© Springer International Publishing AG 2017
Daniel C. Baumgart (ed.)Crohn’s Disease and Ulcerative Colitis10.1007/978-3-319-33703-6_1212. Autophagy and Endoplasmic Reticulum Stress
(1)
Division of Gastroenterology and Hepatology, Department of Medicine, Addenbrooke’s Hospital, University of Cambridge, Cambridge, UK
The two main types of inflammatory bowel disease (IBD ), Crohn’s disease (CD ) and ulcerative colitis (UC ), are characterized by pathologic immune activation directed toward the microbial flora, which arises from a complex gene–environment interaction [1]. While major inroads have been made into the genetic underpinning of disease [2], the environmental factors that trigger disease that could explain the dramatic rise in incidence and prevalence of CD and UC, initially in Europe and North America and more recently globally [3], remain entirely unknown.
Numerous innate and adaptive cell types have been demonstrated to have a role in the pathogenesis of IBD [1]. The intestinal epithelium with its specialized cell types has only more recently emerged as an important orchestrator of the mucosal immune response contributing an important homeostatic function, and importantly also providing a pathophysiologic function in the context of IBD. In particular, stress in the endoplasmic reticulum (ER) is commonly observed in the IBD epithelium, irrespective on the presence or absence of local inflammation [4–6]. ER stress arises when misfolded and hence potentially dysfunctional or toxic proteins accumulate in the rough ER [7]. Highly secretory cells are naturally particularly sensitive to ER stress due to stochastically occurring misfolding and the requirement that the cell’s folding capacity closely follows its translational output. In response to this type of stress, the Unfolded Protein Response (UPR ) is elicited, which is aimed at resolving stress by adapting the translational and protein folding capacity in the ER to increased demands, and by degrading misfolded proteins via ER associated degradation (ERAD) [7].
The UPR is organized along three main branches, each of which is characterized by a pair of an ER transmembrane sensor of misfolded proteins and its downstream transcription factor (IRE1–XBP1, PERK–ATF4, ATF6–ATF6f). In addition to transactivation of their specific target genes, the PERK branch of the UPR also triggers a transient halt in translation via phosphorylation of the elongation initiation factor 2α (eIF2α) [7]. The IRE1–XBP1 branch is the evolutionary most conserved branch. Remarkably, in addition to ubiquitously expressed IRE1α, the intestinal and bronchial epithelium expresses a second isoform of IRE1, IRE1β, possibly hinting toward a particularly important role of this UPR branch at inner body surfaces [8].
It has been demonstrated that genetic deletion of Xbp1 in intestinal epithelial cells results in ER stress due to a consequent impairment in the cells’ capacity to elicit the aforementioned adaptive program [6]. Remarkably, this cell-type specific induction of ER stress causes mild, superficial inflammation that is confined to the small intestine [6]. This is associated with a secretory defect and condensation of the ER in Paneth cells , specialized highly secretory cells at the base of intestinal crypts that are most well known for secreting large amounts of antimicrobial peptides [9]. This type of ER stress-induced small intestinal inflammation has been shown to be dependent on microbial signals as it does not develop in mice re-derived germ-free, and involves IRE1α overactivation and tumor necrosis receptor type 1 (TNFR1) signaling [10].
Importantly, manipulation of further UPR components also affects mucosal homeostasis. Deletion of Atf6α or P58 IPK , the latter an important ER chaperone, results in increased sensitivity to dextran sodium sulfate (DSS )-induced colitis [11], while deletion of Agr2 (anterior gradient 2), a ER protein disulphide isomerase gene family member, results in spontaneous ileocolitis and aberrant Paneth cell secretory apparatus [12, 13]. Furthermore, deletion of Chop, which is downstream of PERK-eIF2α and involved in mediating a terminal UPR with induction of apoptosis, is protective in DSS colitis [14]. Finally, a forward genetic screen has identified two independent mutations in Muc2, the gene encoding the major mucin component, which result in spontaneous colitis development [15]. Misfolding of the large MUC2 protein has been demonstrated to cause ER stress, and unresolved ER stress is thought to drive the colitis in this model [15]. Altogether, these data demonstrate the close mechanistic relationship between unresolved ER stress and the induction and propagation of intestinal inflammation.
The identification of a coding polymorphism in the autophagygene ATG16L1 , which leads to an alanine for threonine substitution at amino acid position 300 (ATG16L1 T300A ), exposed autophagy as a genetically affected mechanism involved in the pathogenesis of CD [16]. Despite its high prevalence in the general population (risk allele frequency in the Caucasian population is 52 %), ATG16L1 T300A is indeed one of the strongest genetic risk factors of CD [2]. Macro-autophagy (herein further referred to as “autophagy ”) is a fundamental biological process that describes the engulfment of intracellular content in double-layered membranes that form autophagosomes [17]. Autophagosomes fuse with lysosomes prompting the subsequent degradation of their content. Cargo that can be engulfed by autophagosomes includes any type of cytoplasmic content, organelles such as mitochondria (“mitophagy ”), infectious agents that have gained cytoplasmic access (“xenophagy ”), and macromolecular complexes (e.g., inflammasomes) [17]. Lysosomal degradation of this cargo releases amino acids and other basic building blocks of life, and hence autophagy is a central catabolic process of the cell. Consistent with this, evolutionarily it is thought to have evolved as a response to starvation. The T300A variant is juxtaposed to a caspase-3 cleavage site, rendering the risk variant susceptible to caspase-3-mediated cleavage of ATG16L1. Hence, under conditions of caspase-3 activation, such as during metabolic stress, death receptor signaling, or intracellular infection, ATG16L1 protein is degraded via this mechanism, resulting in hypomorphic autophagy induction in risk variant carriers [18]. ATG16l1 T300A is amongst the genetic risk factors with the largest effect size in Crohn’s disease , and it is indeed notable that the intracellular pattern recognition receptor NOD2, variants of which account for the largest fraction of heritability amongst all genetic loci associated with risk for inflammatory bowel disease (IBD) [2, 19, 20], can physically interact with ATG16L1, and this interaction is reciprocally impaired with ATG16L1 and NOD2 risk variants [21, 22]. Indeed it has been demonstrated that NOD2 is involved in induction of xenophagy upon intracellular bacterial infection with for example Shigella and Salmonella species [21, 22]. Altogether, this implicates two of the strongest genetic risk factors of Crohn’s disease involved in autophagy and suggests that their function may be directly related to each other under certain conditions. In addition to ATG16L1 and NOD2, variants of additional genes which encode for proteins involved in autophagy confer risk for IBD. This includes IRGM [23], an immunity related GTPase which binds to cardiolipin and induces autophagy via a mechanism that involves mitochondrial fission [24, 25]; NDP52 [26], a receptor involved in specific autophagy [27]; and LRRK2, encoding a protein that acts as a signaling hub, and which also mediates an important regulatory function on autophagy [28]. Altogether, these genetic observations hint toward alterations in autophagy as a major theme in IBD , in particular CD .
Remarkably, hypomorphic function of ATG16L1 [29, 30], NOD2 [31, 32], and LRRK2 [33] is associated with abnormalities in the secretory compartment of Paneth cells, both in murine genetic models and individuals and patients carrying risk alleles. As alluded to above, Paneth cells are specialized intestinal epithelial cells which reside at the base of small intestinal crypts, interspersed with crypt stem cells from which they differentiate and for which they provide the physiological niche [9]. Paneth cells are highly secretory cells with a characteristic elaborate granule network at their apical side that faces the crypt lumen. This granule network contains lysozyme and α-defensins (also known as cryptdins ), with granules secreted into the crypt lumen, thereby thought to protect the crypt by keeping this locale sterile [9]. Secretion of antimicrobial peptides by Paneth cells exerts a protective function towards pathogens and profoundly affects the composition of the intestinal microbiota [34, 35]. It has been speculated that alterations in microbial ecology (“dysbiosis”) imposed by host genetic alterations affecting Paneth cell function might play an important role in the pathogenesis of Crohn’s disease [36]. Indeed, alterations in the microbiota can indeed aggravate models of colitis exogenously induced via DSS [37], and specific constituents of the microbiota have an important role in the maturation and responsiveness of the mucosal (and indeed systemic) immune system [38]. However, since mice rendered genetically deficient or hypomorphic for NOD2 [31], ATG16L1 [29, 30] or LRRK2 [33] function do not develop any form of spontaneous intestinal inflammation, the cause-effect relationship of such microbial alterations for the initiation or propagation of intestinal inflammation remains a critically important, unresolved question. The lack of spontaneous inflammation in these murine models is also an important reminder on the critical importance of—entirely unknown—environmental factors that trigger disease in genetically susceptible individuals. Consistent with this, only 1 in 20 individuals homozygous for the NOD2 3020insC variant does actually develop Crohn’s disease [1].
Strikingly, hypomorphic function of ATG16L1 in healthy individuals and patients with CD carrying the ATG16L1 T300A risk allele [39], or in mice with genetic deletion of Atg16l1 in the intestinal epithelium [10] is associated with evidence of ER stress at the base of their intestinal crypts in Paneth cells. Conversely, ER stress induced via genetic deletion of Xbp1 in the intestinal epithelium causes autophagosome formation, again localized to Paneth cells [10]. The mechanism underlying autophagosome formation involves the PERK–eIF2α–ATF4–Chop branch of the UPR [10]. Pharmacological augmentation of autophagosome formation alleviates the superficial type of ileitis emanating from Xbp1 deletion in the intestinal epithelium, and this protective function is indeed only observable when autophagy function was intact in the intestinal epithelium [10]. Importantly, mice carrying deletions in both Xbp1 and Atg16l1 (‘Atg16l1;Xbp1 ΔIEC ’) in their intestinal epithelium spontaneously develop discontinuous, transmural fissuring ulceration in their terminal ileum, which closely resembles the histological presentation of ileal CD [10]. Notably, no inflammation is observed in the colon. Altogether, these data suggest that autophagy serves an important function in relieving ER stress, and in its absence unresolved ER stress can trigger a disease phenotype originating from the epithelium that phenocopies ileal CD . In this context it is also notable that amongst clinical phenotypes, ATG16L1 T300A is most strongly associated with ileal CD .
The intestinal epithelium and Paneth cells in particular appear particularly sensitive to the perturbations in their capacity to resolve ER stress. Protein folding is an energy-dependent process, profoundly influenced by perturbations in oxygen and nutrient (e.g., glucose) availability, which in itself might make the locale of the intestinal epithelium particularly susceptible. Furthermore, the cell’s protein folding capacity needs to strike a fine balance with the translational burden it experiences. A classic example is the transition of an activated B cells to a plasma cells with its associated vast expansion in ER and overall secretory apparatus, which is critically dependent on the UPR , in particular the IRE1-XBP1 branch [40, 41]. Consistent with this notion are also data that demonstrate that infection and immune activation with the associated inherent increase in protein production burden due to activation of transcription factors requires a fully operative UPR [42, 43]. Finally, microbial metabolites have also been shown to directly affect UPR function. It is therefore intriguing to speculate whether environmental factors may play a role as triggers for ER stress in the intestinal epithelium [44]. Remarkably, UPR -associated genes have indeed been identified as associated with genetic risk for developing IBD. Specifically, rare variants of XBP1 have been associated with both forms of IBD [6], and several other genes (e.g., ORMDL3, LRRK2) have been prioritized as highly likely causative genes located at established IBD risk loci [2].
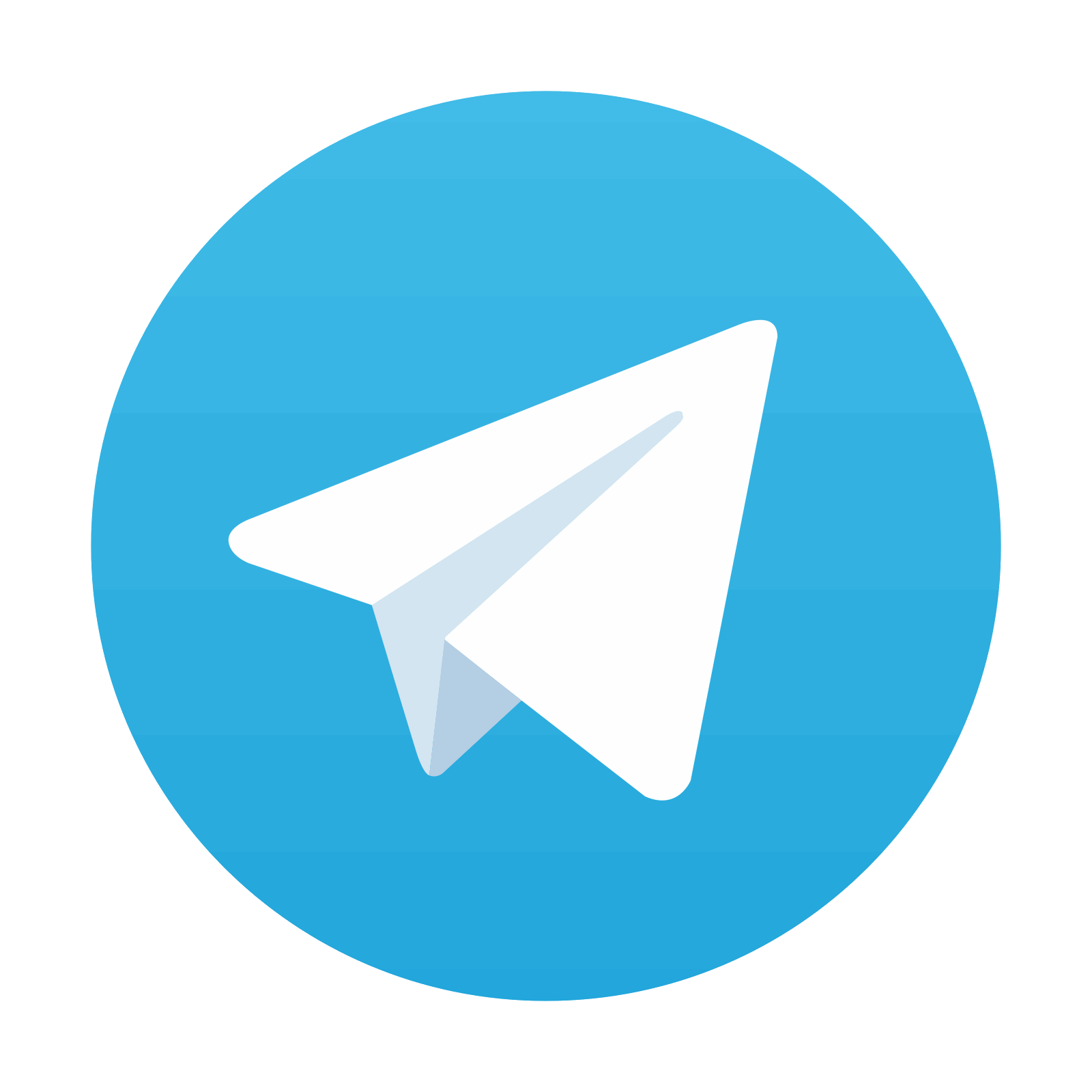
Stay updated, free articles. Join our Telegram channel

Full access? Get Clinical Tree
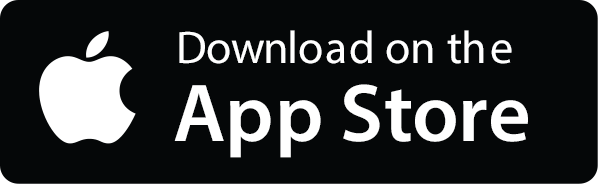
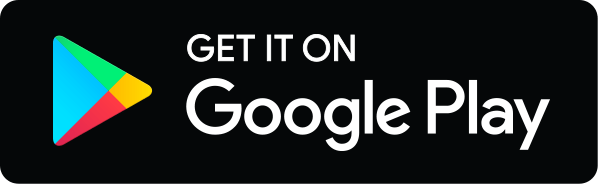