Fig. 12.1
Anatomy of the upper urinary tract demonstrating the anatomical restrictions in the upper urinary tract. The intramural ureter is compressed as it runs through the bladder wall and narrows considerably at the ureterovesical junction (1). By extrinsic compression of the iliac vessels, the crossing ureter is narrowed (2) and at the ureteropelvic junction (3) the proximal ureter is narrowed where it passes into the renal pelvis
In case of a suspect lesion, histopathological examination is mandatory. Biopsy specimens retrieved during ureterorenoscopy are frequently minute because of the application of small caliber instruments [2], and may therefore be difficult to examine by the pathologist. Consequently, a high rate of tumour upgrading (37–96 %) and upstaging (38 %) is reported following nephroureterectomy [3, 4]. Over the past decade, conservative endoscopic treatment has gained increased interest for a selected group of patients with upper urinary tract urothelial carcinoma (UUT-UC), not only in case of a mandatory indication (patients with a solitary kidney), but also for patients with low-volume, low-grade, low stage disease [5, 6] and a healthy contralateral kidney. Although radical nephroureterectomy (including bladder cuff) is the gold standard in the treatment of UUT-UC, estimated Glomerular Filtration Rate (eGFR) decreases significantly in patients with UUT-UC after nephroureterectomy [7–9] and chronic kidney disease (CKD) is associated with a significant increase in cardiovascular events and death of any cause, independent of co-existing comorbidities [10]. For this reason endoscopic treatment is more accepted in patients with low-risk disease.
To decide which patients are eligible for endoscopic treatment, information on tumour stage and grade has become imminent for clinical decision-making. Optimizing endoscopic visualization and accurate diagnosis of UUT-UC is therefore a prerequisite. Novel optical diagnostic techniques, based on the interaction of light with tissue, have the potential to improve this visualization and diagnosis of UUT-UC [11]. These interactions include scattering, absorption and fluorescence, all of which are characteristic for certain tissue types. Some of these techniques aim to provide real time intra-operative information on tumour grade and stage. If knowledge about tumour grade and stage is obtained during URS, a better selection of patients eligible for endoscopic treatment is possible and safe and simultaneous treatment can be applied. It is because of this promise that optical diagnostics might reduce the limitations of the current biopsies.
Most research on the application of optical diagnostics on urothelium has been done in the field of bladder cancer [12, 13]. Results on bladder urothelium resemble ureteral urothelium. However, the limited space in the ureter and the difficulty of reaching the upper urinary tract creates a whole new spectrum of challenges for the applied optical techniques. Given the speed of current technical developments and miniaturization, optical diagnostics have become available for the diagnostic work up of upper urinary tract investigation.
12.2 Endoluminal Ultrasound (ELUS)
Endoluminal Ultrasound (ELUS) is an imaging technique that can be applied in a variety of luminal structures. ELUS is based on detecting an echo time delay of high frequency sound waves, which are backscattered by structures in tissue. Plotting of the reflecting ultrasound amplitude vs depth composes endoluminal images. The most common examples of endoluminal ultrasound are intravascular ultrasound, transvaginal ultrasound and transrectal ultrasound. Advances of this technique has led to small catheter-based ultrasound probes allowing visualization of a variety of other luminal structures, such as bile ducts, fallopian tubes, small bowel, oesophagus and blood vessels [14, 15]. Current ultrasound probes consist of flexible 2.4 mm diameter (7.2 F) catheters containing a 12.5 or 20 MHz transducer (Olympus, Tokyo, Japan) [16]. ELUS probes designed for use in the upper urinary tract have a hole through the end for placement over a Terumo guide-wire. The ultrasound probes are re-usable and can be sterilized in the same way as standard endoscopy equipment. The ultrasound transducer is attached to a motor that continually rotates providing real-time 360° cross-sectional images of the ureteric wall and surrounding structures and a pullback allowing 3D imaging of a UUT segment. Imaging penetration depth is dependent on the frequency of the ultrasound transducer. The 12.5 MHz transducer enables axial imaging with a penetration depth of maximum 40 mm around the ureter. The 20 MHz transducer enables axial imaging with a penetration depth of maximal 20 mm from the centre of the transducer and provides an axial resolution of 100 μm (Olympus, Tokyo, Japan) [16].
The transmission of sound waves needs a conducting medium. This restricts imaging to fluid filled structures, such as the lumen of the upper urinary tract. However, air bubbles introduced accidentally in the irrigation water due to the procedure create large back scattering, disturbing signals. An advantage of endoluminal ultrasound is its deeper imaging depth compared to other endoluminal imaging techniques (Table 12.1) which can be helpful to determine the relation of specific pathologies with its direct surroundings, like increased lymph nodes in malignancies [16]. In the ureter, endoluminal ultrasound has mainly been used for the treatment of UPJ stenosis, when an endoluminal incision was considered and the location of crossing vessels had to be determined. By using ELUS, the exact location of crossing vessels can be determined and therefore the best incision plane can be chosen [17]. Other possible indications for ELUS are identification and staging of upper urinary tract tumours, submucosal calculi, endometriosis and inflammation [16, 18] (Fig. 12.2).
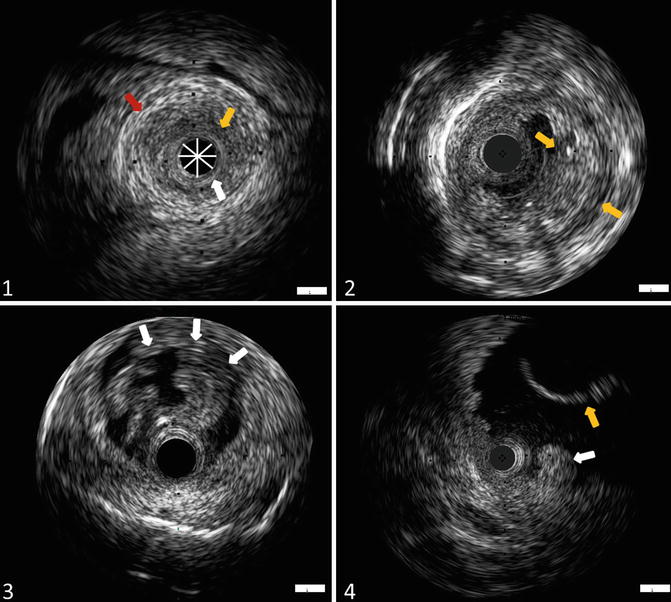
Table 12.1
Overview of the three endoluminal diagnostic techniques, including imaging depth and resolution
Principle | Purposes | Imaging depth | Resolution (μm) | Advantage | Limitations | |
---|---|---|---|---|---|---|
ELUS | Sound scattering | Real time imaging of luminal structures | 20–40 mm | 100 | High imaging penetration depth | Low resolution images movement artefacts low data aquisition speed |
OCT | Light scatteringc | Real time information on pathohistological diagnosis | 2–3 mm | 20 | Information on tumour grade and stage suitable for screening purposus of complete ureter fast data aquisition speed | Diminished imaging depth range |
CLE | Absorption/reflection | Real time information on pathohistological diagnosis | 400 μm | 3.5 | In-vivo microscopy with high resolution images | Sensitivity to tissue movement additional staining needed |
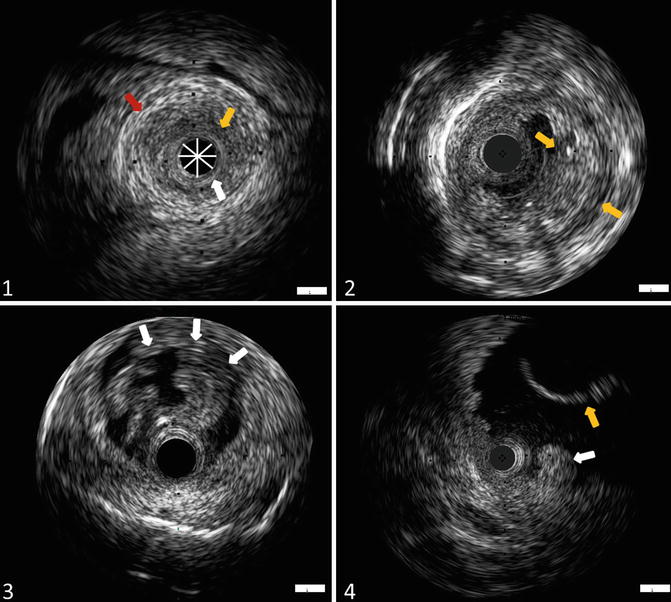
Fig. 12.2
Ex-vivo cross-sectional images of the ureter and pyelum using endoluminal ultrasound (ELUS). (1) ELUS image of normal appearing ureter, demonstrating inner hyperechoic mucosa (white arrow), hypoechoic muscularis (orange arrow) and hyperechoic periureteric fat (red arrow). The probe is indicated in this figure (*). (2) ELUS image of the ureter demonstrating solid urothelial carcinoma (T1G3) showing irregular thickened mucosal layer. (3) ELUS image of a TaG1 papillary urothelial carcinoma of the ureter (white arrows). (4) ELUS image of the pyelum, showing an invasive high-grade urothelial carcinoma (white arrow) and nephrostomy tube (orange arrow)
However, improvement of CT imaging has led to a decrease of the use of ELUS in the field of urology, because it is less invasive and considerable costs are involved.
12.3 Optical Coherence Tomography
Optical Coherence Tomography (OCT) is a high resolution imaging technology originally applied in ophthalmology [19]. OCT is analogous to ultrasonography, using back-scattered light instead of back-scattered sound waves to produce micrometer-scale resolution, cross-sectional images (Fig. 12.3). “State of the art” OCT research investigates the position of this relative novel technology in the diagnostic workup of several epithelial cancers [20, 21]. The use of optical fibers allows OCT to be applied endoluminally through the working channel of small calibre rigid and flexible endoscopes. The commercially available OCT imaging probe currently at use for upper urinary tract imaging is originally designed for cardiovascular applications. For this reason, the 0.9 mm (2.7 Fr) fiber has to be interfaced with an intravascular imaging system. This automatic pullback system scans a longitudinal trajectory of 54 mm in approximately 5.4 s, producing a 540-frame dataset at 20 μm axial resolution. In OCT images of the urinary bladder wall, layered tissue anatomy can be distinguished, e.g., the basement membrane, which integrity is indicative of low stage in case a bladder tumour is identified [22]. Additionally, the light scattering causes a decrease of OCT signal magnitude over depth, and limits the imaging range to approximately 2 mm depth. This rate of OCT signal decrease with depth is quantified by the attenuation coefficient (μoct) that allows in-vivo differentiation between different tissue types [21, 23–25]. This distinction results from differences in intra- and extracellular organization of the tissue, which is reflected in the light scattering properties. Measurement of μoct could therefore discriminate differences in organization that could be associated with different grades of the lesion. This has theoretically been described and tested in an animal study by Xie et al. [26], where they showed differences in scattering properties between normal and malignant bladder urothelium.
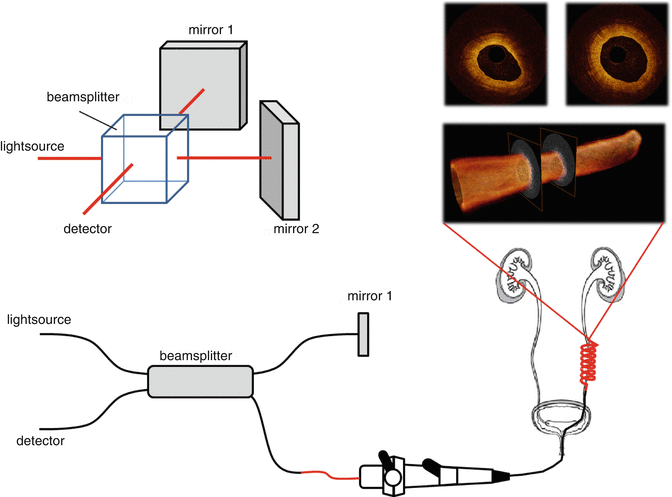
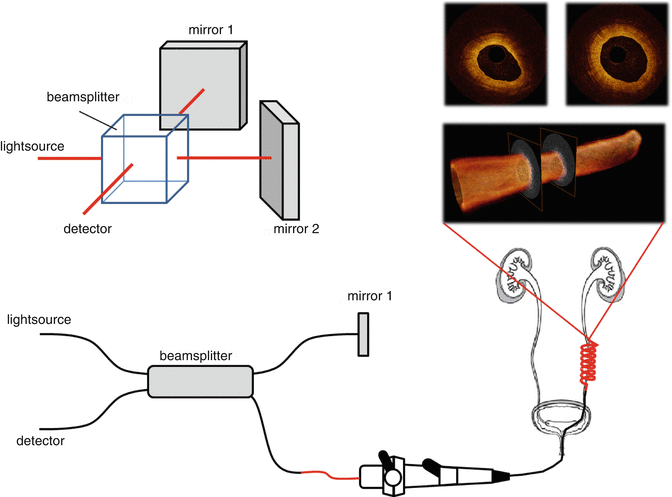
Fig. 12.3
Optical Coherence Tomography (OCT) is the optical equivalent of ultrasound imaging, measuring light reflectivity vs. depth. It is based on white light (large wavelength bandwidth) interferometry, where interference signals are only detected if the light in the sample and reference arm has travelled equal distances. Thus, by varying the length of the reference arm, the imaging location in the tissue can be controlled. Modern embodiments of OCT do not use moving reference mirrors; instead technical complexity is shifted towards either the light source (that sequentially provides each wavelength within the source bandwidth at high speed) or the detector (which detects each wavelength within the source bandwidth in parallel)
The potential of OCT for staging and diagnosis of UUT-UC has been investigated ex-vivo in the porcine ureter and these investigations demonstrated that OCT can clearly distinguish the ureteral wall layers, particularly the urothelium and lamina propria [27, 28]. When compared to endoluminal ultrasonography, OCT significantly better distinguishes the wall layers of ex-vivo porcine ureter [29]. An in-vivo human pilot study showed that normal appearing urothelium including basal membrane, CIS and visible protrusions could be visualized on OCT images (Fig. 12.4). Additionally, OCT is able to visually differentiate between non-invasive and invasive tumours (Fig. 12.5) and it can differentiate between low- and high-grade lesions by quantifying μoct [30]. However, current OCT analyses cannot obtain a reliable μoct from normal appearing urothelium or CIS due to the limited thickness of these layers (around 50 μm). Improvement by increasing the resolution of OCT could solve this limitation. Recently published novel attenuation analysis methods for thin layers could solve this limitation, but these methods do require additional research since it is still in an early stage of development [31].
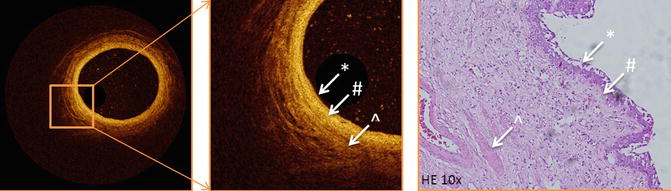
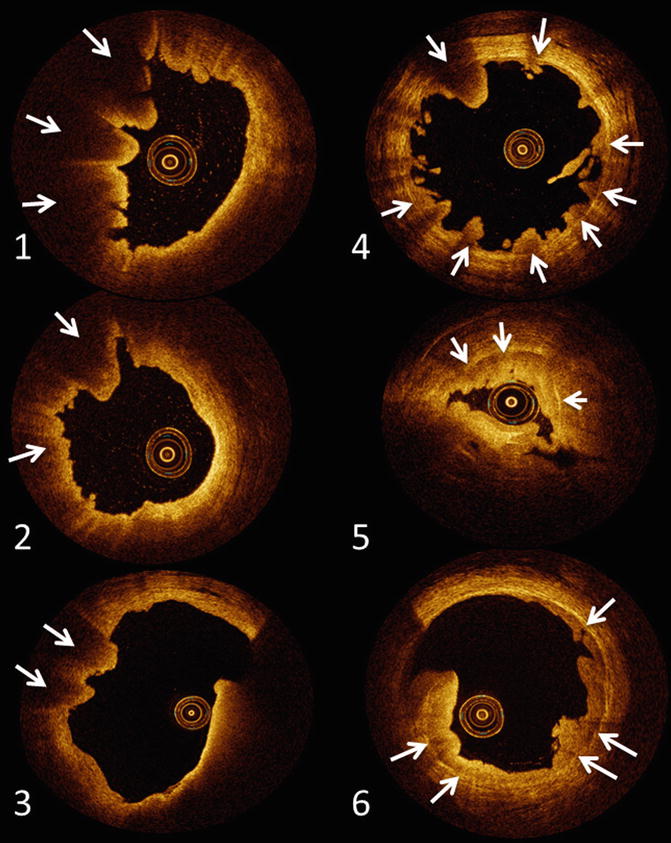
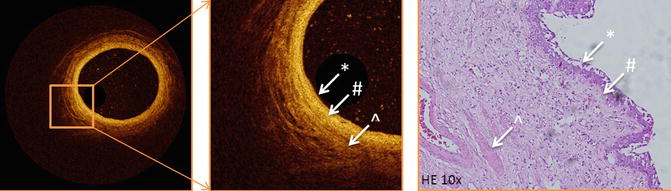
Fig. 12.4
Optical Coherence Tomography image of a normal ureter, showing anatomical layers of the ureter wall compared to histological image of the normal ureter. The layers of the ureter can clearly be recognized. * urothelium, # lamina propria, ^ muscularis propria
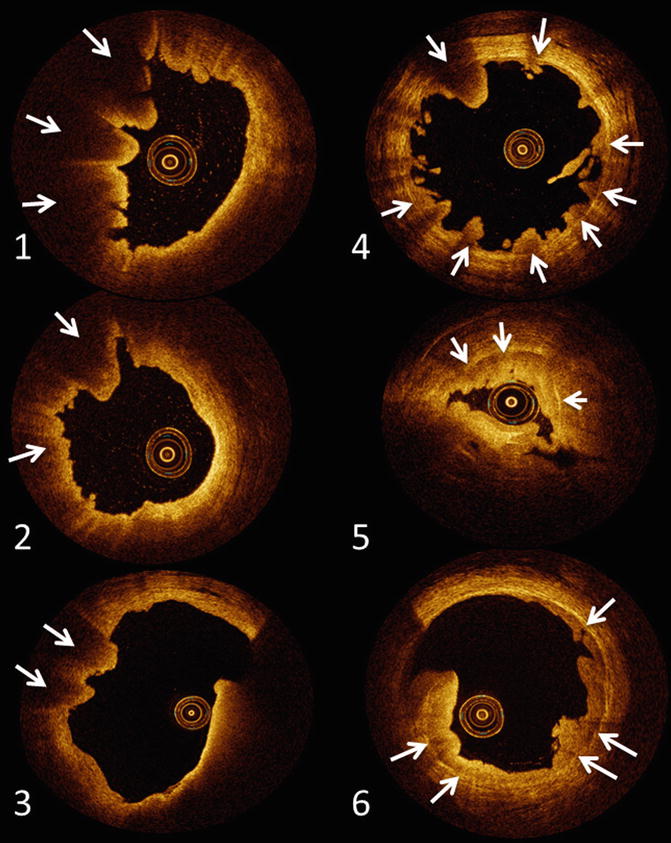
Fig. 12.5
Upper urinary tract urothelial carcinoma as seen with optical coherence tomography imaging. (1–3) Represent invasive UUT-UC. Invasive UUT-UC (≥T1) can be recognized as a loss of architecture of the anatomical layers underneath visible layers. (4–6) Demonstrate non-invasive UUT-UC. Underneath the visible tumour lesions the anatomical layering of the ureter wall can be clearly recognized
Several features of OCT are appealing for intraluminal diagnostics. First, flexible OCT probes are compatible with modern endoscopes and easy to apply in the ureter, pyelum and calyces, enabling OCT imaging at all sites within the urinary tract and not interfering with deflecting properties and rinsing during the procedure. Second, OCT measurement duration per patient adds only maximal five minutes operating time during URS, as the probe is easy to use and a single measurement takes 5.4 s per region under investigation. Third, OCT does not require a conducting medium or direct contact, which makes it easily applicable in the ureter. Finally, the OCT system is compact and portable, which results in an easy to use system in the operating theatre. In conclusion, the advantages of OCT are the non-invasive, real-time and high-resolution 3D imaging that allows visual staging and extraction of the optical attenuation coefficient that allows grading. However, current commercial available OCT systems are limited to image a lumen with a maximal diameter of 10 mm, compromising visualization of the pyelum and bladder as a whole. Furthermore, if tumour thickness transcends scattering-limited imaging depth in tissue (~2 mm), invasiveness cannot be assessed. A recent study quantified the learning curve and inter-observer variance of the μoct. It was concluded that routine μoct determination for tissue classification does not require extensive training and that OCT naïve people only require three trainings to acquire the same results as experienced OCT investigators. This increases the clinical potential of OCT [32].
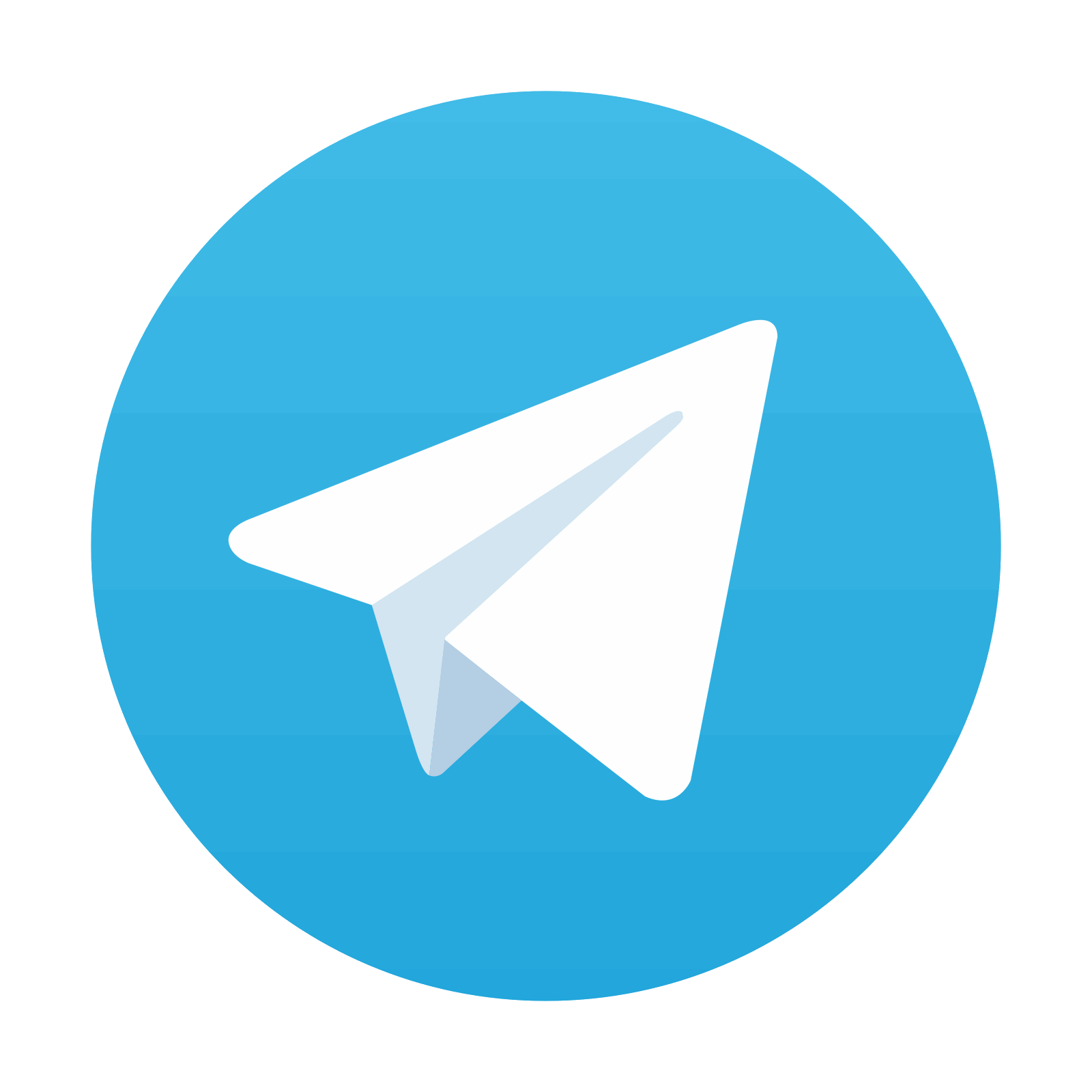
Stay updated, free articles. Join our Telegram channel

Full access? Get Clinical Tree
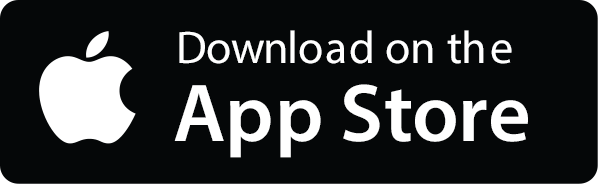
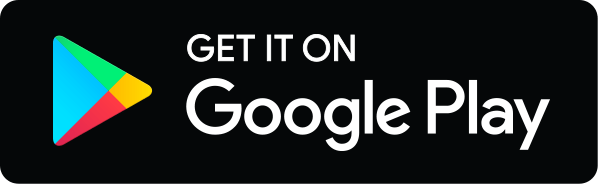