Ferdinando Agresta, Fabio Cesare Campanile and Nereo Vettoretto (eds.)Laparoscopic Cholecystectomy2014An Evidence-Based Guide10.1007/978-3-319-05407-0_14
© Springer International Publishing Switzerland 2014
14. Anesthetic Management for Laparoscopic Cholecystectomy
(1)
UOC Anestesia e Rianimazione ULSS 19 Adria (RO), Piazza degli Etruschi 9, Adria, 45011, Italy
(2)
Dipartimento Assistenziale Integrato Materno Infantile, AOU Careggi, Largo Brambilla 3, Firenze, 50134, Italy
Abstract
Pneumoperitoneum used for laparoscopic cholecystectomy (LC) causes important pathophysiologic alterations that every anesthetist must know. First of all cardiovascular changes are amplified when the patient assumes the reverse Trendelenburg position. In healthy patients these alterations are usually well tolerated. However, patients with cardiovascular disease or hypovolemia may be at increased risk. The increased intra-abdominal pressure also affects the respiratory system: thoraco-pulmonary compliance and functional residual capacity are reduced, while peak respiratory pressures are increased. These changes may lead to hypoxia. Mechanical ventilation with increased tidal volumes and the addition of positive end-expiratory pressure may counteract this problem. General anesthesia with tracheal intubation is the most commonly used, but in the last years supraglottic airway devices (SADs) have been investigated. The second-generation SADs improve pharyngeal seal and allow the passage of a gastric tube to deflate the stomach. SADs do not need neuromuscular blocking agents, but the use of these drugs for LC is still controversial. Spinal or epidural anesthesia may be safely used for LC. Recovery after this operation is usually rapid, and postoperative pain may be treated with the infiltration at all the trocar sites with local anesthetics, with the intraperitoneal nebulization of local anesthetics, and with a multimodal analgesia started before the induction of anesthesia. To prevent postoperative nausea and vomiting, prophylactic dexamethasone and droperidol or 5-HT3 antagonists may be used.
14.1 Introduction
Laparoscopic cholecystectomy (LC) is the treatment of choice for routine gallbladder removal and is one of the most commonly performed abdominal procedures in the Western world [1].
Benefits of laparoscopic procedures include reduced postoperative pain, quicker recovery times, earlier return to normal activities, shorter hospital stays resulting in overall reduction in medical costs, less intraoperative bleeding, fewer postoperative pulmonary complications and postoperative wound infections, and better postoperative respiratory function and cosmetic results [2]. Furthermore, there may be less internal scarring.
All these advantages represent only one side of the same coin: they should be balanced with potential adverse effects caused by pneumoperitoneum.
The physiological effects of intra-abdominal CO2 insufflation combined with the variations of patient positioning and with the effects of anesthetics can have a major impact on cardiorespiratory function.
Thus it is crucial for both the surgeon and the anesthesiologist to understand the physiological consequences of laparoscopy and to work in cooperation to achieve a good surgical outcome.
LC is most routinely performed with general anesthesia, but spinal or epidural block alone or together with a light general anesthesia has been used.
14.2 Pathophysiological Effects of Pneumoperitoneum During Laparoscopic Cholecystectomy
14.2.1 Cardiovascular System: Hemodynamic Effects of Pneumoperitoneum
The creation of pneumoperitoneum is the essential component for LC. It is obtained by insufflation of 2.5–5.0 L of carbon dioxide into the peritoneal cavity to permit adequate visualization of the abdominal viscera. The normal intra-abdominal pressure (IAP) is 5 mmHg or less [3], but pneumoperitoneum increases it to 12–15 mmHg. IAP above 10 mmHg is clinically significant, and above 15 mmHg can result in an abdominal compartment syndrome, which affects multiple organ systems. Increased IAP associated with pneumoperitoneum compresses venous capacitance vessels, causing an initial increase in preload and cardiac output, but later preload decreases significantly. Afterload is raised by the compression of the arterial vasculature resulting in a marked increase in systemic vascular resistance (SVR) while causing a decrease in cardiac output (CO). This change in SVR is generally greater than the reduction in CO, maintaining or even increasing blood pressure [4]. The extent of the cardiovascular changes depends on the interaction of several factors including the IAP attained, the volume of CO2 absorbed, patient’s intravascular volume, patient positioning (head-up tilt position), ventilatory technique, neurohumoral response, surgical conditions, and anesthetic agents used. The increase of SVR is caused by the release of catecholamines and vasopressin and by the activation of the renin-angiotensin system as well [5].
The decrease in CO is due to reduced venous return from compression of the inferior vena cava and from increased resistance in the venous circulation. CO typically decreases from 10 to 30 %. However, despite a decrease in intracardiac blood volume, intracardiac filling pressures may be elevated due to pressure transmitted across the diaphragm to the heart. There are analogous effects in the pulmonary circulation that manifest themselves as an increase in pulmonary vascular resistance (PVR) and a decrease in CO to the lungs.
The patient with normal cardiovascular function tolerates these hemodynamic changes well, and even if CO decreases, organ perfusion is maintained [6]. However, patients with cardiovascular disease or hypovolemia may be at increased risk and require particular attention to volume loading, positioning, and insufflation pressures [7]. Since the increased IAP during pneumoperitoneum is the main cause responsible for alteration of cardiac function, literature suggests a moderate to low IAP (<12 mmHg) to limit these effects [8]. On the other hand at IAP levels greater than 15, venous return decreases leading to decreased CO and hypotension [9].
14.2.2 Cardiovascular System: Hemodynamic Changes Due to Patient Position
In the reverse Trendelenburg position (head up) there are marked effects on the cardiovascular system because a decrease in venous return may lead to a fall in CO and mean arterial pressure. Young and healthy people can compensate it by increasing the heart rate and vascular resistances, but in the elderly patients with ischemic heart disease or cerebrovascular disease a severe hypotension may lead to myocardial or cerebral ischemia. These changes are amplified if these patients are hypovolemic. This may be the case of patients undergoing an urgent LC for acute cholecystitis presenting dehydration for prolonged vomiting.
Bradyarrhythmias, atrioventricular dissociation, nodal rhythm or even asystole can occur during insertion of the Veress needle and the trocar or during the insufflation of the abdomen that causes a sudden stretching of the peritoneum and a related profound increase in vagal tone [10] inducing a cardiovascular collapse even in healthy patients. Increased concentration of CO2 and catecholamines can cause tachyarrhythmias.
14.2.3 Respiratory Effects
The increased IAP displaces the diaphragm upward causing the reduction in lung volumes and a preferential ventilation of nondependent parts of the lung resulting in ventilation-perfusion (V/Q) mismatch.
The thoraco-pulmonary compliance and the functional residual capacity are decreased while there is an increase in peak airway pressure [11]. The reduced thoraco-pulmonary compliance together with the elevated airway pressures may cause pneumothorax and pneumomediastinum particularly in patients with pulmonary disease [12].
14.2.4 Other Effects
An elevated IAP causes an increase in intracranial pressure (ICP) by reducing cerebral venous drainage as a consequence of the higher intrathoracic pressure. The hypercapnia due to the absorption of CO2 from the peritoneal cavity may further raise the ICP, cerebral blood flow, and intraocular pressure. Blood flow to the kidneys, liver and gastrointestinal mucosa is reduced only when the IAP is increased over 20 mmHg. At this level renal cortical and medullary blood flow is reduced together with an associated reduction in glomerular filtration rate, urinary output, and creatinine clearance [6].
Increased IAP reduces femoral venous blood flow. This is due to the increased pressure on the inferior vena cava and iliac veins. Portal blood flow may be reduced as well leading to a transient elevation of liver enzymes.
14.3 Anesthetic Management
14.3.1 Preoperative Assessment
A careful preoperative assessment should be carried out before a laparoscopic procedure. It consists of a detailed history and a physical examination of the cardiovascular and respiratory systems associated with a thorough airway evaluation.
The preoperative laboratory evaluation depends more on patient’s status than on the procedure itself. Patients with cardiac or pulmonary diseases should be carefully assessed and may require additional investigations. Relative contraindications include severe ischemic heart disease, increased intracranial pressure, uncorrected severe hypovolemia, and patients with known right-to-left cardiac shunt.
Premedication is usually not necessary except in anxious patients.
14.3.2 Patient Monitoring
Standard intraoperative monitoring includes noninvasive blood pressure, electrocardiogram, pulse oximetry, airway pressure, and end-tidal CO2 (ETCO2). Monitors of the neuromuscular junction provide the measure of adequate muscle relaxation: train of four (TOF) might be routinely used. Invasive hemodynamic monitoring may be appropriate in patients with severe cardiorespiratory diseases [13]. Ventilatory monitoring of tidal volume, FiO2, and airway pressures is essential to detect the respiratory effects caused by the pneumoperitoneum.
ETCO2 concentration monitoring is mandatory during a laparoscopic procedure because CO2 is absorbed transperitoneally. Its elevation must be reduced, increasing the ventilation. In healthy patients the ETCO2 is correlated to the PaCO2, but in patients with compromised cardiopulmonary function, the gradient increases to become unpredictable, and only a direct arterial blood gas analysis can reveal a hypercarbia. A sudden fall of ETCO2 can be caused by a severe hypotension or air embolism.
If a total intravenous anesthesia (TIVA) is preferred, the use of a bispectral index (BIS) can help to reduce the occurrence of awareness.
14.3.3 Anesthetic Techniques
Both general and regional anesthetic techniques have been performed for LC. General anesthesia with endotracheal intubation is certainly the most commonly used. Balanced anesthesia and TIVA are equally performed: short-acting inhalational agents such as sevoflurane or desflurane or ultrashort-acting opioids such as remifentanil are usually preferred, as many LC are done on an outpatient basis. Target controlled infusion (TCI), an evolution of TIVA in which a plasma or a site-effect drug concentration of propofol and remifentanil or other opioids can be achieved and maintained by using a pharmacokinetic model included in the infusion pump, is particularly useful in this setting because it allows a better control of the anesthesia level and hence of the patient’s awakening at the end of the operation too.
The use of N2O during laparoscopic procedures remains controversial because it has been reported to increase the incidence of postoperative nausea and vomiting (PONV), and a recent study confirmed this correlation [14].
Whatever technique is chosen, at induction of anesthesia, intravenous administration of atropine prevents vagally mediated bradyarrhythmias that can occur during the institution of pneumoperitoneum.
Since 2002, several clinical studies have investigated the use of supraglottic airway devices (SADs) in laparoscopic procedures [15, 16]. In the LC general anesthesia without tracheal intubation can now be performed suitably and effectively with second-generation gastric access SADs like the ProSealTM and the SupremeTM laryngeal mask airway (LMA) [15–17]. Data from larger cohort are needed to determine whether any of the newer SADs (i.e., the i-gel®) offer the same advantages [18, 19]. Second-generation SADs improve pharyngeal seal and allow a passage of a gastric tube (12, 14, 16 Fr) to deflate the stomach. In such a way gastric content reflux is prevented, surgical view is improved, and at the same time gastric injury on trocar insertion is avoided. Usually the drain tube is left in situ until the surgeon has checked the stomach: if it is deflated, the drain tube should be removed. If left inside, the drain tube can cause obstruction, preventing a possible reflux from the stomach.
The main concern that prevents the diffusion of SADs in laparoscopy is the risk of gastroesophageal reflux that the high IAP produced by the pneumoperitoneum might increase. This is not the case, however, because the elevated IAP increases the lower esophageal sphincter tone [20]; moreover, the reverse Trendelenburg position adopted for LC makes the regurgitation of gastric content more difficult, and finally if it does happen, the drain tube allows venting of the stomach content.
The use of SADs results in less hemodynamic and hormonal activation, less postoperative cough, and less postoperative nausea and vomiting (PONV); shortens the recovery time; and decreases early- and late-onset sore throats [21, 22].
Neuromuscular block is achieved using short-acting agents such as cisatracurium or agents that can be antagonized immediately and completely such as rocuronium.
However, the need for muscle relaxant during laparoscopy is still controversial: for example, in patients undergoing laparoscopic gynecological surgery, the use of ProSeal LMATM and SLIPATM was as effective as the use of tracheal tube; they did not require muscle relaxants and gave fewer side effects [21]. Yet other authors suggest that neuromuscular blocking agents give a better surgical view and allow to decrease the IAP from 12 to 8 mmHg, and in this way postoperative pain is lowered [23]. This deep neuromuscular block must be maintained until a few minutes before the end of the operation. Hence, the time for the reverse of this block is very short. Only sugammadex can reverse the neuromuscular block immediately and completely, thus allowing a safe and quick discharge of the patient from the operating room. Unfortunately this drug is expensive and for this reason it is not largely available in Italy. On the other hand, the “curare-free” TCI anesthesia allows a quick discharge of the patient to the ward.
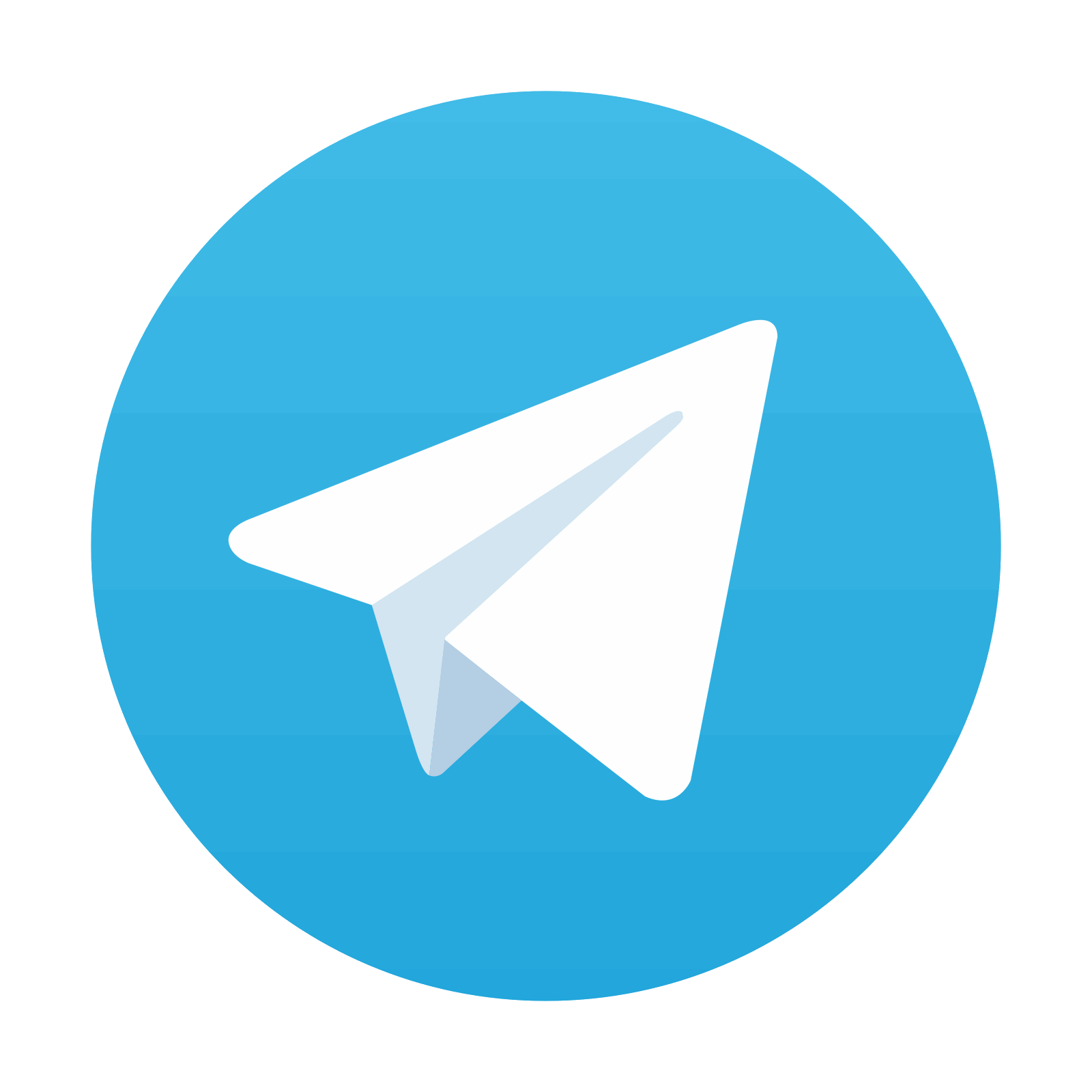
Stay updated, free articles. Join our Telegram channel

Full access? Get Clinical Tree
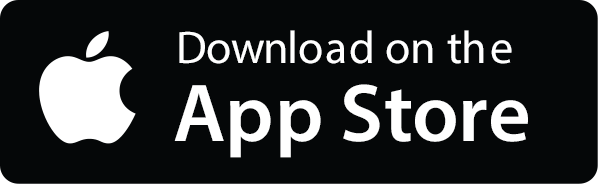
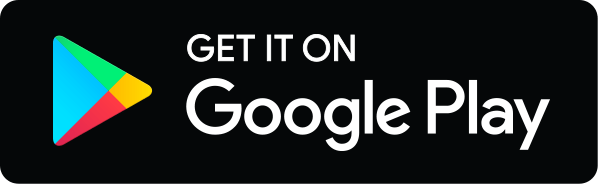