Bile acids are important in the processing of dietary lipids and serve three major functions. They aggregate and form micelles in the upper small intestine, which help solubilize lipolytic products, cholesterol, and fat-soluble vitamins, thereby facilitating absorption across the intestinal epithelium. During their secretion across the biliary canaliculus, bile acids stimulate bile flow. Finally, bile acids are major regulators of sterol metabolism and serve as a major excretory pathway for cholesterol from the body.
Bile acids undergo an enterohepatic circulation within the liver, biliary tract, intestinal tract, and portal and peripheral circulation. This carefully regulated enterohepatic circulation allows for conservation of bile acids. Any alteration in this circulatory pathway can lead to either a loss of bile acids from the body or displacement from the gastrointestinal tract with associated clinical manifestations. This chapter first reviews the normal bile acid physiology and discusses the clinical manifestations of defects of bile acid biosynthesis and clinical conditions associated with alterations in bile acid transport in the liver and gastrointestinal tract.
Biosynthesis
The two primary bile acids, cholic acid (CA; 3α,7α,12α-trihydroxy-5β-cholanoic acid) and chenodeoxycholic acid (CDCA; 3α,7α-dihydroxy-5β-cholanoic acid), are synthesized in the liver from cholesterol ( Figure 3-1 ). The synthesis of these acids occurs through a tightly regulated enzymatic cascade within hepatocytes involving at least 14 different enzymes. Modifications to the cholesterol nucleus occur via two different biosynthetic pathways: the classic, or neutral, pathway and the alternative, or acidic, pathway. Both pathways work to convert a hydrophobic cholesterol molecule into hydrophilic primary bile acids.

The neutral pathway of bile acid biosynthesis involves the formation of a cholic acid-to-chenodeoxycholic acid ratio of approximately 1:1. The initial step of cholesterol synthesis in the neutral pathway involves the 7α-hydroxylation of cholesterol by the rate-limiting enzyme, cholesterol 7α-hydroxylase. Compared with the neutral pathway, the alternative pathway of bile acid biosynthesis predominantly yields CDCA, with smaller amounts of CA. Although the neutral pathway is believed to be the quantitatively more important pathway of bile acid synthesis, the alternative pathway is likely more functional early in life, and alterations in this pathway may have devastating consequences.
Most primary bile acids are conjugated with either glycine or taurine after synthesis by hepatocytes. This conjugation effectively decreases the permeability of bile acids to cholangiocyte cellular membranes, thereby delivering higher concentrations to the intestines. Conjugation also inhibits digestion of bile acids by pancreatic carboxypeptidases and absorption in the proximal small intestine.
Enterohepatic Circulation
The bile acid pool in humans is typically made up of the primary bile acids, cholic and chenodeoxycholic acid, and the secondary bile acids, deoxycholic and lithocholic acid. Ursodeoxycholic acid (UCDA) accounts for only 1% to 3% of the bile acid pool. This pool of bile acids circulates through the liver, biliary tract, intestine, portal circulation, and peripheral serum in response to meal stimuli. Maintenance of a pool of bile acids is essential to normal fat absorption and bile secretion.
For adults and children beyond infancy, newly synthesized bile acids account for approximately 20% to 25% of the total bile acid pool. This percentage can be greatly increased in patients with impaired bile acid reabsorption, as found in patients with Crohn’s disease or necrotizing enterocolitis who have had ileal resection. Once synthesized by hepatocytes, bile acids are excreted into the canalicular lumen. In addition to bile acids, a sodium ion is excreted, which creates a gradient to passively draw water into the biliary canaliculi. This flow of bile acids and water serves as the major stimulus for bile flow. Although bile acids make up the major solute of bile, other components include phospholipids, organic anions, inorganic anions (especially chloride), and cholesterol.
Most of the bile acids secreted from the liver are stored in the gallbladder as mixed micelles accompanied by phospholipid and cholesterol. On consumption of a meal, the gallbladder contracts and bile acid micelles are delivered to the small intestine ( Figure 3-2 ). In the proximal small bowel, bile acids form mixed micelles with dietary lipolytic products, fatty acids, and monoglycerides. Cholesterol, phospholipids, and fat-soluble vitamins are also solubilized in a similar manner. The lipolytic products are absorbed in the proximal small intestine with reabsorption of bile acids in the distal intestine. Bile acids may be reabsorbed by either passive nonionic diffusion along the length of the gastrointestinal tract or by a sodium-dependent mechanism in the ileum. Reabsorption is limited in the upper small bowel because the p K a (acid dissociation constant) of bile acids tends to be too low for them to be absorbed by nonionic diffusion, although there is some absorption of unconjugated and glycine-conjugated bile acids.

On initial entry into the small intestines, bile acids have a net negative charge. As the bile acids pass through the more distal small intestine, they are deconjugated by the colonized bacteria. This deconjugation confers a neutral charge on the bile acids and thus permits rapid uptake by intestinal endothelial cells via passive diffusion. The combination of both passive and active reuptake of bile acids provides a very efficient method of recycling bile acids in humans. With each of the 8 to 12 enterohepatic cycles every day, there is a loss of approximately 3% to 5% of the pool of bile acids with each cycle, largely due to efficient absorption by the combination of passive and active transport systems in the intestine.
A fraction of bile acids in the pool escapes reabsorption in the small intestine and is delivered to the large intestine where bacterial transformation of the bile acids occurs. After conjugated bile acids are deconjugated, bacterial 7α-dehydroxylation of CA and CDCA may occur, causing formation of the secondary bile acids deoxycholic acid (3α,12α-dihydroxy-5β cholanoic acid) and lithocholic acid (3α-hydroxy-5β cholanoic acid) (see Figure 3-1 ).
A small amount of bile acids is lost in the stool each day. Although the amount varies by diet and individual, in the adult, up to 30 g of bile acids are reabsorbed by the intestines, with 0.2 to 0.6 g being eliminated in the stool daily. The bile acids lost in the stool are replaced by newly synthesized bile acids in the liver through a tightly controlled negative feedback system. The rate-limiting enzyme for bile acid synthesis in the neutral pathway, cholesterol 7α-hydroxylase, is tightly regulated by feedback inhibition from the bile acids returning to the liver through the nuclear receptor, farnesoid X receptor (FXR). This feedback inhibition mechanism ensures that the bile acid pool remains constant in healthy humans, with adequate bile acids to promote bile flow, micelle formation, and cholesterol excretion.
Bile acids enter the portal venous system through absorption by intestinal endothelial cells. These bile acids are bound to albumin and other proteins as they are transported in the portal vein to the liver. Up to 90% of these bile acids are removed by the liver during their first pass. Most of the reuptake is performed by periportal hepatocytes, which then secrete the bile acids into the canalicular space, the rate-limiting step of bile acid transport. A small fraction of the circulating bile acids in the portal blood escapes removal by the hepatocytes and spills over into the systemic circulation. Therefore, with each cycling of bile acids there is a characteristic small spillover of bile acids in the serum that can be measured. The postprandial rise of bile acids serves as a reasonable indicator that the enterohepatic circulation is intact. The serum bile acids undergo filtration by the kidney and can be either excreted in the urine or reabsorbed in the renal tubules for transport back to the liver.
Maturation of the Enterohepatic Circulation
Neonates are born with an immature enterohepatic circulation of bile acids. A maturation process occurs within the fetal liver and continues throughout the first year of life; this process effectively increases the amount of bile acids available for digestion. Bile acid synthesis has been demonstrated as early as the 12th week of gestation. The bile acids produced throughout gestation are different from those produced by infants, children, and adults. Whereas the primary bile acids, CA and CDCA, make up approximately 75% to 80% of the biliary bile acid pool in adults, they make up less than 50% of the total bile acid pool in the fetus. An immature synthetic pathway of bile acids exists in the developing fetus that not only leads to a decreased rate of bile acid synthesis, but also to the production of “atypical” bile acids not seen in the healthy child or adult. These “atypical” bile acids have additional sites of hydroxylation, which may be important in the pathogenesis of cholestatic liver disease in the neonate.
Although newborns initially have a decreased synthesis of bile acids and decreased bile acid pool size, both increase during the first several months of life. The decreased size of the bile acid pool is accompanied by a reduced concentration of intraluminal bile salts. When corrected for differences in body surface area, both term and preterm normal newborn infants have reduced rates of cholate synthesis and a reduced bile acid pool size compared to normal adults. In vitro studies suggest that ileal bile acid transport is decreased in human newborns. In addition to the impaired synthesis and ileal uptake of bile acids in newborns, the pressure generated by contraction of the newborn gallbladder may be insufficient to overcome the choledochal resistance to bile flow. For preterm infants younger than 33 weeks of gestation, the gallbladder contraction index may be nonexistent to less than 50%. Impaired gallbladder contraction may explain why 0.5% of normal neonates have gallstones or gallbladder sludge. A decrease in intraluminal bile salt concentration in the neonate contributes to a phenomenon of decreased fat absorption known as physiologic steatorrhea. Over the first months of life, the bile acid synthetic rate increases and the pool expands, with concurrent increase in intraluminal bile acid concentrations.
Despite a decreased rate of bile acid synthesis and decreased bile acid pool size, the serum bile acid concentration is typically increased in normal preterm and term newborn infants. In fact, the serum bile acid concentration during the first 6 months of life is as high as in adults who have clinical cholestasis. The elevated serum bile acids during this period has been termed physiologic cholestasis. The early elevation in serum bile acids relates to a poor hepatic extraction of bile salts from the portal circulation. This hepatic uptake is especially impaired in preterm infants. An improvement in the hepatic uptake of bile acids occurs over the first year of life and corresponds to a decrease in the peripheral serum bile acid concentration. Levels of serum bile acids in infants decrease into the normal range by approximately 10 months of age.
The bile acid composition in neonates is predominantly the primary bile acids, CA and CDCA. The secondary bile acids, lithocholic acid and deoxycholic acid, appear in both the serum and bile of infants undergoing intestinal microflora colonization. As the infant matures, primary and secondary bile acids continue to be synthesized and recirculated. The concentration of bile acids in humans eventually approximates the following: cholic acid (36%), chenodeoxycholic acid (36%), deoxycholic acid (24%), and lithocholic acid (1%) ( Figure 3-3 ).

Alterations in the Enterohepatic Circulation
Disruptions in any part of the enterohepatic circulation of bile acids can lead to the development of clinical manifestations ranging from cholestasis to diarrhea. Alterations may occur at the level of primary bile acid synthesis, in the transport of bile acids across the hepatocyte, at the level of secondary bile acid synthesis, or in ileal transport and the recirculation of bile acids.
Alteration of Primary Bile Acid Biosynthesis
Disorders in bile acid synthesis and metabolism can be broadly classified as primary or secondary. Primary enzyme defects involve congenital deficiencies in enzymes responsible for catalyzing key reactions in the synthesis of CA and CDCA. The primary defects include cholesterol 7-hydroxylase (CYP7A1) deficiency, 3β-hydroxy-C 27 -steroid oxidoreductase deficiency, Δ 4 -3-oxosteroid 5β-reductase deficiency, oxysterol 7α-hydroxylase deficiency, 27-hydroxylase deficiency (or cerebrotendinous xanthomatosis [CTX]), 2-methylacyl-CoA (coenzyme A) racemase deficiency, trihydroxycholestanoic acid–CoA oxidase deficiency, amidation defects involving a deficiency in the bile acid–CoA ligase, and side-chain oxidation defect in the 25-hydroxylation pathway for bile acid resulting in an overproduction of bile alcohols. Secondary metabolic defects that affect primary bile acid synthesis include peroxisomal disorders such as cerebrohepatorenal syndrome of Zellweger and related disorders, and Smith-Lemli-Opitz syndrome. The biochemical presentation of these bile acid synthetic defects includes a markedly reduced or complete lack of CA and CDCA in the serum, bile, and urine, and greatly elevated concentrations of atypical bile acids and sterols that retain the characteristic structure of the substrates for the deficient enzyme and may have intrinsic hepatotoxicity. These signature metabolites are generally not detected by the routine or classic methods for bile acid measurement, and mass spectrometric techniques presently provide the most appropriate means of characterizing defects in bile acid synthesis. Screening procedures using liquid secondary ionization mass spectrometry (LSIMS), formerly known as fast atom bombardment-mass spectrometry (FAB-MS), indicate that inborn errors in bile acid synthesis probably account for 1% to 2% of the cases of samples sent for analysis from infants, children, and adolescents.
Cerebrotendinous Xanthomatosis
CTX is a rare inherited lipid storage disease with an estimated prevalence of 1 in 70,000. Characteristic features of the disease in adults include progressive neurologic dysfunction, dementia, ataxia, cataracts, and xanthomata in the brain and tendons, and in infants includes neonatal cholestasis (K. D. R. Setchell, unpublished data, 2003). Biochemically, the disease can be distinguished from other conditions involving xanthomata by the following: (1) significantly reduced primary bile acid synthesis; (2) elevations in biliary, urinary, and fecal excretion of bile alcohol glucuronides; (3) low plasma cholesterol concentration, with deposition of cholesterol and cholestanol in the tissues; and (4) marked elevations in cholestanol. The elevation in 5α-cholestan-3βol (cholestanol) in the nervous system of patients with CTX and the high plasma concentrations of this sterol are unique features of the disease. Point mutations in the gene located on the long arm of chromosome 2 that lead to inactivation of the sterol 27-hydroxylase have been identified. Neonatal presentation may include elevated serum alanine aminotransferase (ALT), aspartate aminotransferase (AST), and conjugated bilirubin with normal serum γ-glutamyl transpeptidase (GGT), with biochemical abnormalities normalization by about age 6 months. The liver histopathologic findings in these young patients are similar to those observed in idiopathic neonatal hepatitis.
3β-Hydroxy-C 27 -Steroid Oxidoreductase Deficiency
This was the first metabolic defect to be described involving an early step in the bile acid biosynthetic pathway; 7α-hydroxycholesterol is converted to 7α-hydroxy-4-cholesten-3-one, a reaction catalyzed by a 3β-hydroxy-C 27 -steroid oxidoreductase. This is the most common of all of the bile acid synthetic defects described to date. Although the clinical presentation of this disorder is somewhat heterogeneous, most patients present as neonates with elevated serum ALT and AST levels, a conjugated hyperbilirubinemia, and normal serum GGT level. Clinical features include hepatomegaly, with or without splenomegaly, fat-soluble vitamin malabsorption, and mild steatorrhea; in most instances, pruritus is absent. The liver histologic findings are those of hepatitis, the presence of giant cells, and cholestasis. The heterogeneity in clinical course of those with early onset disease is illustrated by some patients, who initially resolve their jaundice and are identified later in life, and others with more fulminant disease, eventuating in death or transplantation at an early age. Although the earliest cases were identified in infants, increasingly, idiopathic late-onset chronic cholestasis has been explained by this disorder. In such patients, liver disease is not always evident initially, and patients may have fat-soluble vitamin malabsorption and rickets, which are corrected with vitamin supplementation. Serum liver enzymes that are often normal in the early stages of the disease later show progressive increases with evidence of progressive hepatic fibrosis. Definitive diagnosis of the 3β-hydroxy-C 27 -steroid oxidoreductase deficiency presently requires mass spectrometric analysis of biologic fluids and is readily accomplished by LSIMS, or by electrospray and tandem mass spectrometry. Molecular techniques that have led to the cloning of the HSD3B7 gene encoding 3β-hydroxy-C 27 -steroid oxidoreductase now permit the accurate genetic basis of the defect. Treatment with cholic acid (available under an Investigational New Drug application [IND] from the U.S. Food and Drug Administration[FDA]) leads to gradual resolution of biochemical and histologic abnormalities with an excellent long-term prognosis.
Δ 4 -3-Oxosteroid 5β-Reductase Deficiency
Application of LSIMS for urine analysis led to the discovery of a defect in Δ 4 -3-oxosteroid 5β-reductase, which catalyzes the conversion of the intermediates 7α-hydroxy-4-cholesten-3-one and 7α,12α-dihydroxy-4-cholesten-3-one to the corresponding 3-oxo-5β (H) intermediates. The clinical presentation of this defect is similar to that of patients with the 3β-hydroxy-C 27 -steroid oxidoreductase deficiency; however, in contrast, the GGT level is usually elevated, and the average age at diagnosis is lower in patients with Δ 4 -3-oxosteroid 5β-reductase deficiency. Infants with Δ 4 -3-oxosteroid 5β-reductase deficiency tend to have more severe liver disease with rapid progression to cirrhosis and death without intervention. The Δ 4 -3-oxosteroid 5β-reductase deficiency has since been found in a number of patients presenting with neonatal hemochromatosis. Infants with Δ 4 -3-oxosteroid 5β-reductase deficiency present with elevations in serum ALT and AST levels, markedly elevated serum conjugated bilirubin, and coagulopathy. Liver histologic and ultrastructural pathology findings include marked lobular disarray as a result of giant cell and pseudoacinar transformation of hepatocytes, hepatocellular and canalicular bile stasis, and extramedullary hematopoiesis with small bile canaliculi that are sometimes slitlike in appearance and show few or absent microvilli containing electron-dense material. Increased production of Δ 4 -3-oxo bile acids occurs in patients with severe liver disease and in infants during the first few weeks of life. It is important to perform a repeat analysis of urine in the case of a suspected Δ 4 -3-oxosteroid 5β-reductase deficiency because on rare occasions a resolution of the liver disease occurs and the atypical bile acids disappear. The liver injury in this defect is presumed to be the consequence of the diminished primary bile acid synthesis and the hepatotoxicity of the accumulated Δ 4 -3-oxo bile acids. The lack of canalicular secretion can be explained by the relative insolubility of oxo-bile acids and the cholestatic effects of the taurine conjugate of 7α-dihydroxy-3-oxo-4-cholenoic acid. Treatment with UDCA or CA leads to resolution of histologic and biochemical abnormalities, with an excellent long-term prognosis.
Oxysterol 7α-Hydroxylase Deficiency
The recent discovery of a genetic defect in oxysterol 7α-hydroxylase establishes the acidic pathway as a quantitatively important pathway for bile acid synthesis in early life. In humans, oxysterol 7α-hydroxylase may be more important than cholesterol 7α-hydroxylase for bile acid synthesis in early life. This defect has been reported in a 10-week-old boy, whose parents were first cousins, who presented with severe progressive cholestasis, hepatosplenomegaly, cirrhosis, and liver synthetic failure from early infancy. Serum ALT and AST levels were markedly elevated and serum GGT level was normal. Liver biopsy findings included cholestasis, bridging fibrosis, extensive giant cell transformation, and proliferating bile ductules. Oral UDCA therapy led to deterioration in liver function tests, and oral CA was ineffective. The patient subsequently died after orthotopic liver transplantation at age months. The accumulating monohydroxy bile acids with the 3β-hydroxy-Δ 5 structure have been shown previously to be extremely cholestatic. Their hepatotoxicity in this patient is presumed to have been exacerbated by the lack of primary bile acids necessary for the maintenance of bile flow. The patient was homozygous for this nonsense mutation, whereas both parents were heterozygous.
2-Methylacyl-CoA Racemase Deficiency
2-Methylacyl-CoA racemase is a crucial enzyme that is uniquely responsible for the racemization of (25 R )THCA-CoA to its (25 S ) enantiomer, while also performing the same reaction on the branched-chain fatty acid (2 R )pristanoyl-CoA. Defects in this enzyme have profound effects on both the bile acid and fatty acid pathways. Mutations in the gene encoding 2-methylacyl-CoA racemase were first reported in three adults who presented with a sensory motor neuropathy, and later in a 10-week-old infant who had severe fat-soluble vitamin deficiencies, hematochezia, and mild cholestatic liver disease. Liver histologic findings included cholestasis and giant cell transformation with modest inflammation. The infant had the same missense mutation (S52P) as that described in two of the adult patients, yet was seemingly phenotypically different. Two of the adult patients had neurologic symptoms caused by tissue accumulation of phytanic and pristanic acids, but were asymptomatic until the fourth decade of life, whereas the other adult was described as having the typical features of Niemann-Pick type C disease at 18 months of age and presumably had some liver dysfunction. In the first infant described with the 2-methylacyl-CoA racemase deficiency, the liver from a -month-old sibling, who 2 years previously had died from an intracranial bleed. The mass spectrum and gas chromatography (GC) profiles in this defect resemble closely those observed in peroxisomal disorders affecting bile acid synthesis, such as Zellweger syndrome. Primary bile acid therapy with CA has proven effective in normalizing liver enzymes and preventing the onset of neurologic symptoms in infants. In addition, dietary restriction of phytanic acid and pristanic acids is likely to be necessary in the long term for such patients to prevent neurotoxicity from accumulation of these fatty acids in the brain.
THCA-CoA Oxidase Deficiency
A number of patients have been reported to have side-chain oxidation defects involving the THCA-CoA oxidase. The clinical presentation differs among these cases, and although all have an impact on primary bile acid synthesis, neurologic disease was the main clinical feature. Whether these are primary bile acid defects or secondary to single-enzyme defects in peroxisomal β-oxidation is unclear. Two distinct acyl-CoA oxidases have been identified in humans. The human acyl-CoA oxidase active on bile acid C 27 cholestanoic acid intermediates has been found to be the same enzyme that catalyzes the oxidation of 2-methyl branched-chain fatty acids. THCA-CoA oxidase deficiency has been shown to be associated with elevated serum phytanic and pristanic acids. All patients had ataxia as a primary feature of the disease, with its onset occurring at about years of age. None had evidence of liver disease. It is possible, with the exception of the patient described by Clayton and colleagues, that these patients had a 2-methylacyl-CoA racemase deficiency, but the analysis of the cholestanoic acids was not sufficiently detailed to permit the diastereoisomers of THCA and 3α,7α-dihydroxy-5β-cholestanoic acid (DHCA) or pristanic acid to be measured.
Bile Acid–CoA Ligase Deficiency and Defective Amidation
The final step in bile acid synthesis involves conjugation with the amino acids glycine and taurine. Two enzymes catalyze the reactions leading to amidation of bile acids. In the first, a CoA thioester is formed by the rate-limiting bile acid–CoA ligase, after which glycine or taurine is coupled in a reaction catalyzed by a cytosolic bile acid-CoA: amino acid N-acyltransferase (BAAT). A defect in bile acid amidation, presumed to involve the bile acid–CoA ligase, was described in patients presenting with fat and fat-soluble vitamin malabsorption. The index case was a 14-year-old boy of Laotian descent who, in the first 3 months of life, presented with conjugated hyperbilirubinemia, elevated serum transaminases, and normal GGT level. Subsequently, an additional six patients, who presented as toddlers or older children/adolescents, have been identified who have presented with a history of neonatal cholestasis, growth failure, or fat-soluble vitamin deficiency. The diagnosis is based on the LSIMS analysis of the urine, serum, and bile, which reveals a unique spectrum of unconjugated cholic acid and sulfate and glucuronide conjugates of dihydroxy and trihydroxy bile acids. All recently identified patients with this defect have been identified with family specific mutations in the bile acid–CoA ligase gene. Carlton et al. have described a kindred of Amish descent with mutations in BAAT. Patients homozygous for the 226G mutation had increased serum bile acids, variable growth failure and coagulopathy without jaundice, and normal serum GGT concentrations. Homozygotes had only unconjugated bile acids in serum, whereas heterozygotes had increased amounts unconjugated serum bile acids. Administration of conjugates of the primary bile acid, glycocholic acid, under an IND from the FDA to five recently identified patients has improved their growth and should correct the fat-soluble vitamin malabsorption in this defect.
Cholesterol 7α-Hydroxylase Deficiency
Several patients have recently been identified with a homozygous mutation deletion in the CYP7A1 gene, and when the complementary DNA (cDNA) of this mutant was expressed in vitro in cultured HEK 293 cells, cholesterol 7α-hydroxylase was found to be inactive. Bile acid synthesis was reduced, and up-regulation of the alternative sterol 27-hydroxylase pathway presumably compensated for the reduced synthesis of bile acids via absent cholesterol 7α-hydroxylase activity. Three patients carrying this mutation were found to have abnormal serum lipids; however, in contrast to an infant identified with a mutation in oxysterol 7α-hydroxylase, there was no liver dysfunction in these patients. Instead, the clinical phenotype was one of markedly elevated total and low-density lipoprotein (LDL) cholesterol and premature gallstones in two patients and premature coronary and peripheral vascular disease in one patient. The elevated serum cholesterol concentration was unresponsive to HMG-CoA reductase inhibitor therapy.
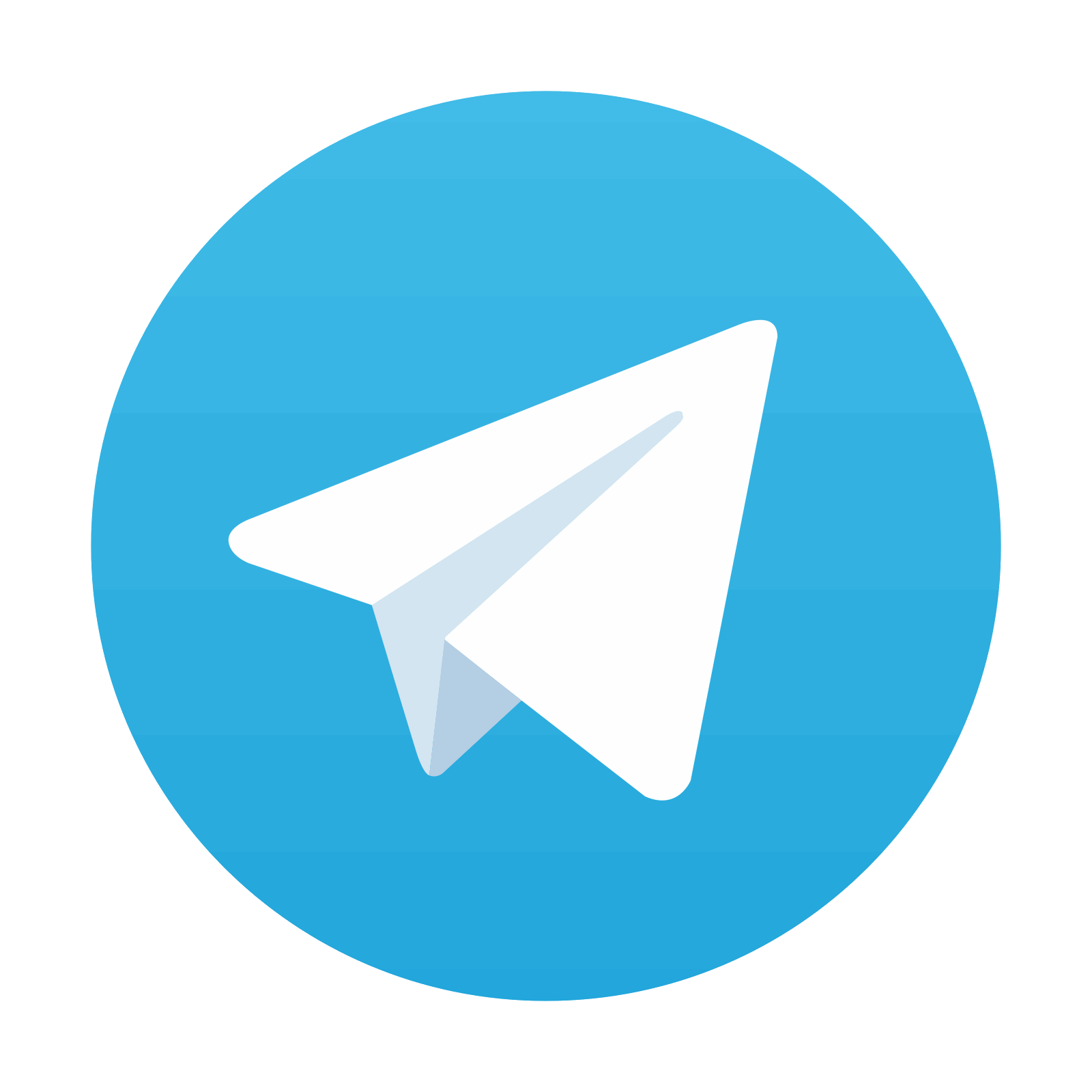
Stay updated, free articles. Join our Telegram channel

Full access? Get Clinical Tree
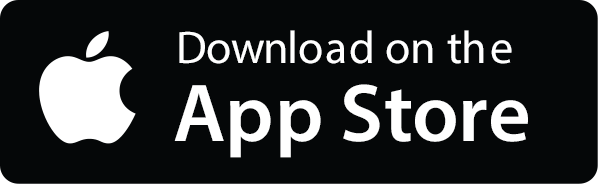
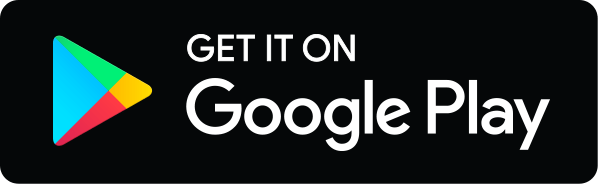