The bladder can lose the ability to store and empty effectively as a result of numerous conditions. When conservative methods to maximize patient safety and quality of life fail, surgical reconstruction of the bladder is usually considered. Augmentation cystoplasty can be performed with the use of the small bowel, large bowel, or less often, stomach. An alternative approach, tissue engineering, identifies the body’s own potential for regeneration and supports this propensity with appropriate raw materials and growth factors so that the body’s original structure and function may be restored. Tissue engineering can involve the use of a scaffold or matrix alone or of cell-seeded matrices. Harvesting cells and culturing them has become an important tool in tissue engineering. Multiple possibilities for sources of cells have been investigated, including stem cells and differentiated cells from organs other than the bladder; however, to date, autologous bladder cells remain the gold standard for culture and seeding.
The properly functioning urinary bladder is composed of a compliant muscular wall and highly specialized urothelium. When operating effectively, it provides a low-pressure, high-capacity reservoir while simultaneously protecting the upper urinary tract from pressure, reflux of urine, and infection. Specialized characteristics of the bladder’s smooth muscle fibers, nerves, and blood vessels permit repeated coordinated bladder contractions without compromising storage capability or upper tract protection. When this organ becomes damaged, any attempt at replacing it, in whole or part, must take into consideration all these properties, including reproducing or recreating them.
The bladder can lose the ability to store and empty effectively as a result of numerous conditions, such as cancer, trauma, infection, inflammation, or iatrogenic injury. Additionally, congenital or neurologic conditions, such as spina bifida or spinal cord injury, can also cause progressive loss of bladder function resulting in debilitating urinary incontinence or renal impairment. Conservative methods can be used to maximize patient safety and quality of life when there is mild-to-moderate bladder dysfunction, including behavioral training, clean intermittent catheterization, suprapubic catheterization, and pharmaceuticals. However, when conservative measures fail to protect the upper tracts or lead to worsening quality of life, surgical reconstruction of the bladder is usually considered. The present gold standard, augmentation cystoplasty, can be performed with the use of the small bowel, large bowel, or less often, stomach. Autoaugmentation, described as a partial removal of the detrusor while sparing the urothelium, has also been advocated as a solution in subsets of patients. However, the potential complications from the use of a portion of the gastrointestinal tract to perform augmentation cystoplasty are well characterized. They include urinary tract infections, intestinal obstruction, mucus production, electrolyte abnormalities, perforation, and carcinogenesis. In many patients with neurogenic bladder, particularly those with spina bifida, surgical reconstruction of the bladder with the bowel can impact fecal transit time, and this risk needs to be reviewed thoroughly with the patient and family. All these issues beg for an alternate solution.
Tissue engineering was proposed as an alternative for generating bladder tissue for reconstruction in the early 1990s, and tremendous progress has been made in this field since that time. Tissue engineering, as defined by Langer and Vacanti in 1993, is “an interdisciplinary field that applies the principles of engineering and life sciences toward the development of biologic substitutes that restore, maintain or improve tissue function or a whole organ.” Tissue engineering identifies the body’s own potential for regeneration and supports this propensity with appropriate raw materials and growth factors so that the body’s original structure and function may be restored. Tissue engineering can involve the use of a scaffold or matrix alone, wherein the body’s natural ability to regenerate is primarily used, or the use of cell-seeded matrices, in which regeneration is even further promoted. Both these technologies have been studied in the quest to develop an engineered bladder.
The use of unseeded matrices involves in vivo implantation of bioactive or resorbable matrices. The goal of this technology is to allow the body’s natural capability to use the matrix as a scaffold for regeneration. Cell-seeded matrices are scaffolds that are seeded in vitro with autologous cells that have been appropriately cultured. The goal of both technologies is to deliver an implant that has the functional characteristics of the human bladder, while carrying a low-safety risk by having low immunogenic and low tumorigenic potential. A study published by Jayo and colleagues reported on the use of cell-seeded and unseeded biodegradable polyglycolic acid scaffolds for large bladder replacement procedures in the canine subtotal cystectomy model. The early stromal and cellular events occurring after implantation were studied in an attempt to determine whether cell-seeded constructs promoted regeneration better than matrices alone. The constructed neobladders of the cell-seeded group demonstrated predominance of myogenic precursors associated with regeneration, whereas the bare matrix tissues demonstrated a predominance of collagen, which is associated with scar formation rather than regeneration. The seeded group outperformed the unseeded group, with resultant bladders having a greater capacity and near-normal bladder compliance. The tissue in the seeded group also appeared more similar to bladder tissue histologically, as it had a urothelial layer, a suburothelial layer, and a smooth muscle layer. The unseeded scaffolds resulted in re-epithelialization of the graft lumen with fibrosis, graft contraction, and small bladder capacity. These findings led to a conclusion that seeded scaffolds are superior to unseeded, particularly when demand for a large portion of bladder tissue exists, because they promote a regenerative process as opposed to a reparative process.
Harvesting cells from the organ of interest and culturing them has become an important tool in tissue engineering. Autologous bladder cells are harvested from the diseased organ, separated into cell lines of interest, and expanded in vitro to sufficient quantities. They can then be seeded onto matrices to create tissue for implantation back into the host. Previous work has demonstrated that in certain populations, such as in patients with a neurogenic bladder, cells can be safely and effectively harvested and cultured. However, the search for cells that possess more characteristics of healthy tissue and have more regenerative potential continues. Smooth muscle cells from neuropathic bladders have shown abnormal growth, less contractile ability, and inferior adherence compared with normal controls, making them less than ideal cells for culture in this particular population. Other sources of cells are also important, because often, the diseased bladder does not contain enough normal cells to begin expansion. The patient’s own cells may also not be desirable for creation of new bladder tissue, as is the case in patients with previously demonstrated bladder malignancy. Multiple possibilities for sources of cells have been investigated, including stem cells and differentiated cells from organs other than the bladder; however, to date, autologous bladder cells remain the gold standard for culture and seeding.
Alternative sources of cells
Stem cells hold great promise when autologous cells from the bladder cannot be harvested. The 2 characteristics that define stem cells are self-renewal, or the ability to divide and produce identical cells, and potency, the ability to differentiate into specialized cells. Totipotent stem cells that are formed when an egg and sperm merge can differentiate into embryonic and extraembryonic cell types and can construct a complete, viable organism. Stem cells that can differentiate into cells from all 3 germ layers are descendants of totipotent cells and are called pluripotent, whereas the ones that are more restricted are multipotent. The use of stem cells avoids graft rejection and use of long-term medications needed with allogenic transplantation. Much research has been performed in the area of in vitro stem cell differentiation to produce smooth muscle and urothelial derivatives.
Pluripotent stem cells are of great interest in tissue engineering because of their unlimited self-renewal potential and their ability to differentiate into any cell type of endodermal, mesodermal, or ectodermal lineage. The isolation of pluripotent cells, called embryonic cells, from the inner cell mass of the blastocysts of mice was first performed by Evans and Kaugman in 1981. Subsequently, techniques have been developed to direct these cells to differentiate into specific tissue types. Human embryonic stem cells were isolated in 1998 from unused in vitro fertilization embryos. Sixty cell lines were initially developed from these embryos, 21 of which exist today and are viable and approved for funding by the National Institute of Health. Subsequently, other cell lines have also been developed nationally and internationally. Human embryonic stem cells are surrounded by ethical and moral concerns because of the need for destruction of human embryos to develop these cell lines. Their use has prompted a reevaluation of what, in fact, constitutes the beginning of human life, which is beyond the scope of this article.
Two types of nuclear cloning have been described and developed. Further elucidation of their definition could help in the development of guidelines and laws for the use of embryonic cell lines. Reproductive cloning is used to generate an embryo with the identical genetic make up of its source and is banned in most countries. Therapeutic cloning, otherwise called somatic cell nuclear transfer, uses a donor oocyte with its nuclear material removed and replaced with nuclear material from a donor cell. The autologous stem cell lines developed using this technique have the potential to become any type of cell in the adult body. Currently, this technology is still inefficient and limited. The ethical and social issues associated with embryonic stem cells are complex, and guidelines and laws surrounding their use need to be developed if their use is to become clinically widespread. Issues apart from the ethical and legal ones exist, which have halted the routine use of embryonic stem cells. Their ability to proliferate indefinitely is an important reason that embryonic cells are so attractive but also feared, because this uncontrolled proliferation potential could be associated with tumorigenesis and teratoma formation in the recipient.
A new and exciting advancement in stem cell research involves genetic reprogramming. This technique involves the dedifferentiation of adult somatic cells to produce patient-specific pluripotent stem cells or induced pluripotent state cells. This technique was first described in 2006; however, cells were shown to be incompletely reprogrammed in that pilot study. Subsequent studies were performed using human rather than mouse cells and revealed complete reprogramming of cells. There being no need for embryos, the ethical and social issues related to embryonic stem cells are avoided.
An alternate source of stem cells is the amniotic fluid and the placenta. Multiple partially differentiated cell types derived from the fetus have been identified in the amniotic fluid and are referred to as amniotic fluid and placental stem cells. They could be obtained via amniocentesis, chorionic villi sampling, or from the placenta at the time of birth. The cells could be banked to provide a source for autologous therapy later in life or for matching of histocompatible donor cells with recipients. These cells express embryonic and adult stem cell markers. They have been shown to retain long telomeres and a normal karyotype for more than 250 population doublings, making them genetically stable. Thus, they lack the teratoma-forming characteristics of embryonic stem cells, although maintaining the ability to differentiate into cells of all 3 embryonic germ layers. However, additional studies must be conducted to better characterize their differentiation potential and their limitations, as well as to characterize their malignancy potential.
Urine has also been investigated as a potential source of cells and would be an attractive option, because it would provide cells for tissue regeneration in a noninvasive manner. Recent studies have isolated cells from adult urine samples with progenitor cell features and the potential to differentiate into several bladder cell lineages, including cells with urothelial, smooth muscle, endothelial, and interstitial cell markers. These urine progenitor cells comprise only 0.2% of urine cells, but these cells that are found in sparse numbers may hold great promise for future tissue regeneration. The cells are easily cultured, appear genetically stable after several passages, and maintain their ability to give rise to more differentiated cells. They express markers, such as c-Kit, SSEA4, and CD44, which are associated with stem cells. The use of these cells also avoids ethical issues, because they are autologous somatic cells; however, their self-renewal potential has not yet been clearly defined.
Adult stem cells are a potential source of smooth muscle cells for bladder tissue engineering. They are particularly attractive, because they avoid the ethical problems associated with embryonic stem cells. However, they are found only in sparse numbers in the host, do not expand well in cultures, and have a more restricted differentiation potential. Adult stem cells have been isolated from bone marrow, skeletal muscle, adipose tissue, lung, testis, umbilical cord, and placenta. Mesenchymal stem cells are found in the bone marrow and have been studied as a source of bladder cells for seeding of matrices. These cells could be especially helpful in engineering bladders for patients with bladder cancer who need bladder augmentation, because the bone marrow is not a target for bladder cancer metastases, making these cells safe for use in this patient population. These cells have been shown to have the capability to differentiate into a large number of cells and the ability to differentiate into various specialized cell types under appropriate conditions. Expansion and differentiation of bone marrow mesenchymal stem cells varies with external signals and with the environment in which they are grown. Recently, bone marrow mesenchymal cells have been shown to be able to differentiate into mesodermal and endodermal cell lines, and a recent study was conducted to determine their potential to differentiate into multiple cell types. This study showed that bone marrow-derived mesenchymal stem cells demonstrated urothelium-specific genes and proteins , including uroplakin-Ia, cytokeratin-7, and cytokeratin-13. The urothelium-derived medium contained several growth factors, including endothelial growth factor, platelet-derived growth factor (PDGF-BB), transforming growth factor (TGF-B1), and vascular endothelial growth factor (VEGF). However, the cells demonstrated smooth muscle cell genes and proteins when cocultured with smooth muscle cells or in a smooth muscle cell medium, specifically desmin and myosin. Several growth factors were detected in this growth medium as well, and included hepatocyte growth factor, PDGF-BB, TGF-B1, and VEGF. Scaffolds have proven useful for expansion of bone-marrow mesenchymal cells. These have included natural small intestine submucosa and synthetic 3-dimensional (3D) nanofibrous scaffolds, such as poly-L-lactic acid polymer. Further studies are needed to evaluate whether these cells are transformed permanently and to characterize the physical properties of the engineered tissue.
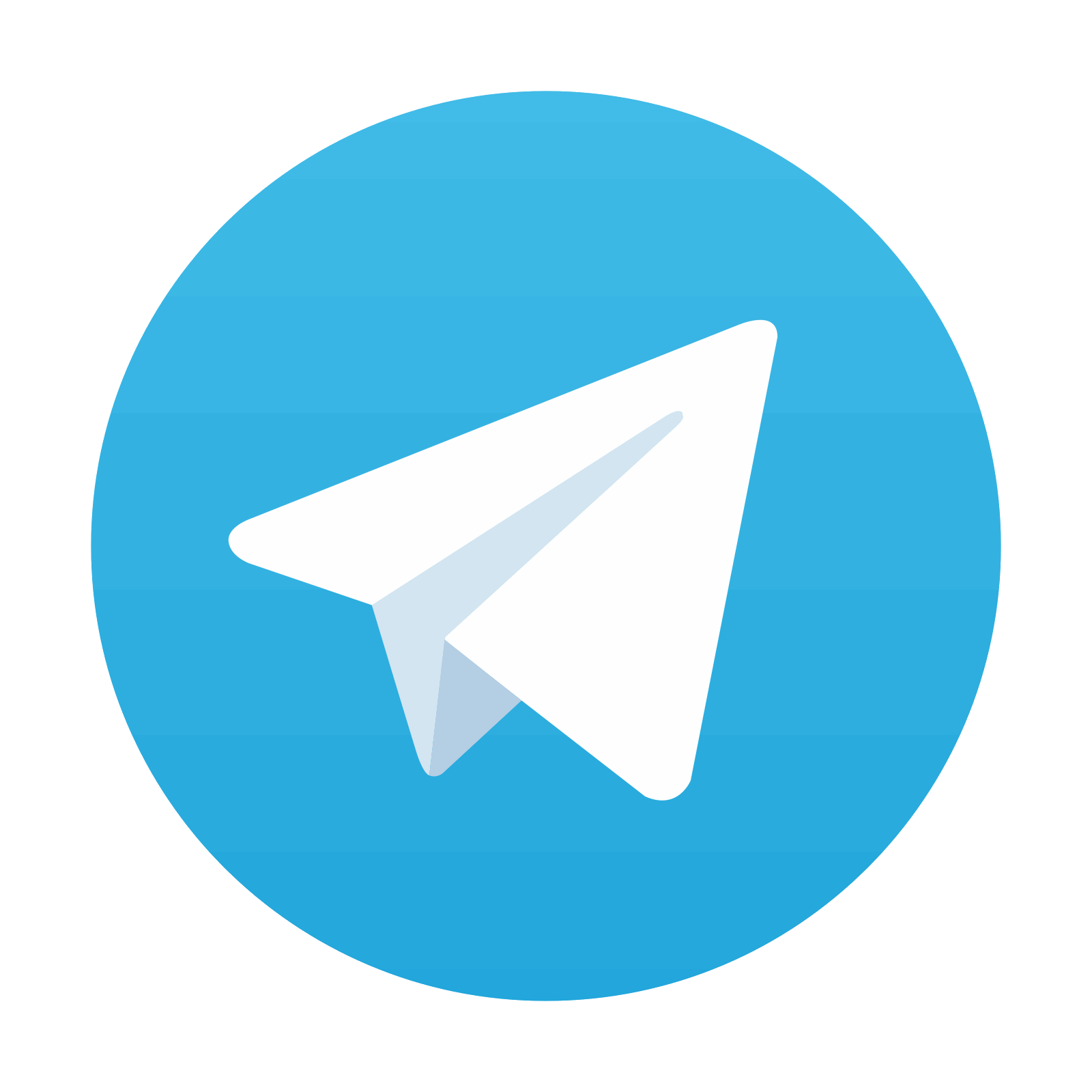
Stay updated, free articles. Join our Telegram channel

Full access? Get Clinical Tree
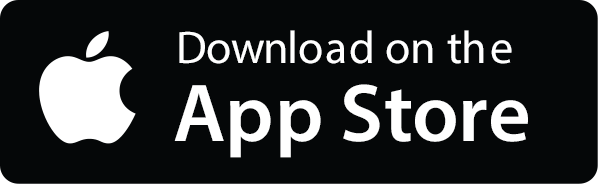
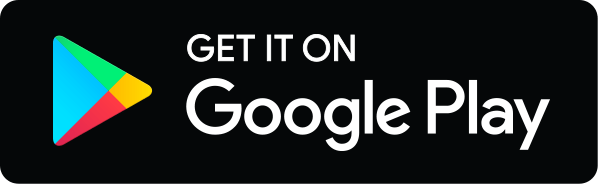