological origin of the female reproductive tract.
Not all cases of CBAVD are caused by CFTR mutations, but in males with either unilateral or bilateral congenital absence of vas deferens (CAVD), CFTR mutation testing is indicated [16, 17]. Diagnostic criteria for CFTR-related CBAVD have been outlined (Table 10.1) [12] and could be used as a prompt to initiate CFTR testing in males with these clinical features.
Table 10.1
Diagnostic criteria for CFTR-related CBAVD
Clinical feature | Further definition or laboratory values |
---|---|
Azoospermia | |
Low seminal fluid volume | <2.0 ml |
Typical biochemical features | pH < 7.2, absent/decreased fructose and α(alpha)1–4 glucosidase |
Absence of palpable vas deferens | Absent on trans-scrotal USS |
Anatomical abnormalities on trans-rectal ultrasound | Absence of intra-abdominal tract of vas deferens, globus major, and hypoplasia of the seminal vesicles |
FSH, LH, and testosterone levels within normal limits | See local laboratory limits |
CFTR-related male infertility might thus be suspected based on a combination of clinical features: demonstration of OA with typical biochemical and clinical findings of absent vas deferens on clinical examination or trans-scrotal ultrasound (Table 10.1). The finding of 2 CF mutations on the molecular testing of peripheral blood lymphocytes, often in compound heterozygous state, in a male patient with CBAVD confirms CFTR-related male infertility. Depending on the genotype, further clinical evaluation for features of classical or atypical CF may be required.
Can CFTR Mutations Cause Non-CBAVD Infertility?
The question of whether CFTR mutations can cause non-CBAVD infertility was raised by Van der Ven et al., who screened 127 unrelated, healthy males attending infertility clinics for 13 common CFTR mutations [18]. They found a single CFTR mutation in 17.5% of the men attending with reduced sperm quality and in 9.5% of men attending with azoospermia. No CFTR mutations were found in the 26 men with normozoospermia. The authors reported an increased frequency of mutations, including the IVS8-5T allele, in non-CBAVD azoospermic males compared to both fertile males and the general population incidence. The conclusion of this study was that heterozygous mutations in CFTR may confer a risk of non-CBAVD male infertility [18].
Pallares-Ruiz et al., however, compared the CFTR genotypes of men with severe oligoasthenoteratozoospermia (OAT) to matched background controls and found no significant differences in the frequency of CFTR mutations [19]. The authors concluded that neither the IVS8-5T allele (see later) nor any other CFTR mutations were implicated in OAT and that the findings by van der Ven et al. may have been due to undetected CBAVD cases. Further studies have also supported this theory [20]. There is therefore no firm evidence that heterozygosity for CFTR mutations, the IVS8-5T variant included, confers an increased risk of non-CBAVD male infertility.
Does the Type of CFTR Mutation Determine the Phenotype?
More than 2000 CFTR mutations have been described to date [12, 21–23], and they are often individually rare, making genotype–phenotype correlations complex, especially as clear phenotypic categories are difficult to define [24]. The frequencies of specific mutations vary depending on geographical and ethnic backgrounds [9, 25]. The most common CFTR mutation is p.Phe508del in exon 11, accounting for 75% of all UK mutations [26]. The p.Phe508del mutation has historically been described in various ways, including Phe508del and Δ(Delta)F508, but the most accurate current nomenclature is c.1521_1523delCTT [23]. This type of mutation nomenclature gives the exact changes of the base pairs and describes deletion of the nucleotides CTT between positions 1521 and 1523 of the CFTR gene. For ease of recognition, however, the protein coding nomenclature, p.Phe508del, for this mutation will be used throughout the chapter. All other mutations are described using their current coding DNA (cDNA) names.
Classification of CFTR mutations based on their effect on CFTR protein function and production is widely quoted; however, many CFTR mutations have a number of different functional consequences [8, 12, 27]. Nevertheless, some mutations are more likely to confer a phenotype of classical CF, whilst others might be associated with no clinical features, isolated CBAVD or CBAVD with mild or atypical CF features, such as CBAVD with bronchiectasis.
How Are CFTR Mutations Detected and Reported?
Guidelines were produced in 2006 based on expert consensus opinion, to recommend a standard practice for testing and reporting of mutations in CFTR [12]. Both targeted (level 1, see later) and scanning methods (level 2, see later) exist. The former techniques detect known mutations that are common in the local population or test for a previously identified familial mutation; the latter involves more extensive sequencing of the whole CFTR gene. No single method for mutation detection is recommended, but laboratories can advise on the limitations of testing via their chosen method. Laboratories will make efforts to detect all mutations occurring at a frequency of >1% in the local population; therefore, if a patient is of an ethnic origin not commonly seen within the local population, further discussion with the local laboratory will be needed. In Liverpool and most of the UK, the standard practice is to test for the 50 most common CF mutations that occur in the local population using a commercial kit; the 50 mutations include p.Phe508del, and the next most common mutations c.1624G>T (previously known as G542X), c.1652G>A (G551D), c.3846G>A (W1282X), and c.3909C>G (N1303K); each of which accounts for only 1–2% of known mutations.
Many causative mutations remain undetected in patients with atypical presentations of CF, including CBAVD. They seem to be harder to detect in non-Northern European populations. Reasons for this are unclear but perhaps due to lack of commercial kits for targeting mutations in non-Northern European populations. Undetected CFTR mutations may lie in introns or regulatory regions that are not routinely analyzed by laboratories. Large CFTR gene rearrangements occur in 1% of CBAVD patients and can also escape detection using conventional amplification assays [12].
Searching for unknown mutations by extensive sequencing may detect sequence variants of uncertain significance. The terms “mutation” and “sequence variant” are synonymous and do not denote functional consequences or disease association [12]. The term “mutation” has been used throughout this chapter for the purposes of consistency. Similarly, the term “polymorphism” refers to a mutation with an allelic frequency of greater than 1% in the general population and does not mean that the mutation has no clinical consequences. A number of criteria are used to determine whether a sequence variant is likely to be causative (i.e., a pathogenic mutation). Such considerations include the following: (1) whether the mutation results in an amino acid change that severely affects CFTR synthesis or function; (2) the introduction of a premature termination signal (leading to shortening of the CFTR protein) and (3) variants that alter invariant intron splice sites and delete one or more exons of the CFTR gene [12]. If a mutation has been reported previously in patients with CF or CFTR-RD, laboratories can be more confident in classifying it as likely to be pathogenic. However, functional studies have only been undertaken on a very small number of mutations, meaning data about their effect on CFTR protein structure or function are lacking.
Which CFTR Mutations Are Common in CBAVD Patients?
In a large systematic review, Yu et al. looked at mutations in CFTR in males with CBAVD presenting between 1992 and 2011 [14]. This study found that 75% of patients had at least one CFTR mutation and 53% had 2 mutations detected. Where only one mutation was found, the authors felt that a second mutation would be detectable if more extensive sequencing had been available [14]. Utilising the current techniques to scan the whole CFTR gene, it would be expected that only a small minority of mutations would be technically difficult to detect, for the reasons described above. As CFTR mutations in CBAVD follow the Mendelian pattern of autosomal recessive inheritance, if only one mutation is detected, it is accepted that there is a second mutation that has escaped detection [9]. This is an important concept when counselling patients and families and is discussed further later in the chapter.
Almost all the described CF mutations have been seen in CBAVD, being p.Phe508del, seen in 17% of reported series and the milder IVS8-5T allele seen in 25% [14]. In the series published by Phillipson et al., 24% of CF chromosomes carried the c.350G>A (previously known as R117H or Arg117His), similar to the 22% initially reported in CBAVD by Gervais [28, 29]. Compared with an incidence of 0.3% of the c.350G>A variant in classic CF, these data suggest that the c.350G>A allele is seen at an increased frequency in men with CBAVD.
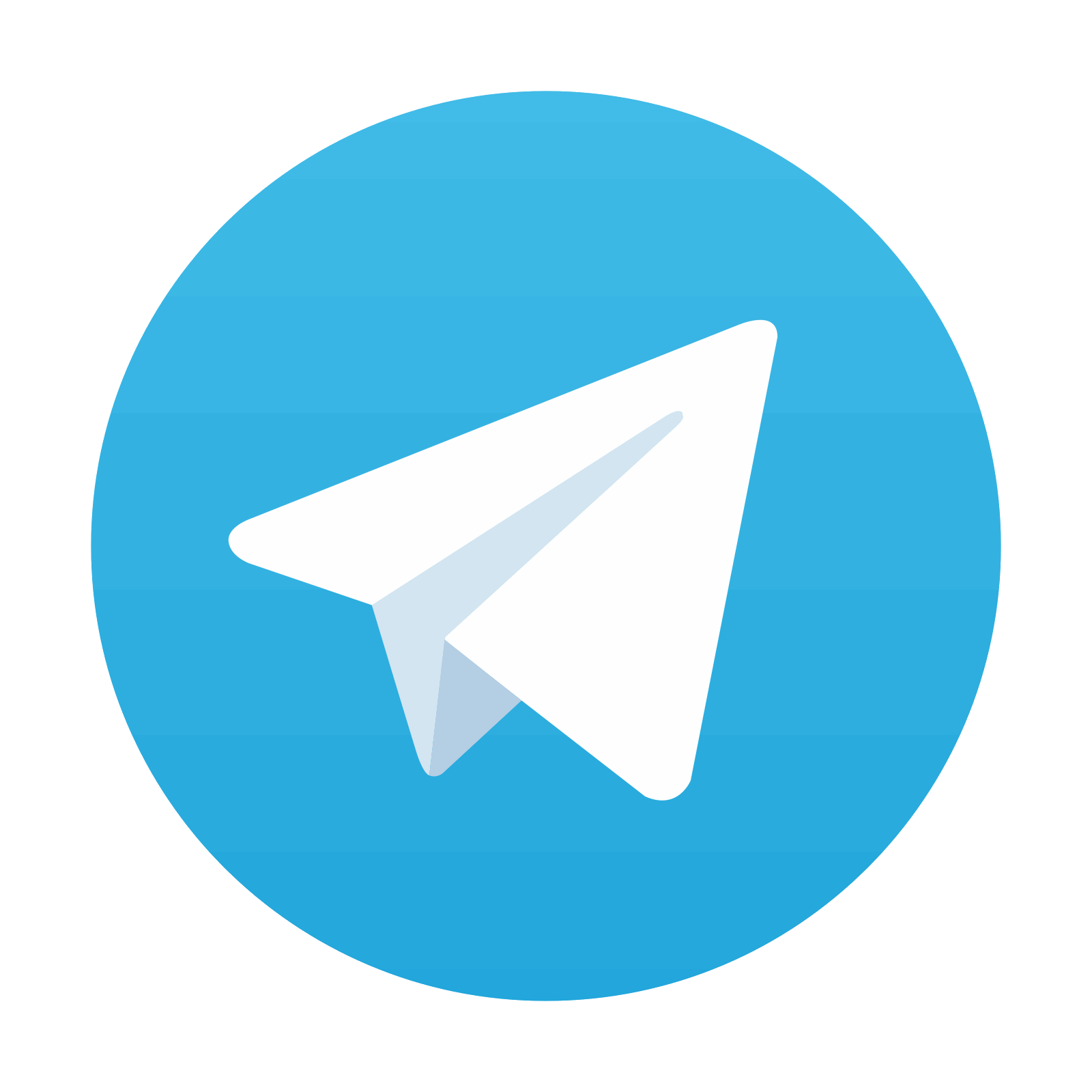
Stay updated, free articles. Join our Telegram channel

Full access? Get Clinical Tree
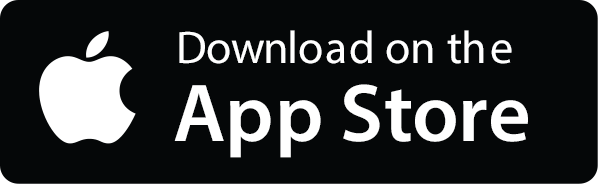
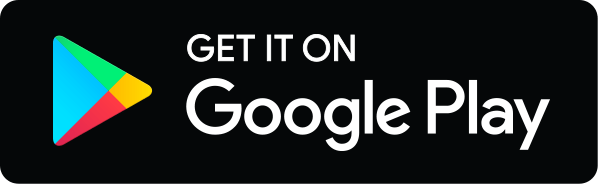