Introduction
Whereas calcineurin inhibitors (cyclosporine and tacrolimus) block early signaling events and cytokine production, both azathioprine and mycophenolates, often termed antiproliferatives, exert effects downstream in the cell cycle, interfering with cytokine-dependent signals and lymphocyte proliferation. Azathioprine, usually in combination with corticosteroids, was the backbone of immunosuppression in renal transplantation from the early 1960s to the early 1980s, after which cyclosporine (CsA) was added to the mix. “Triple” therapy, with CsA, azathioprine, and steroids then became standard in most centers through the mid-1990s. Mycophenolate mofetil (MMF) was developed in the early 1990s as an alternative to azathioprine, supplanting it as standard therapy (in combination with calcineurin inhibitors and steroids) by the end of the decade in most developed countries. At the time of this writing, MMF is the most widely prescribed transplant immunosuppressant in the world, although some would maintain its superiority compared with azathioprine is modest. In addition, because azathioprine is less expensive, it will continue to have a role in transplantation, in particular, in developing countries where cost is a greater determinant of immunosuppressive protocols. Thus both remain relevant to clinical practice in transplantation.
History
Azathioprine
6-Mercaptopurine was developed by Elion and Hitchings at Burroughs Wellcome as an anticancer agent in the 1950s. Subsequently, 6-mercaptopurine was shown to be immunosuppressive by Schwartz and Dameshek ; it attenuated the humoral response to a foreign protein and prolonged survival of skin allografts in rabbits, termed drug-induced immunologic tolerance. This work was noted by Calne in the UK and Hume in the US; independently, these investigators found that 6-mercaptopurine delayed or prevented rejection of renal allografts in dogs. In Calne’s laboratory, although only two dogs survived the kidney transplant operation, at death a little more than a month later, there was no histologic evidence of rejection, a unique finding at the time. Almost simultaneously, similar results in a much larger series of canine transplants emerged from Hume’s unit. Soon thereafter, Elion and colleagues produced azathioprine, an imidazolyl derivative of 6-mercaptopurine that appeared somewhat less toxic than 6-mercaptopurine. Azathioprine was first used in humans in Boston at the Peter Bent Brigham Hospital in 1961 and then subsequently utilized (at doses of 2.5–3 mg/kg/day) in a rapidly increasing number of kidney transplant units globally.
In those early days, rejection seemed inevitable, and almost every patient experienced at least one episode. At first, corticosteroids were used to treat rejection in patients on azathioprine; later they were added to azathioprine-based prophylaxis by Starzl at the time of transplantation. In this so-called azathioprine era, arbitrarily large doses of steroids were administered from the time of transplantation, with a gradual reduction over 6 to 12 months to maintenance levels. These high doses of steroids may have been primarily responsible for significant morbidity (discussed in Chapter 16 ); only in the 1970s, after a series of randomized trials and clinical observations documenting the efficacy of lower doses in preventing rejection, was a major reduction in steroid-related complications noted (see Chapter 16 ). By the late 1970s, azathioprine and low-dose steroids, sometimes used together with an antilymphocyte serum or globulin for induction (particularly in North America), had become standard immunosuppressive therapy.
After CsA emerged in the early 1980s, azathioprine was deemed obsolete. However, it quickly reemerged, utilizing lower doses (1.5–2 mg/kg/day) as adjunct therapy to enable administration of lower, presumably less-toxic, doses of CsA. For more than a decade, triple therapy with CsA (4–8 mg/kg/day), azathioprine, and low-dose corticosteroids was considered standard immunosuppressive therapy for kidney recipients in most units in the developed world. During this era, acute rejection rates fell from 80% or greater to around 50%, with some improvement in graft survival and patient survival, and substantially less morbidity than in preceding decades. It was the search for even better immunosuppressants in the late 1980s that led to the introduction of mycophenolates.
Mycophenolates (Mycophenolic Acid, Mpa)
MPA is a fermentation product of Penicillium brevicompactum and related fungi. It was first isolated by Gosio in 1896, named in 1913 by Alsberg and Black, and subsequently found to have weak antibacterial and antifungal activity. Raistrick and colleagues published the structure of MPA in 1952. In the 1960s, it was found to have a strong antimitotic effect in mammalian cells and was regarded as a potential antitumor agent. Subsequent work by Franklin and Cook documented its major mechanism of action (inhibition of the do novo pathway of purine synthesis). At about the same time, a Japanese group documented MPA’s immunosuppressive properties, which were initially viewed as an impediment to clinical utility.
As noted earlier, discovery and development of CsA changed the landscape for transplant immunosuppression. In the search for new agents targeting other pathways, Allison and Eugui, among others, working first in the UK and subsequently at Syntex Pharmaceuticals, began examining in vitro the effect of MPA on lymphocyte function, documenting inhibition of cytotoxic T cell generation, antibody formation, lymphocyte adhesion, and the mixed lymphocyte reaction. Scientists at Syntex, via morpholinoethyl esterification of MPA, produced a prodrug (RS61443, mycophenolate mofetil, or MMF) with substantially improved oral bioavailability in humans and other mammals. Initial animal studies demonstrated the agent to be difficult to administer to dogs and primates, with substantial gastrointestinal toxicity, especially at higher doses (>20 mg/kg). Optimal kidney graft outcomes in dogs occurred when RS61443 was combined with low-dose CsA and prednisone. MMF monotherapy, even at substantially higher doses, demonstrated only limited efficacy. The initial human experience was in rheumatoid arthritis, where a dose of 2 g/day was associated with “significant clinical improvement” among refractory patients. Side effects were minimal and primarily of gastrointestinal origin (nausea, vomiting, abdominal pain, diarrhea). No other significant toxicities (including bone marrow suppression) were noted.
In 1988, it was elected to proceed with clinical trials in kidney transplantation. The initial study was a phase I/II trial involving 48 kidney recipients at two centers. All the patients received polyclonal antibody induction, CsA, and prednisone. Eight dosing groups of six subjects each received MMF in daily doses ranging from 100 to 3500 mg. The greatest efficacy in preventing acute rejection with an acceptable adverse event profile was seen in the 2000 and 3000 mg dosing groups, providing the basis for the design of three large registration trials.
The phase III MMF trials, commencing globally in 1992, were the first of their kind: rigorously conducted studies involving large numbers of patients in solid-organ transplantation. Each study included approximately 500 subjects assigned to one of three treatment arms. Two of the groups in each study received MMF, either 2 or 3 g/day, in combination with CsA and corticosteroids. In the US trial, all the patients underwent polyclonal antibody induction, with the comparator group receiving azathioprine instead of MMF. In the Tricontinental trial, treatment arms were identical, with an azathioprine control group, but without antibody induction. In the European trial, there was no antibody induction, and comparator patients received placebo instead of azathioprine. Findings of all three trials were remarkably similar: a 50% reduction in rates of acute rejection with MMF (to less than 20% overall) within the first 6 months after transplantation, with identical graft and patient survival among treatment arms at 1 and 3 years ( Fig. 15.1 ) For most patients, the 2-g dose appeared to offer optimal efficacy with fewer adverse effects. Based on these findings, on May 3, 1995, the US Food and Drug Administration (FDA) approved MMF (CellCept) 2 to 3 g/day for use in combination with CsA and corticosteroids, with global availability soon to follow. A starting dose of 2 g/day became widely accepted in adults, and, by 2002, MMF was the most widely prescribed immunosuppressant in the US.

In ensuing years, there have been at least three other landmark developments affecting the use of mycophenolates in transplantation. First, to address the gastrointestinal adverse events that plague some patients, Novartis developed an enteric-coated formulation, mycophenolate sodium (EC-MPS, Myfortic). Designed to delay MPA release and absorption in the gut (with the assumption that gastric and early small-bowel exposure were major determinants of gastrointestinal toxicity), clinical trials documented EC-MPS efficacy equivalent to MMF, with trends to fewer adverse gastrointestinal events. EC-MPS became available in 2004. Second, in 2008, patent protection for the innovator formulation of MMF (CellCept) began expiring in the US and globally. The clinical effect of the availability of multiple MMF preparations on patients and the marketplace remains controversial. Finally, in 2009, Tacrolimus/MMF became a FDA-approved combination in kidney transplantation. Previously, use of MMF or EC-MPS with tacrolimus, the most common immunosuppressant combination in the world, had been “off-label,” limiting its use as a comparator protocol in drug development. This label change altered the landscape for new drug development and provided guidance for MPA dosing in tacrolimus-based protocols.
Mechanism of Action
Although both azathioprine and mycophenolate are considered antiproliferative agents, their effects result from differing mechanisms of action. Azathioprine is a thiopurine, an imidazolyl derivative of 6-mercaptopurine. It is transformed to 6-mercaptopurine in the liver, in turn, converted to thioinosinic acid and 6-thioguanine. These latter metabolites are incorporated into DNA and RNA, inhibiting lymphocyte proliferation by suppressing de novo purine synthesis.
Given the documented immunosuppressive effect of azathioprine, a relatively nonspecific inhibitor of nucleotide synthesis, MPA use in transplantation was based on the observation that inherited deletions in nucleic acid synthesis resulted in immunodeficiencies: children lacking adenosine deaminase show combined T cell and B cell deficits. In contrast, subjects with absence of hypoxanthine-guanine phosphoribosyl transferase display essentially normal immune function, indicating the purine salvage pathway as relatively unimportant in lymphocytes ( Fig. 15.2 ). MPA acts as a reversible, noncompetitive inhibitor of inosine 5′-monophosphate dehydrogenase (IMPDH), the rate-limiting enzyme in the de novo synthesis of guanine nucleotides. This effect arrests new DNA synthesis in proliferating cells at the G 1 /S interface, with guanosine triphosphate (GTP) decreasing to 10% of levels measured in unstimulated T cells. Addition of guanosine or deoxyguanosine reverses the inhibition, confirming the IMPDH target. T and B lymphocytes are highly dependent on this pathway for proliferation in response to stimulation. There are two isoforms of IMPDH, with type I expressed in many cell lines, but type II the predominant isoform in activated T and B lymphocytes; IMPDH type II is four times more sensitive to MPA. These two factors impart some degree of lymphocyte-specific selectivity to the antiproliferative activity of MPA. Although monocyte and dendritic cell replication may also be affected by MPA, there is relatively little effect on neutrophils.

The action of MPA at the G 1 /S interface is also selective. Neither the production of interleukin-2 nor the expression of its receptor is affected, showing a lack of influence on signal 1 of lymphocyte activation. The primary antiproliferative effect also retards induction of cytotoxic T cells. Similarly, MPA mitigates primary and ongoing B cell responses, presumably as a result of blockade of cell division, with subjects receiving MMF in the US pivotal trial demonstrating far less production of xenoantibody toward equine antithymocyte globulin (ATG) than those on azathioprine. MPA treatment also blunts the synthesis of natural xenoantibodies after plasma exchange and splenectomy in rats and inhibits the humoral response to influenza vaccine in humans.
Another distinct mechanism of MPA activity may relate to the requirement for GTP to activate fucose and mannose transfer and glycoprotein synthesis, thus diminishing expression of adhesion molecules. When GTP is depleted as a consequence of MPA, adhesion of lymphocytes and monocytes to activated endothelial cells is decreased in a dose-dependent fashion.
Cell types other than lymphocytes may also be sensitive to MPA inhibition, with some models indicating vascular smooth muscle cells, mesangial cells, and myofibroblasts to display dampened proliferation. In a subhuman primate model of orthotopic allograft vasculopathy, intravascular ultrasound documented dose-dependent inhibition of the progression of intimal volume changes. The efficacy of MMF was confirmed using aortic allograft in another subhuman primate model and in a rodent chronic rejection system. The effects were potentiated when MMF was administered with sirolimus, a combination that also reduced transforming growth factor-β 1 and its effects on extracellular matrix synthesis and degradation. There may also be a more direct antifibrotic effect of MPA mediated by upregulation of neutral endopeptidase in the kidney.
Clinical Pharmacology and Blood Monitoring
Azathioprine
Dosing, Metabolism, and Monitoring
Azathioprine is given as a single daily dose. If used with steroids alone, a suitable starting dose is 2.5 mg/kg/day, with careful monitoring of leukocyte counts. The dose of azathioprine may be reduced somewhat over time, particularly in the face of evidence of marrow suppression. Analysis of data from the Collaborative Transplant Study suggested that, for patients receiving azathioprine and steroids only, long-term doses greater than 1.5 mg/kg/day were associated with superior graft survival. When azathioprine is administered with a calcineurin inhibitor (CNI) and steroids (triple therapy), lower doses are appropriate. A fairly standard dose of azathioprine in a triple protocol is 1.5 mg/kg, 100 mg/day. At this level, hematologic toxicity is uncommon.
Xanthine oxidase has an important role in the catabolism of 6-mercaptopurine, and, therefore, azathioprine. Concomitant use of xanthine oxidase inhibitors (e.g., allopurinol) requires significant azathioprine dose reduction to avoid excessive toxicities, particularly in the bone marrow. Although the metabolites are excreted in the urine, these are inactive, and no reduction in dosage is required in acute kidney failure.
Regular monitoring of hematologic parameters is an important aspect of azathioprine therapy; common practice is to reduce the dose when the leukocyte count decreases to less than 3 × 10 9 /L. As already mentioned, if xanthine oxidase inhibitors are used, the azathioprine dose should be reduced by at least 25% or consideration given to conversion to MPA. Blood levels of azathioprine or its metabolites are not routinely monitored in clinical practice. It has been noted, however, that the development of leukopenia can also result from viral infection, leading to the suggestion that erythrocyte 6-thioguanine nucleotide levels may be a better indicator of azathioprine activity in transplant patients.
A number of genetic variations in the thiopurine S-methyltransferase (TMPT) gene have been identified, which have been related to azathioprine-induced toxicities. Polymorphisms in the TMPT enzyme, which catalyzes the S-methylation of 6-mercaptopurine and azathioprine, may be associated with an increased likelihood of myelotoxicity and leukopenia and require dose modification. Genotyping for this polymorphism before commencing azathioprine might allow the appropriate azathioprine dose for an individual to be determined, however, the cost/benefit ratio of this approach remains controversial.
Side Effects
The major complication of azathioprine therapy is bone marrow suppression, most commonly manifest as leukopenia, although, in more severe settings, anemia and thrombocytopenia may also occur. Megaloblastic anemia has been described in association with the use of azathioprine. Although hepatotoxicity has been attributed to azathioprine for many years; it is probably rarer than thought, with many cases likely reflective of unrecognized viral hepatitis. Hair loss is an uncommon complication. Increased risk of nonmelanoma skin cancers and other malignancies in transplant recipients has been attributed to azathioprine. Evolving experience, with both skin and colon cancer, does indeed indicate azathioprine-treated patients to be at greater risk, with a recent meta-analysis indicating increased skin cancers like squamous cell, but not basal cell carcinoma. Mechanistically, Hofbauer and colleagues have shown that mercaptopurine metabolites intercalated into DNA of skin increase sensitivity to ultraviolet irradiation, providing a plausible mechanistic explanation for the clinical observations.
Mycophenolates
Dosing
In adults with kidney transplants, MMF is most commonly administered orally at a dose of 1 g twice daily. In pediatric patients, the recommended dose of MMF oral suspension is 600 mg/m 2 at 12-hour intervals up to a maximum of 2 g daily. Intravenous MMF is administered at the same dose and frequency as the oral preparation, infused over 2 hours. EC-MPS is administered orally at a dose of 720 mg twice daily in adults. This suggested dose of EC-MPS in a stable pediatric patient is 400 mg/m 2 twice daily, but its use is not recommended in patients less than 5 years old.
Absorption
Orally delivered MMF is rapidly and almost completely absorbed in the stomach and upper intestine and then efficiently hydrolyzed to MPA ( Fig. 15.3 ). Drug absorption, as assessed by area under the curve (AUC), is not significantly altered by coadministered food, although the maximal concentration (C max ) is 40% lower in simultaneously fed renal transplant recipients. The time to maximal concentration (T max ) of less than 1 hour is independent of hepatic or renal function. The T max is slightly delayed, however, in the period immediately after transplantation (1.31 ± 0.76 hours) and in diabetic patients (1.59 ± 0.67 hours). In contrast, by 3 months it is 0.90 ± 0.24 hours. Metabolism of MMF to MPA yields 94% bioavailability. Over a range of 100 to 3000 mg/day, the MPA area under the concentration-time curve for 24 hours (AUC 0–24 ) is proportionate to dose in healthy subjects, although a recent study of MMF kinetics in kidney transplant recipients revealed a nonlinear relationship, with reduced bioavailability as doses increase from 250 mg to 2000 mg. The magnitude of the nonlinearity was greater (176%–76%) in combination with tacrolimus than with CsA (123%–90%). A recent multicenter crossover study comparing pharmacokinetics of two MMF preparations (Teva and Roche) in 43 stable kidney recipients found them to be comparable in all parameters studied.

EC-MPS does not release MPA under acidic conditions (pH <5) as in the stomach but is highly soluble in a neutral-pH environment like the intestine. Consistent with this property is a T max of 1.5–2.75 hours, substantially delayed compared with MMF. Gastrointestinal absorption is 93%, and absolute bioavailability of MPA after administration of EC-MPS is 72%. There is a substantial effect of food on MPA absorption with EC-MPS, although overall AUC is not affected; to avoid variability, it should always be taken on an empty stomach. Like MMF, EC-MPS pharmacokinetics are dose-proportional over its administration range, and its profile regarding metabolism and clearance (see later) is similar as well. An EC-MPS dose of 720 mg most closely approximates the MPA exposure of 1000 mg of MMF.
Metabolism and Clearance
MPA is rapidly metabolized to an inactive glucuronide (MPAG) via one or more isoforms of the UGT1 gene family of uridine diphosphate–glucuronosyl transferases in the gastrointestinal tract, liver, and possibly kidney. Two minor metabolites also are formed: the acyl glucuronide and the phenolic glucoside (see Fig. 15.3 )
The apparent elimination half-life of MPA in healthy volunteers is 17.9 hours – a clearance of 11.6 L/h. At 8 to 12 hours after oral drug administration, 37% of patients (range 10%–61%) display a secondary peak in plasma MPA concentrations representing enterohepatic recirculation. The additional peak results from excreted MPAG in bile undergoing deglucuronidation by intestinal bacteria with subsequent reabsorption of MPA, and may account for up to 40% to 60% of the total dose interval MPA AUC.
MPAG is the major urinary excretion product (93% of the radioactive parent compound); urinary excretion of MPA is negligible (1%). Fecal excretion accounts for 6%. Neither hemodialysis nor peritoneal dialysis significantly affects MPA plasma concentrations, although in multiple-dose studies either dialysis method may remove some MPAG. With renal dysfunction, there is a moderate increase in plasma MPA and a marked accumulation of MPAG. MPA binds tightly and extensively (97%), but reversibly, to serum albumin, decreasing its ability to inhibit IMPDH. The free fraction, constituting 1% to 3% of the total amount in the blood of stable patients, is cleared by biliary and renal routes.
Hypoalbuminemia is associated with increased free MPA and greater MPA clearance. Renal allograft dysfunction also increases the MPA-free fraction because it decreases the binding of acidic drugs; in contrast, hepatic oxidative impairment produces no effect. Estimates of free MPA in ultrafiltrate samples have not been shown to offer any advantage over measurements of total MPA to predict therapeutic versus adverse reactions, except in the presence of impaired graft function.
Assays and Blood Monitoring
The MPA parent compound is readily measured in plasma by high-performance liquid chromatography. In contrast, the widely available automated enzyme multiplier immunoassay technique (EMIT) yields 15% to 20% higher results because its antibody reagent cross-reacts with the acyl-MPAG metabolite (see Fig. 15.3 ), which may contribute to both immunosuppressive and toxic effects. Because of these properties, some have suggested the EMIT assay may be preferable and is the most widely utilized.
The gold-standard pharmacokinetic measure of MPA exposure is a frequent sampling of blood over a 12-hour period, with the calculation of the AUC concentration-time exposure. MPA AUC can be calculated from either full (8 or more samples over 12 hours) or limited (2–5 samples over 4 hours) sampling strategies. Although the evidence is conflicting regarding utility, 12-hour predose (C 0 ) MPA levels are convenient to obtain and have been utilized effectively in some studies. Correlation ( r 2 ) of the predose level with AUC of MMF ranges from 0.32 to 0.68 and may differ by concomitant CNI usage ( Fig. 15.4 ). For EC-MPS, variability in absorption means significantly poorer correlation between predose MPA level and AUC ( r 2 = 0.02). Long-term, intrapatient variability of both AUC and trough levels is relatively low.
After the first few weeks after transplantation, dose-normalized MPA exposure increases by 30% to 50%, perhaps reflecting a decline in drug clearance, MPA saturation of tissues, or other factors. MPA exposure/dose relationships may also differ in settings other than kidney transplantation (e.g., liver and small-bowel transplantation, bone marrow transplantation). Typically, younger children require higher MMF doses per body mass index than older children or adults to achieve comparable MPA exposure.
Considerable interpatient pharmacokinetic variability of MMF has been documented to be due to differences in hepatic/renal function, concurrent drug administration, and the presence of diarrhea, but not to ethnicity or gender. Pharmacodynamic monitoring of patients receiving MPA therapy has been studied. Peripheral blood lymphocytes (PBL) from patients on MMF demonstrate reduced response to stimulation assays and diminished antibody production. However, so many other factors affect the proliferative activity of PBL in this setting that the clinical utility of these assays is modest. IMPDH assays are technically demanding and difficult to reproduce; in addition, the whole-blood matrix may not reflect the effect in activated lymphocytes, which are the cells of interest. Estimates of IMPDH activity in isolated peripheral blood mononuclear cells indicate considerable interindividual variability. The time course of IMPDH inhibition, as measured by the production of xanthine monophosphate by isolated mononuclear cells, parallels the MPA plasma concentration. Patients with lower IMPDH levels before transplantation, indicative of genetic susceptibility to the drug, more frequently underwent dosage reductions within 6 months; pretransplant IMPDH activity has been suggested as a guide to MPA dosing in pediatric patients. It is unclear, however, whether levels of fluctuations in IMPDH activity can be of utility in predicting either efficacy or toxicity of MMF in clinical transplantation.
Drug–Drug Interactions
In general, important drug–drug interactions with mycophenolates are rare. Cholestyramine may reduce MPA absorption. Antibiotic therapy disrupting the gastrointestinal flora may interfere with deglucuronidation and enterohepatic recirculation, decreasing drug levels. Because acyclovir and ganciclovir compete with MPAG for tubular secretion, they may increase drug concentration slightly, particularly among patients experiencing renal impairment. Coadministration of proton pump inhibitors has been reported to reduce exposure to MPA by 25% to 35%, but the clinical effect of this interaction is uncertain.
Clearly, however, the most significant drug–drug interaction is with CsA: its importance is magnified because most dosing regimens of MMF and EC-MPS were established utilizing this combination. When either mycophenolate preparation is administered with CsA, subjects display lower MPA concentrations than those not receiving CsA or in whom the drug was discontinued. This effect is likely the result of reduced enterohepatic recirculation. In contrast, coadministration with tacrolimus does not alter MPA exposure compared with placebo, and MPA exposure in combination with sirolimus is likewise higher than observed with CsA. There appears to be no drug–drug interaction between belatacept and MMF. Similarly, the experimental Janus kinase inhibitor tofacitinib does not affect MPA pharmacokinetics. This property resulted in 37% greater MPA exposure in tofacitinib-treated (versus CsA) subjects in a phase IIb trial, with a profound influence on the outcomes in kidney transplant recipients. Finally, the corticosteroid complement of most regimens increases MPAG slightly by inducing hepatic glucuronosyl transferase.
Clinical Toxicities of Mycophenolates
The initial clinical trials of MMF indicated the absence of nephrotoxicity, neurotoxicity, or hepatotoxicity. Myelotoxicity and gastrointestinal side effects were reportedly “modest.” In general, adverse events, including invasive cytomegalovirus (CMV) infections, were more common in patients receiving 3 g (vs. 2 g) of MMF daily, suggesting a dose-dependent relationship. In the pivotal trials, adverse events accounted for study withdrawal in 15% of subjects in the MMF 3 g/day dose, 9% from MMF 2 g/day dose, and 5% from the comparator cohorts. Early on, these side effects were thought to be related to the MMF dose rather than to the plasma concentration of parent compound or its metabolites, and indeed adverse events often respond to MMF dose reduction. However, whereas findings have been somewhat inconsistent, more recent evidence has correlated gastrointestinal and hematologic adverse events to MPA exposure as well as dose. The frequency and severity of MMF-related adverse events are also influenced by the other immunosuppressants with which it is combined. The common clinical practice has been to reduce MMF dosing in response to adverse events; there is some evidence, however, that dose reduction can be associated with increased risk of rejection and graft failure, and should be approached cautiously.
Gastrointestinal Adverse Reactions
One or more gastrointestinal symptoms from a constellation that includes diarrhea, indigestion, bloating, nausea, vomiting, and abdominal pain, are the most commonly reported adverse effects of MMF therapy. In the European trial, the overall incidence of any gastrointestinal complaint was 53% and 46% for the MMF 3-g and 2-g doses, respectively, versus 42% in the placebo comparator arm. These adverse effects often improve with MMF dose reduction, or, in some cases, with the maintenance of the same total daily dose administered more frequently in smaller increments (e.g., 500 mg four times daily rather than 1000 mg twice daily).
The most common and troublesome gastrointestinal adverse event is diarrhea, experienced by up to 35% of subjects in the pivotal trials using CsA, almost twice the frequency as in the comparator arms. Diarrhea occurs even more often, and with greater severity, when MMF is used in combination with tacrolimus (45% vs. 25% with CsA in one study). Because diarrhea also increases tacrolimus absorption and blood levels, its clinical consequence may be greater with this combination as well. In a registry analysis, Bunnapradist and colleagues found significantly increased risk of diarrhea (hazard ratio [HR] 1.37) associated with the tacrolimus/MMF combination, which, in turn, was linked to increased risk of graft failure (HR 2.13) and patient death (HR 2.04). After renal transplantation, however, diarrhea can be due to a variety of causes other than immunosuppressant therapy, including preexisting diabetic or uremic conditions, intercurrent infectious diseases, and concurrent antibiotic treatment. Maes and colleagues reported 60% of 26 cases of diarrhea were due to an infectious origin: CMV, Campylobacter, bacterial overgrowth, or, in another report, microsporidiosis. In the other 40%, erosive enterocolitis was attributed to MMF, characterized by faster colonic transit, crypt distortion, and focal inflammation, thought to represent a toxic action of the acyl-MPAG metabolite on absorptive cells, leading to a predominance of goblet cells. In the presence of persistent diarrhea, colonic biopsy specimens have shown apoptosis of intestinal gland epithelial cells and atrophy of the intestinal villi, which has been documented to disappear a few months after MMF withdrawal.
EC-MPS was developed specifically to mitigate the gastrointestinal toxicities associated with MMF by delaying absorption in the gastrointestinal tract with the goal of reducing peak levels and delivering the active drug to more distal portions of the bowel. Compared with MMF, EC-MPS showed equivalent efficacy and overall equivalent MPA drug exposure in kidney transplant patients, but very similar gastrointestinal adverse events when administered in a randomized fashion (with CsA and corticosteroids) to either de novo or stable recipients. Some conversion patients, however, showed marked improvement in EC-MPS, and, in another study of gastrointestinal intolerance of MMF, fewer dose changes of EC-MPS were required, with an apparently reduced symptom burden, better functioning, and improved health-related quality of life.
Myelosuppression
Dose-dependent myelosuppression has been observed with MMF. This was particularly notable in the European trial, as subjects receiving 3-g or 2-g doses of MMF had more anemia or leukopenia (26% and 24%, respectively) than placebo-treated controls (13%). However, in the other pivotal trials, hematopoietic adverse events may have occurred slightly less frequently with MMF than in azathioprine controls. As with gastrointestinal side effects, it should be remembered that among kidney recipients there may be multiple causes of anemia or leukopenia, including renal dysfunction and infection, as well as concomitant immunosuppressants and other drugs.
A prospective study involving 978 patients at seven North American transplant centers documented anemia (hemoglobin ≤10 g/dL) in 10% of MMF-treated subjects and an association with recipient age, use of antiviral prophylaxis, and posttransplant dialysis. In the Fixed Dose-Concentration Controlled (FDCC) trial in Europe, anemia was reported in 38% of subjects. Greater MMF dose and MPA predose (C 0 ) plasma concentrations have been correlated with decreased hemoglobin values among stable renal transplant recipients. Another study suggested an even better correlation of anemia with MPA metabolites, MPAG and acyl-MPAG than with MPA itself. Conversely, others have found no association. In a single-nucleotide polymorphism analysis of kidney recipients with MMF-related anemia, one study found a protective effect of the HUS1 allele, with interleukin-12A and CYP2C8A genes associated with an increased risk for anemia. Other investigators, however, while confirming an association with CYP2C8A (but not the other genotypes) noted that expression of the “at-risk” phenotype is so rare (0.5% of population) as not to explain the observed frequency of anemia (or leukopenia). In the current era, leukopenia (fewer than 3000 leukocyte/mm 3 ) is reported in 14% to 23% of kidney transplant recipients. A multivariable analysis (Cox regression) of Medicare-covered kidney recipients found a significant association between mycophenolate use and leukopenia (adjusted HR 1.21). Other documented risk factors for leukopenia included corticosteroid-free regimens, weight, previous kidney transplant, decreased donor, and CMV seronegativity with a CMV-positive donor (and lengthier antiviral prophylaxis). MMF-related leukopenia may be associated with markedly abnormal neutrophil morphology. As with anemia, some, but not all, studies have shown MPA plasma concentrations and particularly free MPA AUC 0–12h to be significantly related to severe infections and leukopenia. The leukopenia has been associated with stomatitis, particularly when combined with a sirolimus-based regimen.
Infections
In the pivotal trials of CsA with MMF, whereas viral infections (herpes simplex and zoster, and tissue-invasive CMV) were more common in MMF-treated recipients than controls, other opportunistic infections were not. More recently, several studies have indicated significantly fewer herpes viral infections (especially CMV) with mammalian target of rapamycin (mTOR) inhibitors than with mycophenolates. However, widespread use of antiviral prophylaxis is now the norm, and most centers now view CMV as a manageable problem.
Recognition of BK virus infection and nephropathy coincided with the rapid assimilation of mycophenolates and tacrolimus into clinical practice, and most attribute its rise to more potent immunosuppression associated with these combinations. Aggressive screening of BK viruria or viremia, with immunosuppressant dose reduction (focused on mycophenolates), has diminished the effect of BK nephropathy on risk of allograft failure. Progressive multifocal leukoencephalopathy (PML) is a rare but debilitating central nervous system manifestation of polyomavirus infection, and it has been associated with mycophenolate use. Registry analysis of PML in the US found all reported cases in kidney recipients to be taking MMF, although MMF use was so ubiquitous that no statistical association could be documented. Another analysis of reported cases of PML in the US found a strong association with not only mycophenolates, but also azathioprine, CsA, cyclophosphamide, efalizumab, rituximab, and tacrolimus, among others, indicating a potential role for interactions among components of combination therapy and overall intensity of immunosuppression.
Introduction of MMF therapy has been reported to produce a significant increase in hepatitis C virus viremia in some, but not all, patients receiving concomitant CsA. In contrast, MMF seems to show no significant effect on hepatitis B virus viremia despite in vitro studies that suggested that it inhibited viral replication. Recent analysis of outcomes in kidney recipients with hepatitis C indicated no adverse effect of mycophenolate therapy on long-term graft or patient survival.
Neoplastic Diseases
Early experience with MMF was associated with a numerical increase in subjects with lymphoma compared with placebo or azathioprine control groups. However, large case-control studies now indicate little or no additional adverse effect of mycophenolates on the risk of lymphoma or other malignancy in patients receiving pharmacologic immunosuppression. Registry data also indicate malignancy risk associated with MMF use to be similar to other immunosuppressants. Despite an emerging consensus that mTOR inhibitors may be associated with reduced malignancy risk, much of the purported benefit is in comparison to CNIs rather than mycophenolates.
Pregnancy and Reproduction
MMF demonstrated teratogenic effects at subclinical doses in animal studies, which generated caution regarding its use in both males and females of reproductive age. Avoidance of mycophenolates in males fathering children is no longer recommended. However, fetal malformations have been reported in the offspring of female kidney recipients taking MMF. Mycophenolates are now recognized by the US FDA as category D agents in pregnancy (positive evidence of human fetal risk, but the benefits from use in pregnant women may be acceptable despite risk). In a female recipient with stable kidney allograft function at least 1 year posttransplant who desires pregnancy, common practice is to convert from mycophenolate to azathioprine at least 6 weeks before discontinuing contraception.
Early Clinical Trials of Mycophenolates in Kidney Transplantation
As mycophenolate emerged as a potential immunosuppressant in the late 1980s, azathioprine was the antiproliferative agent of choice, most commonly utilized with CsA and corticosteroids as triple therapy. A phase I/II dose-finding study of MMF in combination with polyclonal antibody induction, CsA, and corticosteroids in de novo kidney recipients indicated the therapeutic benefit of MMF at doses of 2 to 3 g/day. There was also suggested efficacy at these doses, demonstrated in the treatment settings of an ongoing or steroid-resistant acute renal rejection episode. Three blinded, randomized phase III trials were initiated in 1992. Patients in each trial were assigned to one of three treatment arms: a control group or one of two groups receiving MMF 2 g or 3 g daily, respectively. All patients also received CsA and prednisone. The primary end-point of each study was the prevention of acute rejection episodes during the first 6 months after transplantation, thought to be indicative of poorer 1-year and possibly long-term graft survival.
The European Mycophenolate Mofetil Study compared MMF with placebo with no antibody induction therapy. At 6 months, 46% of those receiving placebo had experienced biopsy-proven acute rejection (BPAR), versus only 17% (2 g) and 14% (3 g) of those randomized to MMF. The US Renal Transplant Study, which required polyclonal antibody induction for all patients and included an azathioprine control arm, yielded corresponding acute rejection rates of 38%, 20%, and 18%, respectively. The Tricontinental study, which included an azathioprine comparator but without antibody induction, demonstrated BPAR in 36%, 20%, and 15% of subjects, respectively. Each study also found significantly fewer histologic and clinically severe rejections among the MMF-treated cohorts. Thus each trial documented: (1) similar benefit of MMF compared with azathioprine (or placebo) in the prevention of early rejection episodes, (2) fewer withdrawals in MMF-treated subjects due to treatment failure, and (3) more adverse effects in the 3-g than the 2-g MMF groups.
Although none of the studies individually was powered to test efficacy in terms of graft survival, a planned pooled analysis of 12-month data from the three studies, with a total of 1493 randomized subjects, confirmed the benefit of MMF on acute rejection episodes: 20% and 17% in the two MMF arms versus 41% among controls, yielding a relative risk (RR) ratio of 0.46. There was significantly less steroid-resistant rejection with MMF, and renal function at 1 year was substantially better (despite identical CsA exposure) in the MMF-treated patients. Despite a trend indicating fewer graft losses over time with MMF, the combined incidence of graft loss and death was 10%, 11%, and 12%, respectively, at 1 year ( P = not significant). Longer-term follow-up failed to suggest a benefit of MMF versus azathioprine or placebo in the US or Tricontinental studies, although data from the European trial revealed that the 2-g, but not that 3-g, dose of MMF reduced death-censored graft loss compared with placebo: 9%, 13%, and 16%, respectively ( P = 0.03).
After FDA approval in 1995, MMF was rapidly incorporated into clinical practice in the US. By 1999, over 80% of the US kidney recipients were on MMF, usually in combination with CsA, and now its use is ubiquitous. From the beginning, pharmacoeconomic analyses documented benefit; the cost of adding MMF to a regimen was more than offset by reduced costs associated with diagnosing and treating acute rejection and its consequences. Subsequent analyses of clinical outcomes of almost 50,000 kidney recipients in the US Renal Data System (USRDS) suggested that prescription of MMF yielded significantly better early and late patient and graft survivals. The risk of late acute rejection episodes was reduced by 65%, with the risk of long-term deterioration of graft function reduced by 20% to 34%. More recently, Wagner and colleagues conducted a meta-analysis of MMF versus azathioprine. They included 23 studies with 3301 patients and demonstrated that MMF significantly reduced death-censored graft loss (RR 0.78, 95% confidence interval [CI] 0.62–0.99, P < 0.05) ( Fig. 15.5 ) and acute rejection (RR 0.65, 95% CI 0.57–0.73, P < 0.01), ( Fig. 15.6 ). Adverse events also had a slightly different profile with gastrointestinal symptoms being more common with MMF and thrombocytopenia and elevated liver enzymes with azathioprine.



Combination Therapy Utilizing MMF or Azathioprine in Kidney Transplantation
Cyclosporines
Shortly after the introduction of mycophenolates, CsA microemulsion (Neoral and generic, CsA-ME) began replacing its original oil-based formulation (Sandimmune). Data reported with the newer CsA-ME/MMF combinations generally confirmed the initial experience cited previously. The ELITE-Symphony trial, enrolling more than 1600 subjects, included two CsA-ME cohorts, normal and reduced-dose (with MMF) and daclizumab induction. Rejection rates after 1 year approximated 25%, similar to those observed previously. Alternatively, a multicenter European study using CsA-ME demonstrated only modest insignificant reductions in acute rejection episodes with MMF compared with azathioprine (34% and 35%, respectively, after 1 year) and questioned the value of a 10- to 15-fold cost differential. Five-year follow-up likewise documented no evidence of a long-term benefit of MMF versus azathioprine with CsA-ME.
Studies comparing MMF with sirolimus on everolimus (in combination with CsA) have typically shown comparable outcomes, with a differing side effect profile. With a significant percentage of CsA-treated recipients on standard doses of MMF or EC-MPS not achieving adequate exposure to MPA early after transplantation (see section on therapeutic drug monitoring later), a European trial examined the effect of a “loading dose” approach. Investigators found more adverse events but substantially less early rejection (3% vs. 17% of subjects) using higher-than-standard doses of EC-MPS (2880 then 2160 mg/day) for 6 weeks.
African Americans are generally considered at greater immunologic risk than others, and a subgroup analysis of the US pivotal trial documented a benefit of MMF for black recipients only in the 3-g dose group: an acute rejection rate of 12% compared with 32% in the 2-g group and 48% in the azathioprine cohort. These findings were seemingly confirmed in a single-center study: the standard 2-g dose of MMF produced no benefit in rejection risk among African Americans (relative risk 0.88), whereas white recipients fared much better (RR 0.35). Registry data, however, supported an overall benefit for African American recipients. Review of data from the pivotal trial and other studies found that the excess acute rejections among African Americans, and others, occurred quite early after transplantation, a time when MPA pharmacokinetics are not stable and MPA exposure at standard doses is low in a substantial number of recipients on CsA-based protocols. A subsequent study, conducted in stable renal allograft recipients, indicated no difference in MPA pharmacokinetics based on race or gender. In current practice, there is no evidence supporting long-term use of a 3-g dose in non-CsA-based regimens.
A 2003 paper regarding late kidney allograft failure identified chronic CsA-induced nephrotoxicity as its principal etiology. The study was based on serial surveillance biopsies in recipients treated with CsA, azathioprine, and corticosteroids. A subsequent study from the same group, however, substituted MMF for azathioprine, found substantially fewer changes suggestive of chronic allograft nephropathy and interpreted the data as indicating that MMF may attenuate CsA nephrotoxicity. Indeed, data from the earliest days of MMF use have suggested it may mitigate chronic allograft nephropathy. Patients in the USRDS registry who were maintained on MMF for at least 2 years were reported to show a 34% reduced risk of worsening renal function, with significantly less late rejection. A Spanish study indicated that MMF demonstrated a benefit for patients with chronic allograft nephropathy even when introduced late after transplantation, though contradictory data exist. It now appears that most late allograft failure is the result of immunologic mechanisms, with antibody-mediated injury playing a major role. The putative benefit of MMF in preserving renal function and preventing late graft failure may actually reside in its efficacy as an immunosuppressant with both anti-T cell and anti-B cell effects.
Tacrolimus
Studies evaluating the tacrolimus/MMF combination began appearing in the mid-1990s. A single-center, randomized study evaluated adding MMF to a tacrolimus/steroid regimen and documented a reduction of acute rejection rates at 1 year from 44% to 27%. A subsequent multicenter trial reported 2 g/day of MMF reduced the incidence of acute rejection episodes compared with 1 g/day or azathioprine (9%, 32%, and 32%, respectively). The low acute rejection rate with the higher MMF dose, without overt evidence indicating greater risk of infection or malignancy, was thought to support the 2-g dose as optimal with tacrolimus; however, the mean time to rejection was 79 days, there was almost no rejection beyond 180 days in the 1-g MMF group, and, by 1 year, patients in the 2-g group had undergone dose reduction to a mean of 1.6 g/day (see section on therapeutic drug monitoring, later). In contrast, despite a 1-year rejection rate of 15% with the tacrolimus/MMF combination, another study failed to show a significant benefit of tacrolimus versus CsA-ME in combination with MMF on the incidence of acute rejection episodes, graft survival, or patient survival in 220 subjects. A subsequent analysis of the Scientific Registry of Transplant Recipients data in recipients of living donor kidneys found greater risk of graft failure with the tacrolimus/MMF than with the CsA-ME/MMF combination. Although the Symphony trial documented fewer rejection episodes (12%) and better graft survival with tacrolimus/MMF than two CsA-ME-based control groups, a trial of modified-release tacrolimus documented efficacy and safety only similar to the CsA-ME/MMF control group. Eventually, efficacy and safety data obtained in two studies that compared tacrolimus with CsA-ME in combination with MMF led to the FDA’s approval of the tacrolimus/MPA regimen in 2009, at a recommended starting dose of 2g/day.
With tacrolimus/MMF established as a new standard, several reports documented similar efficacy of tacrolimus-based protocols with sirolimus. In a randomized, multicenter clinical trial comparing patients treated with MMF ( n = 176) versus sirolimus ( n = 185) (along with tacrolimus and steroids), there was no difference in rejection, graft survival, or patient survival. However, the MMF cohort displayed better renal function, less hypertension, and reduced hyperlipidemia. Among patients on a tacrolimus-based regimen, improvements in renal function were observed in 19 patients converted from sirolimus to MMF compared with 78 recipients remaining on sirolimus.
mtor Inhibitors
Mycophenolates have been utilized with sirolimus in an attempt to avoid CNIs altogether. Patients receiving sirolimus (and presumably everolimus) in combination with mycophenolate have substantially greater MPA exposure than patients on CsA, and many of the side effects of the two drugs are similar (e.g., diarrhea, myelosuppression), making the combination difficult for some patients to tolerate. However, an initial clinical study suggested that MMF paired with sirolimus was at least as effective as MMF/CsA to prevent acute rejection. In the Symphony study, one of four treatment groups received sirolimus after daclizumab induction in combination with MMF and corticosteroids. Almost 40% of the patients experienced acute rejection, with a high rate of study discontinuation, compromised graft survival, and relatively impaired glomerular filtration rate (GFR) in 1 year. In a conversion trial involving 830 patients switched between 6 and 120 months posttransplant from CNIs to sirolimus (in combination with MMF and corticosteroids), only subjects with baseline estimated GFR ≥40 mL/min demonstrated improved GFR 2 years later. Again, adverse effects were problematic, with converted patients experiencing more hyperlipidemia, anemia, diarrhea, stomatitis, and discontinuation from the study than those remaining on CNI-based therapy. A study of conversion from CsA-ME to everolimus demonstrated similar results: more acute rejection, with disappointing benefit in terms of GFR, adverse effects, and study discontinuation. Nonetheless, some studies have demonstrated, in patients able to tolerate the mTOR/mycophenolate combination, stability of kidney function over time and a trend to fewer malignancies.
Belatacept
Belatacept (Nulojix) is a monoclonal antibody that blocks costimulation of lymphocytes by inhibiting the interaction between CD80/86 and CD28. Approved for use in kidney recipients by US and European authorities in 2011, it is the first biologic agent to be utilized as maintenance therapy. It is administered intravenously at varying intervals after transplantation, and, from its earliest trials, has been combined with mycophenolate and corticosteroids. Two treatment regimens (moderate and low intensity) of belatacept have been studied in three large multicenter trials involving almost 1500 patients. Efficacy in preventing acute rejection and promoting graft and patient survival is comparable to CsA, with better preservation of renal function. There may also be a benefit of the belatacept/MMF combination in reducing the formation of de novo anti-HLA antibodies. In 2016, 7-year data from the BENEFIT study documented improved patient and graft survival among patients who remained on belatacept for the duration. Adverse effects were more common in a “moderate-intensity” belatacept cohort that received more of the agent early after transplantation; the “low-intensity” regimen is now approved for clinical use. The Emory group has recently published a novel protocol to reduce early acute rejection while capturing the long-term beneficial effect of a belatacept/MMF combination on renal function by adding tacrolimus for a short duration after transplantation.
The belatacept/MMF combination was associated with more posttransplant lymphoproliferative disease than in comparator patients, particularly in those naïve for Epstein-Barr virus before transplantation; the combination is contraindicated in such patients. A single case of PML has also been reported.
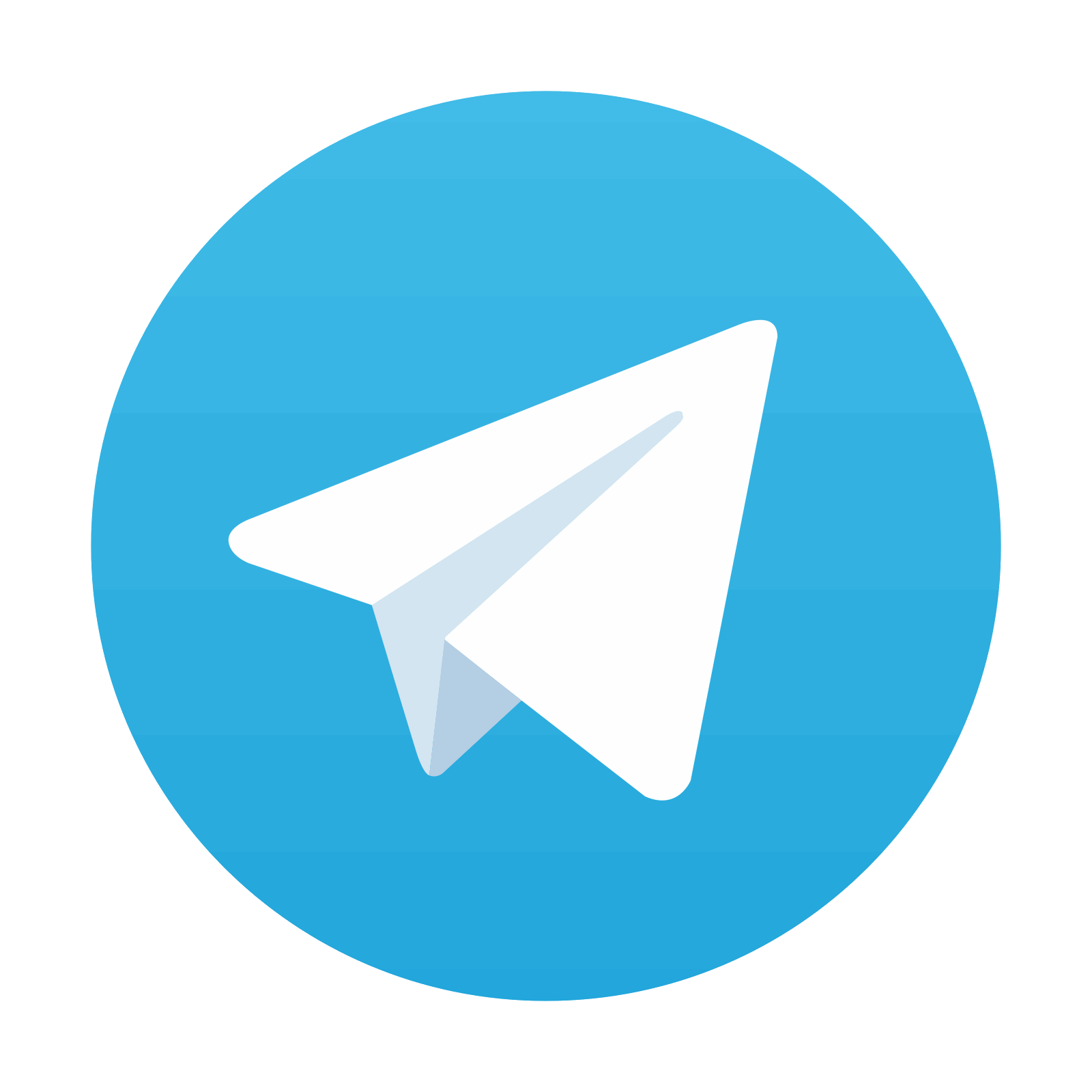
Stay updated, free articles. Join our Telegram channel

Full access? Get Clinical Tree
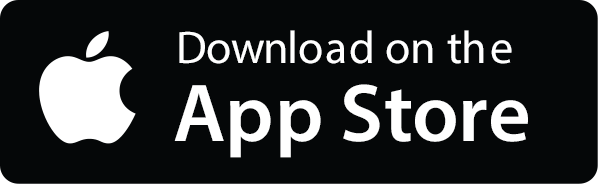
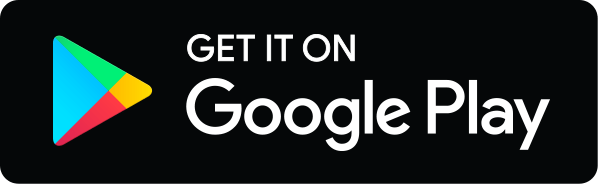