Cardiopulmonary bypass (CPB) has developed into an invaluable tool for operations on the thoracic viscera. By interfacing with the cardiovascular system, total CPB completely replaces the function of the heart and lungs for a short time. Modifications of CPB have been designed to partially replace or control more specific aspects of the cardiopulmonary systems. To fully utilize the tremendous flexibility available with “the pump,” one must understand its components, operation, and potential for complications.
The development of CPB is a testament to personal perseverance and collaboration and demonstrates how cardiac surgery has truly evolved as a science. Initial progress in the field of extracorporeal circulation required mastery of the principles of total-body oxygen delivery and consumption. Later, further refinements in the understanding of metabolic demands at the cellular level served to enhance the safety and flexibility of circulatory support. Undoubtedly the most important milestone in the development of CPB is John Gibbon’s use of the first clinical application of CPB. In 1953, after devoting most of his career to this work, he successfully repaired an atrial septal defect in a young woman with a pump-oxygenator. Additional milestones include the work of Bigelow, who, in the early 1950s, reported on systemic hypothermia with topical cooling as a means to reduce oxygen demand during the periods of reduced oxygen delivery inevitably encountered during operations on the heart. Shortly thereafter, Lillehei began performing congenital repairs using “cross circulation,” employing a parent’s cardiopulmonary system to support a child’s during cardiac surgery. Finally, in the mid-1950s, Kirklin and colleagues at the Mayo Clinic ushered in the era of routine congenital repairs using the Mayo–Gibbon pump-oxygenator. Modern refinements in equipment and techniques have subsequently evolved as a consequence of the proliferation of coronary revascularization surgery and increased industrial interest.
Current-generation CPB circuits maintain much of the simplicity of older perfusion equipment but have much more flexibility and ability to more precisely control various perfusion parameters. Core components of any circuit include cannulas, tubing, a pump, and an oxygenator (Fig. 24-1). In reality, however, even basic circuits used in routine clinical practice include many other features such as additional pump heads for suction, venting of the heart, and delivery of cardioplegia. Venous reservoirs are also needed to maintain adequate circuit volume and remove air. Filters are required at various levels to prevent embolic complications. Finally, a heat exchanger facilitates systemic and myocardial cooling and rewarming.
Cannulation for CBP is typically performed centrally with an inflow cannula in the ascending aorta and outflow cannula(s) in the right atrium. Basic requirements for an arterial cannula include a size large enough to permit adequate flows without creating excessive back pressure at the pump head. At the same time, the size and design of the cannula must allow for easy placement with minimal vessel trauma. Beyond these basic requirements, a multitude of other aspects of cannula design may arguably affect performance. Such features include tip angle, size and number of end or side holes, wire reinforcement, and filters. Most arterial catheters are rated by a performance index, which relates external diameter, flow, and pressure differential. High-velocity jets may damage the aortic wall, dislodge atheroemboli, produce dissections, disturb flow to nearby vessels, and cause cavitation and hemolysis. Aortic catheters with only side ports are designed to minimize jet effects and better distribute arch vessel perfusion and pressure; they may be associated with fewer strokes.1
Various locations on the ascending aorta and aortic arch are most often used as sites for insertion of the arterial cannula because of the simplicity and maintenance of antegrade blood flow. In patients with no significant ascending aortic atherosclerosis, this location is also associated with the least risk of inflow-related complications in routine cardiac cases. However, multiple other locations can be good alternatives, including the common femoral, axillary, and innominate arteries; descending thoracic aorta; and rarely the left ventricular (LV) apex. The choice is influenced by the planned operation and the distribution of atherosclerotic disease.
To cannulate the ascending aorta, most surgeons place two purse-string sutures with or without pledgets partially through the aortic wall to encompass a diameter of approximately 1 to 1.5 cm. Next, with a systolic blood pressure preferably less than 100 mm Hg, a 4- to 5-mm full-thickness stab wound is made, occasionally dilated, and then controlled under finger or forceps pressure. The cannula is then inserted under the finger or forceps approximately 1 to 2 cm into the lumen of the aorta. Backbleeding is detected to ensure that the tip is completely within the lumen and positioned to direct flow to the midtransverse aorta. A long catheter with the tip placed beyond the left subclavian artery may also be used. Proper cannula placement is critical and is confirmed by noting pulsatile pressure in the aortic line monitor and equivalent pressure in the radial artery.
Complications of ascending aortic cannulation include bleeding from tears in the aortic wall, malposition of the cannula tip, atheromatous or air emboli, and aortic dissection. It is essential to monitor aortic line and radial artery pressures and to carefully observe the aorta for possible cannula-related complications during the onset of CPB and placement of aortic clamps. Asymmetrical cooling of the face or neck may suggest a problem with cerebral perfusion. Late bleeding and infected or uninfected false aneurysms are delayed complications of aortic cannulation.
The common femoral artery is probably the second most common location for CPB inflow. Reasons for cannulating the femoral artery include inadequacy or inavailability of the ascending aorta in cases such as aortic dissection, reoperation, descending aortic surgery, and minimally invasive cardiac procedures. Severe atherosclerosis of the ascending aorta is generally not a good indication for femoral cannulation because of the likely coexistence of severe disease in distal segments of the aorta and iliac arteries. Alternative strategies for CPB in this scenario are discussed later in this chapter.
Exposure of the femoral artery is through a small oblique incision just below the inguinal ligament. Only the anterior surface of the vessel needs to be exposed. After placement of a 5-0 monofilament purse-string suture, the common femoral artery is punctured and a guidewire is passed to the aorta. Transesophageal echocardiography (TEE) guidance can be used to confirm the location of the wire. A long, thin-walled cannula is then passed over the wire into the distal aorta. Most adult men can be perfused adequately with a 21F cannula; in women, a 19F cannula usually suffices.
Disadvantages of femoral cannulation include local complications such as vessel injury or groin wound complications and perfusion-related problems. These include abdominal aortic dissection or perforation, embolic phenomena from retrograde flow through a diseased abdominal aorta, and malperfusion of aortic branches in acute aortic dissection. In addition, ischemic complications of the distal leg may occur during prolonged retrograde perfusions; these may result in the release of myoglobin and subsequent renal injury. Finally, some speculate that retrograde flow to the cerebral and coronary arteries may result in lower perfusion pressures or oxygen saturation of blood at the end of the perfusion stream.
The axillary subclavian artery is increasingly used for cannulation.2,3 Advantages include freedom from atherosclerosis, antegrade flow into the arch vessels, and protection of the arm and hand by collateral flow. Due to these advantages and the dangers of retrograde perfusion in patients with aortic dissection, some surgeons prefer this cannulation site for this and other conditions involving the aortic arch. The right axillary artery is usually used and is approached through a subclavicular incision, taking care not to damage the adjacent brachial plexus. In order to prevent injury to the fragile axillary or subclavian artery, some prefer to anastamose a small-caliber graft end to side to the axillary artery followed by insertion of the cannula into the graft.
In most applications, venous blood enters the circuit through negative pressure generated by siphonage. Thus, venous return flow rates are determined largely by the height differential between the right atrium and venous reservoir. Venous return can be impeded by resistance in cannulas, tubing, and connectors and may cease completely with entrainment of significant amounts of air into the venous line, creating an “air lock.” This situation can be managed by correcting the source of air entrainment (e.g., tightening a loose purse-string suture) and then elevating the venous tubing such that the air rises in the direction of the venous reservoir. Continued arterial inflow from the pump creates positive central venous pressure, which will eventually drive the air into the reservoir, where it evacuates. Venous cannulas are usually made of flexible plastic, which may be stiffened against kinking by wire reinforcement. The size required is determined by the patient’s size, the anticipated flow rate, and cannula flow characteristics, such as resistance. Catheters are typically inserted through purse-string–guarded incisions in the right atrial appendage, lateral atrial wall, or directly in the superior vena cava (SVC) and inferior vena cava (IVC).
Two basic approaches for central venous cannulation are used. For coronary artery or aortic valve operations, single venous cannulation of the right atrium alone suffices. In adults, this is usually performed with a 40F to 46F “two-stage” device that has a set of drainage holes in the distal portion and a second set located more proximally (Fig. 24-2). The distal end is positioned in the IVC while the proximal side holes drain the right atrium. This cannula may provide for better drainage than a single-stage cannula, especially when positioning of the heart may kink the venae cavae. Bicaval cannulation is used primarily for isolating the right atrium when surgery dictates opening this chamber. Caval tourniquets are also necessary in this situation to prevent bleeding and air entry into the system. Because of coronary sinus return, caval tourniquets should not be tightened without decompressing the right atrium. Bicaval cannulation is often preferred to facilitate venous return during exposure of the left atrium and mitral valve, mainly because of the potential for obstruction of the SVC or IVC during exposure. Using both techniques, the operative team should carefully monitor central venous pressure to prevent obstruction of the SVC.
Placement of a single venous cannula is most easily accomplished through the right atrial appendage. After application of a vascular clamp, the right atrial appendage is excised, or it may be opened longitudinally when the appendage is small. A purse-string suture is then placed just below the free edges, which are controlled with forceps. After release of the clamp, the cannula is inserted toward the IVC. The height of blood within the unclamped cannula is a good measure of central venous pressure. During bicaval cannulation, the IVC cannula is generally placed on the lateral wall of the right atrium near the junction with the IVC. During placement of the purse-string suture, the right atrium is gently retracted with the assistant’s hand or forceps while monitoring hemodynamics. A stab incision is made in the middle of the purse-string, the defect is dilated with a curved clamp, and the cannula, either straight or right-angled, is then directed into the IVC. The cannula should not be inserted with the proximal side holes located too distally within the IVC, as this may impede hepatic venous drainage. SVC cannulation is typically accomplished with either a straight cannula placed through the lateral wall of the atrium, as in IVC cannulation, or with a right-angled cannula placed directly into the SVC. In this procedure, the anterior surface of the SVC is exposed by dividing the pericardial reflection overlying its superior border. An oval-monofilament purse-string suture oriented longitudinally is placed, and an incision is made along the length of the purse-string suture while controlling the free edges with forceps. The tip of the cannula is then directed superiorly, taking care to avoid tip placement in the azygos vein.
Access to the central venous system for blood return can also be obtained through the common femoral or internal jugular veins. Remote cannulation for CPB is particularly beneficial for reoperative cardiac procedures, minimally invasive “on-pump” cardiac procedures such as mitral valve repair via right thoracotomy/thoracoscopy, and aortic surgery through a left thoracotomy. Depending on the size of the cannula, either open or percutaneous methods can be used. Most surgeons would recommend a maximum size of 21F to 22F for percutaneous venous access without the need for direct vessel repair after decannulation. Typically, a long, wire-reinforced cannula with an ultrathin wall is advanced over a guidewire through the femoral vein to the right atrium under transesophageal TEE guidance. For bicaval drainage, some femoral venous cannulas are designed to be advanced into the SVC; multiple levels of side holes subsequently drain both venae cavae individually. An alternative strategy for bicaval drainage employs two individual venous cannulas, one in the femoral vein to drain the IVC and one in the right internal jugular vein to drain the SVC.
As an adjunct to remote cannulation for venous drainage, most surgeons employ some means of active assist over the standard gravity or siphonage power for blood return. “Assisted venous return” can be achieved by applying a regulated vacuum to a closed hard-shell venous reservoir or by kinetically assisting return via a centrifugal pump placed in the venous return line. Assisted venous return is also useful in cases using standard central cannulation sites but where the use of smaller cannulas may be beneficial, such as a MAZE procedure or operation through a ministernotormy. The most significant disadvantage of assisted venous drainage is an increased risk of air entrainment and subsequent microembolization to the arterial circulation.4,5
The various components of the heart–lung machine are connected by polyvinyl tubing and fluted polycarbonate connectors. Medical-grade polyvinyl chloride tubing is universally used because it is flexible, compatible with blood, inert, smooth, transparent, resistant to kinking and collapse, and can be heat-sterilized. In adults, 1/2-in. tubing is generally used for inflow and 5/8-in. tubing for outflow. Wider tubing improves flow dynamics but also increases priming volume. Tubing lengths between patient and pump should also be minimized so as to reduce priming volume.
One controversy that continues over tubing is the use of herparin bonding to the interior surfaces, a technique that has many theoretical advantages associated with a reduced blood–plastic interface. The use of heparin-coated circuits during CPB has some proven advantages,6–8 such as reduced activation of complement pathways. However, there is only weak evidence that heparin-coated perfusion circuits reduce the need for systemic heparin or reduce bleeding or thrombotic problems associated with CPB, and the overall inflammatory response to CPB is not reduced.
Two types of pumps can be used, and each has its own set of advantages and disadvantages. Centrifugal pumps consist of a vaned impeller or of nested, smooth plastic cones, which, when rotated rapidly, propel blood by centrifugal force. An arterial flowmeter is required to determine forward blood flow, which varies with the speed of rotation and the afterload of the arterial line. Centrifugal pumps produce pulseless flow and generate less negative pressure; therefore, they may produce less cavitation and fewer gaseous microemboli. They can pump small amounts of air but become “deprimed” if more than 30 to 50 mL of air enters the blood chamber. Centrifugal pumps are probably superior to temporary extracorporeal assist devices and left-heart bypass, for assisting venous return. They are often used as the arterial inflow pump on the CPB circuit.
Roller pumps consist of two rollers situated 180 degrees apart inside a curved raceway. Forward flow is generated by roller compression of tubing, which can be sized from 1/4 to 5/8 in. ID depending on flow requirements. Flow rate depends on the diameter of the tubing, rate of rotation, length of the compression raceway, and completeness of compression, which is adjusted to be barely nonocclusive against a standing column of fluid that produces 45 to 75 mm Hg of back pressure. At this degree of compression, hemolysis and tubing wear are minimal. Flow rate is determined from calibration curves for each pump for different tubing sizes and rates of rotation. Roller pumps are inexpensive, reliable, and safe; they are insensitive to afterload, have small priming volumes, but can produce high negative pressures and may shed microparticles from compressed tubing. Roller pumps are used almost exclusively for vents, sucker systems, and the delivery of cardioplegia.
One advantage roller pumps have is the ability to produce pulsatile blood flow. Normally, standard roller pumps produce a sine-wave pulse of around 5 mm Hg, but they can be set to generate pulse pressures above 20 mm Hg as detected on peripheral arterial pressure monitors. Theoretically, pulsatile perfusion delivers more energy to cells and may result in improvements in peripheral resistance, renin release, and cellular hypoxia. However, studies on the clinical benefits of pulsatile perfusion are inconclusive. Complications that may occur during operation of either type of pump include loss of electricity; loss of the ability to control pump speed, producing “runaway pump” or “pump creep” when turned off; loss of the flowmeter or revolutions per minute (RPM) indicator; rupture of tubing in the roller pump raceway; and reversal of flow by improper tubing in the raceway. A means to provide pumping manually in case of electrical failure should always be available.
The venous reservoir serves as a receiving chamber for venous return; facilitates gravity drainage; is a venous bubble trap; provides a convenient place to add drugs, fluids, or blood; and adds storage capacity for the perfusion system. During full CPB, as much as 1 to 3 L of blood may be stored in the reservoir, depending somewhat on the patient’s preoperative volume status. Reservoirs may be rigid (hard) plastic canisters (“open” types) or soft, collapsible plastic bags (“closed” types). The rigid canisters facilitate volume measurements and the management of venous air, often have larger capacity, are easier to prime, permit suction for vacuum-assisted venous drainage, and may be less expensive. Soft bag reservoirs minimize the blood–gas interface and reduce the risk of pumping massive air emboli. A closed system is preferred to avoid the large amount of air–blood contact in open systems.
Membrane oxygenators are most commonly used and imitate the natural lung by interspersing a thin membrane of either microporous polypropylene (0.3- to 0.8-μm pores) or silicone rubber between the gas and the blood phases. Compared with bubble oxygenators, membrane oxygenators are safer, produce less particulate and gaseous microemboli, are less reactive to blood elements, and allow superior control of blood gases. The plasma-filled pores prevent gas from entering blood but facilitate transfer of both oxygen and CO2. The most popular design uses sheaves of hollow fibers (120–200 μm) connected to inlet and outlet manifolds within a hard-shell jacket. Arterial PCO2 is controlled by gas flow, and PO2 is controlled by the fraction of inspired oxygen (FIO2) produced by an air-oxygen blender, which also adds in variable amounts of volatile anesthetics. Modern membrane oxygenators add up to 470 mL of O2 and remove up to 350 mL of CO2 at 1 to 7 L of flow with priming volumes of 220 to 560 mL. Most units combine a venous reservoir, heat exchanger, and hollow-fiber membrane oxygenator into one compact unit.
Bubble oxygenators are obsolete in most Western countries but are used elsewhere for short-term CPB because of their low cost and good efficiency. Because each bubble presents a new foreign surface to which blood elements react, bubble oxygenators cause progressive injury to blood elements and entrain more gaseous microemboli. In bubble oxygenators, venous blood drains directly into a chamber into which oxygen is infused through a diffusion plate that produces thousands of small oxygen bubbles within the blood. Gas exchange occurs across a thin film at the blood–gas interface around each bubble. Bubbles and blood are separated by settling, filtration, and defoaming surfactants in a reservoir. Commercial bubble oxygenators incorporate a reservoir and heat exchanger within the same unit and are placed upstream to the arterial pump.
Heat exchangers control body temperature by heating or cooling the blood passing through the perfusion circuit. Frequently, hypothermia is used during cardiac surgery to reduce oxygen demand or facilitate operative exposure by temporary circulatory arrest. Gases are more soluble in cold than in warm blood; therefore, rapid rewarming of cold blood in the circuit or body may cause the formation of bubble emboli. Most membrane oxygenator units incorporate a heat exchanger upstream of the oxygenator so as to minimize bubble emboli. Blood is not heated above 40°C to prevent denaturation of plasma proteins, and temperature differences within the body and perfusion circuit are limited to 5°C to 10°C to prevent bubble emboli. The heat exchanger may be supplied by hot and cold tap water, but separate heater/cooler units with convenient temperature-regulating controls are preferred.
Filters are universally used within CPB circuits to prevent both macro- and microemboli, which, in both gaseous and particulate form, likely produce much of the morbidity associated with cardiac operations using CPB. As compared with no filter, studies indicate that all commercially available filters effectively remove gaseous and particulate emboli. Most investigations find that the Dacron wool depth filter is most effective, particularly in removing micro- and macroscopic air. Pressure differences across filters vary between 24 and 36 mm Hg at a flow of 5 L/min. Filters cause slight hemolysis and tend to trap some platelets.
Because of significant debris present within cardiotomy suction blood, the need for microfilters in the cardiotomy suction reservoir is universally accepted, and most commercial units contain an intergrated micropore filter. Most circuits also contain an arterial line filter of some type, although efficacy in this location remains unsettled. These filters are usually also of the Dacron wool type and must be used with a bypass line during priming to purge trapped air. Leukocyte-depleting arterial filters have not been proven effective at limiting the inflammatory response or other complications.
Blood aspirated from the surgical wound may be directed to the cardiotomy reservoir for defoaming, filtration, and storage before it is added directly to the perfusate. A sponge impregnated with a surfactant removes bubbles by reducing surface tension at the blood interface, and macro-, micro-, or combined filters remove particulate emboli. Negative pressure is generated by either a roller pump or vacuum applied to the rigid outer shell of the reservoir. The degree of negative pressure and blood level must be monitored to avoid excessive suction or introducing air into the perfusate.
The cardiotomy suction and reservoir are major sources of hemolysis, particulate and gaseous microemboli, fat globules, cellular aggregates, platelet injury and loss, cytokine-generated thrombin formation, and fibrinolysis. Air aspirated with wound blood contributes to blood activation and destruction and is difficult to remove because of the high proportion of nitrogen, which is poorly soluble in blood. High suction volumes and the admixture of air are particularly destructive of platelets and red cells. Commercial reservoirs are designed to minimize air entrainment and excessive injury to blood elements. The removal of air and microemboli is also facilitated by allowing aspirated blood to settle within the reservoir before it is added to the perfusate.
An alternative method for recovering field-aspirated blood is to dilute the blood with saline and then remove the saline to return only packed red cells to the perfusate. Centrifugal cell washers automate this process and remove air, thrombin, and nearly all biologic and nonbiologic microemboli from the aspirate at the cost of discarding plasma. A third alternative is to discard all field-aspirated blood. Increasingly, field-aspirated blood is recognized as a major contributor to the thrombotic, bleeding, and inflammatory complications of CPB.
During CPB, blood escaping atrial or venous cannulas and from the coronary sinus and Thebesian veins may pass through the unopened right heart into the pulmonary circulation. This blood plus bronchial venous blood, blood regurgitating through the aortic valve, and blood from undiagnosed abnormal sources (patent foramen ovale, patent ductus, etc.) may distend the left ventricle. Venting of the heart during CPB aids in myocardial protection by preventing ventricular distention and keeping warm blood out of the heart during hypothermic cardioplegic arrest. Ventricular overdistention can be a particularly serious problem in the presence of severe aortic valvular insufficiency.
There are several methods for venting the left heart during cardiac arrest. The most common techniques include placement of a multihole catheter from the right superior pulmonary vein across the mitral valve; use of an aortic root vent, often in combination with an antegrade cardioplegia catheter; placement of a suction catheter in the main pulmonary artery, which decompresses the left atrium across the pulmonary capillary bed; and, less commonly, placement of a suction catheter directly into LV apex. Vent catheters are drained to the cardiotomy reservoir, usually by a dedicated roller pump. The most common complication of left heart venting is residual air when the heart is filled and begins to contract. De-airing maneuvers (Table 24-1)—such as manual displacement, Valsalva breaths, and TEE detection of residual pockets—are important methods for ensuring its removal.
|
Cardioplegic solutions are delivered through a separate perfusion system, which includes a reservoir, heat exchanger, roller pump, bubble trap, and perhaps microfilter. The system may be completely independent of the main perfusion circuit, or it may branch from the arterial line. The system may also be configured to vent the aortic root between infusions.
Hemoconcentrators, like oxygenators, contain one of the several available semipermeable membranes (typically hollow fibers) that transfer water, electrolytes (e.g., potassium), and molecules up to 20 kDa out of the blood compartment. Hemoconcentrators may be connected to either venous or arterial lines or a reservoir in the main perfusion circuit, but they require high pressure in the blood compartment to effect fluid removal. Thus, a roller pump is needed unless the unit is connected to the arterial line. Suction may or may not be applied to the air side of the membrane to facilitate filtration. At flows from 500 mL/min, up to 180 mL/min of fluid can be removed. Hemoconcentrators conserve platelets and most plasma proteins as compared with centrifugal cell washers and may allow greater control of potassium concentrations than is possible with the use of diuretics. Aside from cost, disadvantages are few and adverse effects rare.
The performance of CPB requires constant interaction between the surgeon, the perfusionist, and the anesthesiologist, especially during periods of transition to and from artificial perfusion. Although frequent dialogue is important, often a member of the team, usually the surgeon, may be focused on other aspects of the operation and unavailable to discuss nonemergent aspects of perfusion in a particular case. For this reason, written protocols and procedures developed in a multidisciplinary fashion are important for ensuring the safe conduct of CPB.
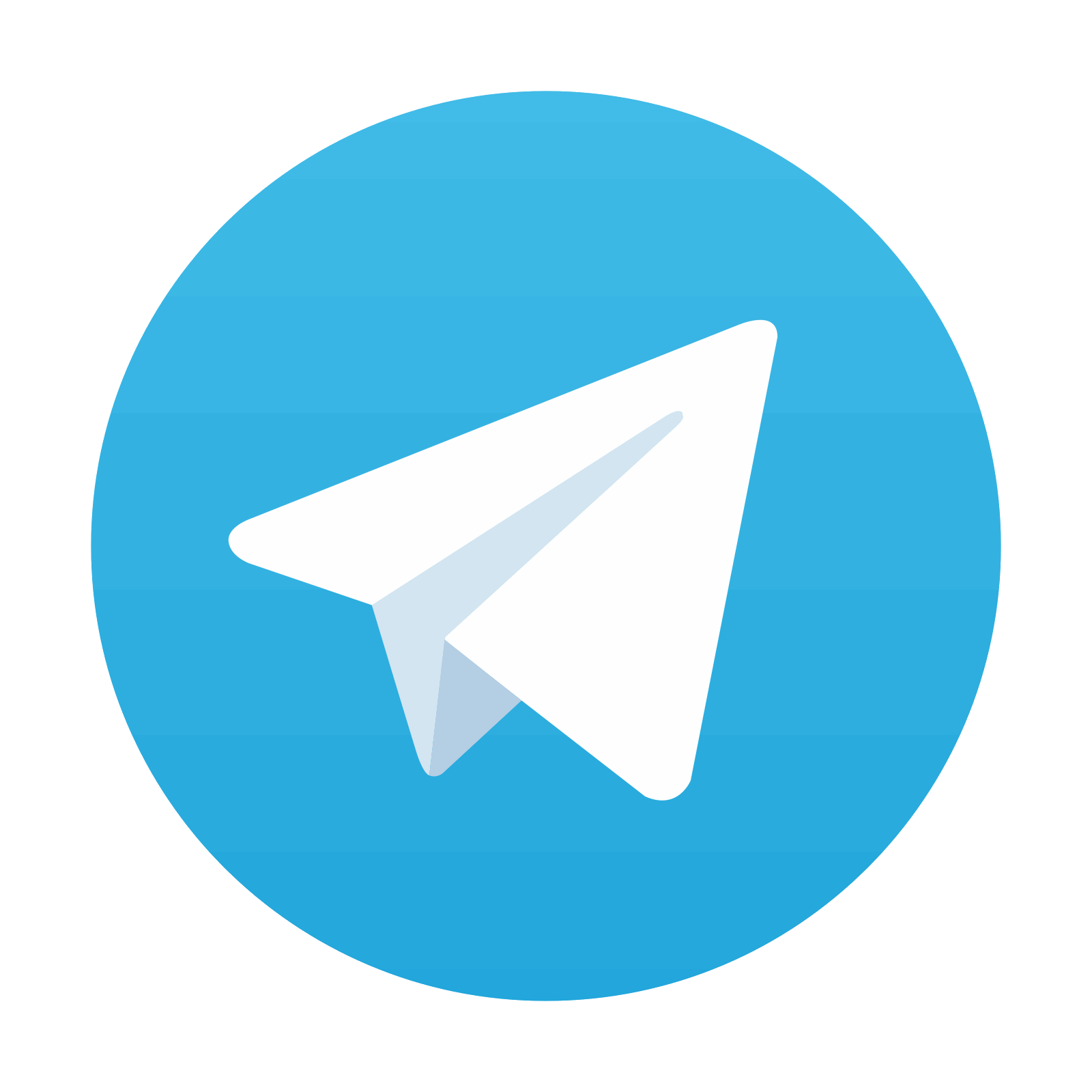
Stay updated, free articles. Join our Telegram channel

Full access? Get Clinical Tree
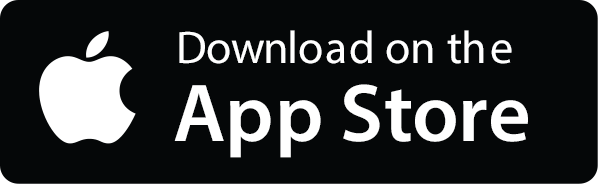
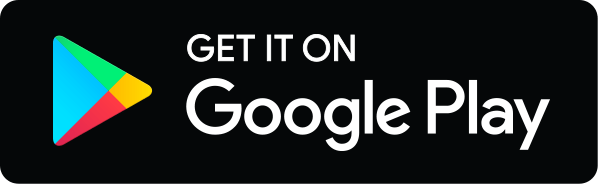