Ohm’s law simply states that the current induced in a conductor increases as the applied driving force (V) is increased or as the opposition to charge movement (R) is decreased.
Alternatively, Ohm’s law may be expressed in terms of conductance (G) rather than resistance:


4.4.5 Capacitance and Impedance
In order to understand current in biological tissues, two other electrical concepts must also be introduced. Capacitance is the property of a system of conductors and insulators that allows the system to store charge. Currents produced in biological tissues are influenced not only by tissue resistance but also by tissue capacitance.
The capacitance is expressed in farads (F); 1 F is the magnitude of capacitance as 1 C of charge is stored when 1 V of potential difference is applied.
The term impedance (Z) describes the opposition to alternating currents, analogous to the way resistance describes the opposition to direct currents, that a system presents when a voltage is applied. Alternating current could be defined as a continuous or uninterrupted bidirectional flow of charged particles.
Impedance takes into account both the capacitive and resistive opposition to the movement of charged particles. When dealing with clinical electrical stimulation, it is more appropriate to express the opposition to current in terms of impedance, because human tissues are better modeled as complex resistor and capacitor networks.
Because impedance depends on the capacitive nature of biological tissues, its magnitude depends on the frequency of applied stimulation. In general, the higher the frequency of stimulation, the lower the impedance of tissues. The standard unit of impedance is the ohm.
4.5 Intuitive Approach to Electric Parameters
The concepts of voltage, current, and impedance are easily related to more intuitive parameters, allowing basic understanding to grow from a conceptual basis as well as from quantitative analysis.
Mechanical systems, fluid flow, heat transfer, and electric systems are all described by the same differential equations. To understand any one of these processes is equivalent to understanding them all. The only difference is the parameters. Substituting voltage for pressure and electric current for fluid flow allows an electric engineer to work in fluid mechanics [12].
Voltage is a measure of electric potential energy just as height is a measure of gravitational potential energy in the approximately constant gravitational field near the earth’s surface. The gravitational potential energy of an apple is realized when it drops from the tree. The apple’s velocity, when it hits your head, depends on the difference between the original height of the apple and the height of your head. Both height and voltage are measured as a difference between two locations, rather than as absolute numbers.
Current is a measure of flow. A river current corresponds to the volume of water that flows in some amount of time (e.g., liters per second). The current is determined by the steepness of the river grade (voltage) and the friction of the water and riverbed (resistance). A wide river flowing from a steep mountain passes huge amounts of water, just as a small resistor and a large voltage results in a huge electric current.
The electrons in a conductor that are free to move are also analogous to water in a long, straight, horizontally positioned pipe. The water has the capability of flowing through the pipe, but it will only dribble out the ends as long as the pipe is exactly level. Only when one end of the pipe is raised above the other end will a flow occur.
For direct currents (DCs), impedance is the same as resistance and simply corresponds to friction in a mechanical system. When alternating currents (ACs) are used, however, some of the energy from one cycle can be stored for use in later cycles. This concept of energy storage forms the basis of capacitance and impedance. A classic example of capacitance/impedance could be considered the Newton’s cradle. If one ball is pulled away and is let to fall, it strikes the first ball in the series and comes to nearly a dead stop. The ball on the opposite side acquires most of the velocity and almost instantly swings in an arc almost as high as the release height of the last ball. This shows that the final ball receives most of the energy and momentum that was in the first ball, with the results of bouncing forever. In this system, friction is virtually eliminated. This is impedance without resistance. Energy is simply shifted back and forth between the first and the last ball. If friction is introduced, then energy is dissipated as heat and, over time, the bouncing subsides.
In complete analogy to the electrical impedance could be defined the acoustic impedance, a complex number which describes how a medium absorbs sound by relating the amplitude and phase of an applied sound pressure to the amplitude and phase of the resulting sound flux.
4.6 Stimulation Parameters
4.6.1 Frequency
Frequency is the number of occurrences of a repeating event per unit time. The standard international unit for frequency is the hertz (Hz), named after the German physicist Heinrich Hertz; 1 Hz means that an event repeats once per second.
Electrical frequency refers to the pulses produced per second during stimulation (e.g., 40 Hz = 40 pulses per second).
Nerve activation in applications for functional electrical stimulation is usually restricted to frequencies below 50 Hz. It has been suggested that frequencies above 50 Hz may predispose to nerve damage with continuous stimulation [13]. Frequencies above 100 Hz have been defined as high frequency and such frequencies have often been reported to result in the failure of evoked neural responses [14, 15]. The blocking effects of high-frequency alternating current (HFAC) waveforms have been variously reported since 1939 [16]. Bowman and McNeal evaluated the effect of voltage-controlled biphasic rectangular pulses between 100 Hz and 10 kHz and achieved a nerve conduction block above 4 kHz [17]. Stimuli at 300 Hz applied to the pudendal nerves have been reported to show a pressure reduction of the urethral sphincter by 30–45 % [18]. Li et al. have used 200–300 Hz stimuli to cause sphincter fatigue prior to evoked voiding and suggested that the optimal parameters to induce sphincter fatigue were 3 V, 100–500 Hz, and 100 microseconds for 15–20 s [19, 20]. Applied frequencies of 600 Hz have been claimed to produce a conduction-type block [21]. Tai et al. have shown that in the pudendal nerve, isolated from the spinal cord, evoked responses could be blocked with HFAC above 7 kHz [22].
The changes in anal pressure could be obtained without fatigue at stimulation frequencies of 10–20 Hz [23].
The effect of sacral nerve stimulation on bladder function has been suggested to be dependent on stimulation frequency, with bladder excitation dominating at frequencies of 2–5 Hz and bladder inhibition dominating at 10 Hz [24].
Low-frequency TENS stimulation induces the selective release of endorphins in the central nervous system [25].
4.6.2 Pulse Width
Electrical stimulation devices deliver pulses in waveform patterns that are often represented by geometric shapes such as square, peaked, or sine wave. These shapes characterize electrical current that rises above a zero baseline for the extent of the stimulation paradigm (uniphasic; e.g., direct current) or current that alternates above and below the baseline (biphasic or alternating current) [26]. The time span of a single pulse is known as the pulse width or pulse duration, so the pulse width is how wide each pulse is. It is measured in microseconds. Generally speaking, the higher the pulse width, the more “aggressive” the stimulation feels.
Dudding and colleagues, which examined changes in rectal compliance following acute changes in stimulation parameters, observed an increase in rectal compliance when the pulse width was decreased to 90 μs or the frequency increased to 31 Hz, from the conventional SNS settings of 14 Hz and 210 μs [27].
Dinning and colleagues [28] found no differences between stimulation with a pulse width of 300 and 400 μs about colonic motor responses.
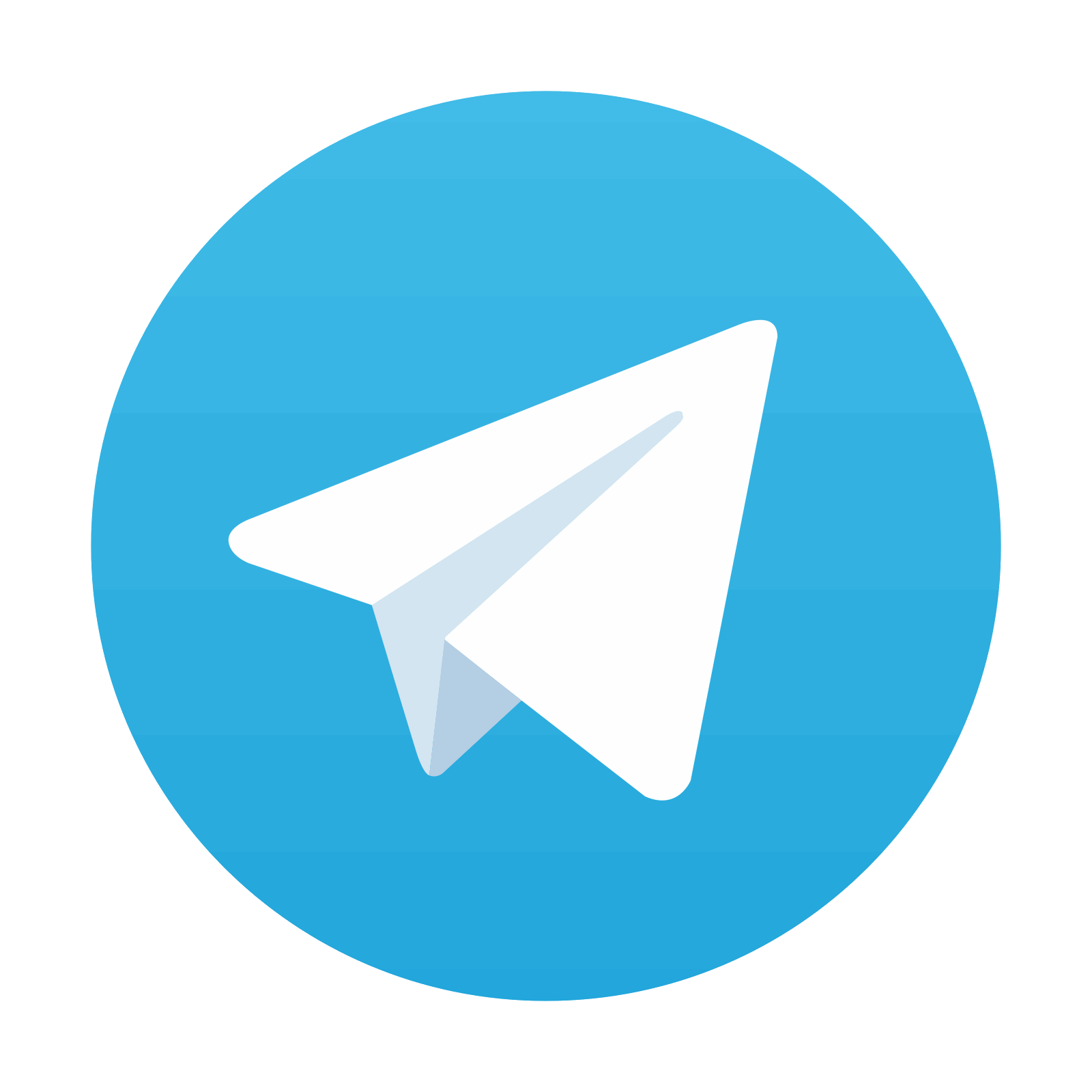
Stay updated, free articles. Join our Telegram channel

Full access? Get Clinical Tree
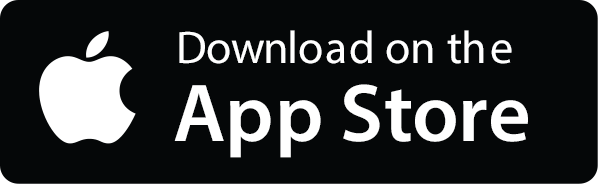
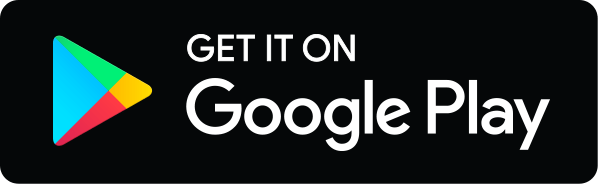