© Springer International Publishing Switzerland 2015
Andrea Lenzi, Silvia Migliaccio and Lorenzo Maria Donini (eds.)Multidisciplinary Approach to Obesity10.1007/978-3-319-09045-0_11. Anatomy and Physiology of Adipose Tissue
(1)
Department of Basic Medical Science, Neuroscience and Sense Organs, University of Bari, Piazza Giulio Cesare 11, Bari, 70124, Italy
1.1 Overview of Adipose Tissue
The adipose organ has been traditionally classified into white (WAT) and brown adipose tissue (BAT). WAT is the storage organ of energy in the form of lipids, whereas BAT regulates body temperature by producing heat via the expenditure of stored energy. The morphology of white and brown adipocytes is reasonably different, because these lipids are organized in a unique droplet in white adipocytes (unilocular) and in many droplets in brown adipocytes (multilocular) [1]. Another feature that differs in the morphology of these two cell types is the presence of numerous large mitochondria in brown adipocytes. These latter are also characterized by the expression of uncoupling protein 1 (UCP1), the protein able to uncouple oxidative phosphorylation from ATP synthesis, resulting in the production of heat [2].
Interestingly, UCP1 immunoreactive brown adipocytes are present in all depots of the adipose organ [3]. These adipocytes are also named “beige” [4] or “brite” (brown in white) [5], and their origin is debated [6]. These brown/beige/brite cells in WAT are derived from precursor cells that could be different from classical brown adipocytes and are closer to the white adipocyte cell lineage [7]. However, in response to certain stimuli, these adipocytes can be activated into cells having BAT-like phenotype, as demonstrated by the expression of UCP1 and other BAT-like genes [8]. This process, named browning response, is a transdifferentiation program from white-to-brown phenotype, and it will be discussed below.
Although the presence of adipocytes in the bone marrow is well recognized, the role of these cells has been recently better defined. Traditionally, marrow adipocytes were described as passive cells, occupying space not required for hematopoiesis or bone formation [9], but it has been finally documented that these cells have an active role in the bone marrow microenvironment. Hence, marrow adipose tissue (MAT) is considered a type of adipose tissue, holding different features from white and brown adipose tissue. At birth, the bone marrow is mostly hematopoietic (the red marrow), but, throughout life, it is progressively transformed to fatty yellow marrow [10]. Therefore, the marrow adiposity expansion is age related in both genders [11]. The earliest fatty conversion of the human marrow begins at birth, accelerates between 4 and 8 weeks of age, and reaches full fatty conversion after 1 year [12]. MAT continues to accumulate in the appendicular skeleton from distal to proximal until age of 20–25, and, throughout life, gradual MAT formation continues in the axial skeleton [13]. Although in young subjects the content of marrow fat appears higher in men than women [14], this trend is reversed in elderly subjects, so that older women have higher bone marrow adiposity [15]. This age-related increases in marrow fat content are defined hypertrophy, if depends on increased size, or hyperplasia, if depends on increased number of adipocytes in the marrow. As physiological role, MAT serves as a local storage for energy. In pathological conditions, such as in state of calorie restriction and anorexia, although there is a considerable reduction in the volume of WAT, the amount of MAT appears strongly increased [15]. For long time, MAT has been considered a negative regulator of bone formation. Hence, MAT accumulation are correlated with osteoporosis and osteopenia [16]. Despite these correlations, it is unclear whether excessive MAT could directly influence bone metabolism or the bone loss occurring provokes changes in the marrow microenvironment that indirectly influence MAT formation.
A fourth type of adipocyte has recently been characterized in mouse subcutaneous fat depots during pregnancy and lactation, when the adipose portion of the mammary gland gradually disappears and an extensive development of milk-secreting lobuloalveolar epithelial glands appears. These newly formed epithelial cells, called pink adipocytes, derive from a direct transdifferentiation of white adipocytes into milk-secreting epithelial cells (adipo-epithelial transdifferentiation) [17]. The formation of pink cells is reversible, and, following end of lactation, they revert into white adipocytes, restoring the adipose component of the mammary gland [17, 18].
1.2 The Anatomy of the Multi-depot Adipose Organ
White and brown adipocytes have different morphology and functions, but numerous studies have shown that, in several locations, both cells cohabit together, forming the multi-depot adipose organ [19]. These depots are located in the subcutaneous space (subcutaneous depots) or close to organs located in the trunk (visceral depots).
The anterior subcutaneous depot is mainly located in the upper dorsal area at the level of the scapulae. It is composed by interscapular, subscapular, axillary, and cervical depots [20]. The posterior subcutaneous depot is located primarily in the lower ventral part of the body, and it is also formed of different parts: dorsolumbar, inguinal, and gluteal depots. The truncal or visceral depots are contained in the mediastinum and abdomen. All truncal depots are intimately associated with the aorta. Furthermore, in females, it has been also described another anatomical form of fat called the abdominopelvic depot, which comprises perirenal, periovarian, parametrial, and perivesical fat [3, 20]. Of note, the subcutaneous tissue in female mice has been found infiltrated by ramified epithelial ducts ending in five symmetrical pairs of nipples. Therefore, it can be considered as part of mammary glands subcutaneous depots [21, 22].
Pericardic, omental, mesenteric, and subcutaneous depots are characterized by the presence of lymphatic tissue [23]. All adipose depots are associated with specific nerve-vascular peduncles [24], enabling the connection with the nervous system in order to respond to physiological and environmental stimuli. Most of nerve fibers in contact with adipocytes express tyrosine hydroxylase (TH), the enzyme marker of noradrenergic fibers [24]. When mammals are exposed to temperatures below thermoneutrality, the sympathetic nervous system is activated because thermogenesis is required. This implies the action of norepinephrine on beta-3 adrenoreceptors, which promotes the molecular pathway for thermogenesis in brown adipocytes [2]. Interestingly, patients affected by pheochromocytoma, a norepinephrine-secreting tumor of the adrenal gland, show increased amount of BAT detectable by positron emission tomography (PET) [25]. Moreover, elegant data have shown that the density of TH-expressing nerve fibers is much higher in the brown adipose tissue than in white, and the density of these fibers increases during cold exposure, as well as increase the number of brown adipocytes [26]. Therefore, adipose tissues exposed to cold temperature appear browner and more innervated than those exposed to a warm environment.
In contrast, aging seems to have an opposite effect on white and brown fat balance. Thus, experiments performed in rats demonstrated an increase in the number of white adipocytes in the anterior subcutaneous depot correlated with the increase of age and with a concomitant progressive reduction in the number of brown adipocytes [27]. In agreement with this, human studies showed that BAT seems to be replaced by WAT in elderly [28]. Interestingly, cold exposure restores the numbers of brown adipocytes to the levels of young animals [29], and similar effects have been found in humans [30].
Large white adipocytes are mainly present in subcutaneous depots, whereas small white adipocytes are mostly located in visceral depots [31]. Brown adipocytes in visceral depots are mainly found near the aorta. Because beige/brite adipocytes were recently defined [32], brown adipocytes are termed classical or constitutive brown adipocytes to distinguish them from brown-like cells in WAT. Constitutive brown fat is predominantly located around interscapular, paravertebral, and perirenal sites. The most classical brown fat depots reside subcutaneously between the shoulders and can be easily excised. In contrast, perirenal BAT is difficult to remove without removing the kidney. Small areas of interscapular BAT could also reside in the supraclavicular region [33]. Conventionally, BAT was thought to be restricted to the neonatal and early childhood periods [33]. However, PET scanning analysis has showed the presence of active BAT in adult humans, particularly in the upper trunk, such as cervical, supraclavicular, paravertebral, pericardial, and to a certain extent, in mediastinal and mesenteric regions [34].
1.3 Adipose Cell Differentiation
The formation of WAT begins shortly after birth. Mesenchymal stem cells become adipoblasts and afterward differentiate into preadipocytes. The primary phase of adipogenesis is characterized by the proliferation of preadipocytes, which move forward into mitosis until they reach growth arrest. At this point, if preadipocytes exit the cell cycle, they change their morphology and accumulate cytoplasmic triglycerides, gaining characteristics of mature adipocytes and loosing the ability to divide [35]. Both white and brown adipocytes originate from the mesoderm, but they derive from different precursor cells. Mesenchymal stem cells can be committed to either a Myf5-negative adipogenic and osteoblastogenic lineage (white adipocytes) or to a Myf5-positive myogenic lineage (brown adipocytes) [36]. Although the adipocytes originate from two different lineages, the differentiation of adipocytes shares common transcriptional pathway involving CCAAT/enhancer-binding proteins (C/EBPs) [37].
In white adipocytes, once differentiation is active, the cAMP response element-binding protein becomes phosphorylated and then induces the expression of C/EBP-β [38]. The mitogen-activated protein kinase and GSK3β phosphorylate C/ EBP-β, which leads to dimerization of C/EBP-β, thus inducing a DNA-binding domain. The binding of C/EBP-β to DNA allows preadipocytes either to reenter the cell cycle or to increase levels of transcripton factors C/EBP-α and peroxisome proliferator-activated receptor-γ (PPAR-γ) [37]. PPAR-γ is crucial to promote lipid and glucose metabolism and insulin sensitivity of adipocytes.
BAT develops before birth because it plays role in protecting newborn against cold. The differentiation of brown preadipocytes into mature brown adipocytes is positively and negatively controlled by bone morphogenetic protein (BMP)-7 [39] and myostatin [40], respectively. C/EBP-β and PR domain containing 16 (PRDM16) have been shown to act as key transcriptional factors in the differentiation of brown adipocytes [41]. Thus, when PRDM16 was suppressed in brown precursor cells, the cells differentiated into skeletal muscle cells. Likewise, myoblasts in which PRDM16 was ectopically expressed are able to transdifferentiate into brown fat cells [41]. The fate of BAT from the myogenic lineage depends on transcriptional complex formed between PRDM16 and C/EBP-β [42] that induces the expression of peroxisome proliferator-activated receptor gamma coactivator-1 alpha (PGC-1α) [43], which in turn regulates mitochondrial biogenesis and oxidative metabolism [44, 45]. Furthermore, C/EBP-β is a key transcriptional activator that leads to UCP1 expression, inducing the thermogenesis process [42].
1.4 Transdifferentiation Process
The white adipose depots have the ability to switch between energy storage and energy expenditure. Thus, in specific physiologic conditions, white adipocytes transform into brown adipocytes to contribute to thermogenic needs [46].
As mentioned above the beige/brite cells in WAT are derived from precursor cells that are different from classical brown adipocytes. These depots can shift from a WAT phenotype to a BAT-like phenotype showing morphology, gene expression pattern, and mitochondrial respiratory activity quite identical to those of classical BAT [47]. This induction of the brown adipocyte-like phenotype in WAT is called browning response [7]. Understanding the molecular mechanisms of this process is taking great relevance for human health since it could be used for developing therapeutic strategies to prevent the excessive formation of white adipocytes, in favor of increasing amount of brown adipocytes. The strongest inducer of beige/brite cells activation is cold exposure via several molecular mechanisms. This conversion is principally mediated by the sympathetic nervous system through the beta3AR because it has been shown that browning response can be induced by the administration of beta3AR agonists [48] and, additionally, beta3AR knockout mice do not respond to the browning transdiffrentiation [8].
Transcription factors such as PPAR-γ, its coactivator 1 alpha (PGC-1α), C/EBP beta, and PRDM16 seem to play key roles in regulation of the browning program [43]. The thiazolidinediones, a group of PPAR-γ activator agents, are synthetic ligands used to control glycemia [49], and they have proved effective in transforming WAT into BAT-like tissue in mice, rats, and dogs [50–52]. PGC-1α, originally described as a cofactor of PPARγ, is inducible by cold exposure in BAT [53]. Furthermore, human white adipocytes transfected with PGC-1α express UCP1, the marker gene of brown adipocytes [54]. C/EBPβ and PRDM16 are powerful inducers of the brown phenotype of fat cells. They form a molecular complex that is essential for the brown differentiation of mesenchymal stem cells. Hence, transplantation of skin fibroblasts, in which C/EBPβ and PRDM16 were ectopically expressed, gave rise to functional BAT [42]. Moreover, PRDM16 transgenic expression in all adipose tissues induced browning of WAT, particularly in subcutaneous fat, enhancing energy expenditure and suppressing weight gain in high-fat diet [55].
Fibroblast growth factor 21 (FGF21) is a growth factor produced by the liver and BAT under the control of sympathetic nervous system [56]. It has been recently shown that FGF21 acts in an autocrine/paracrine manner to increase expression of UCP1 and other thermogenic genes in fat tissue through the β-Klotho receptor [57]. FGF21 modulates this process by enhancing PGC-1α protein levels in adipose tissue [58]. Conversely, transforming growth factor beta (TGF-beta) negatively affects the browning process. Thus, the absence of smad3, a downstream molecule of TGF-beta signaling, induces white-to-brown conversion with positive metabolic response on glucose levels in diet-induced obese mice [59]. BMP7 stimulates a full program of brown adipogenesis through PRDM16 and PGC-1α activation and promotes commitment of mesenchymal progenitors to a brown adipocyte lineage. Furthermore, adenoviral-mediated expression of BMP7 in mice induces significant increases in BAT that consequently enhances energy expenditure and reduces weight gain [39].
Cold exposure is not the unique stimulus triggering the browning process. Interestingly, a recently discovered hormone called irisin, which is produced by skeletal muscles during physical exercise, has been proven to be a potent inducer of white-to-brown conversion both in vitro and in vivo [60].
The ability to transform itself according to the need of more energy consumption is defined as plasticity of white adipose tissue. However, this extraordinary plasticity is not solely related to the browning response. During pregnancy and lactation, all subcutaneous depots of the adipose organ can turn into mammary glands [22]. During pregnancy, white adipocytes lose their lipid content, acquire epithelial features, and form glandular structures able to produce and secrete milk. Following lactation, part of the epithelial component of the gland converts into adipocytes, allowing recovery of the adipose component in the subcutaneous depot [22].
The adipose cell plasticity and ability to adapt to environmental stimuli, modifying their phenotype, satisfy important physiological needs of the organism. These new insights in the adipocyte biology establish that these cells have a multipotent differentiation potential in vitro [61] and in vivo [22].
1.5 Adipokines and Cytokines
Considerable evidence shows that molecules secreted by adipose tissue play a key role in metabolic homeostasis, and their deficiency, caused by massive adiposity or adipocyte dysfunction, leads to obesity or several obesity-related pathologies. Therefore, adipose tissue is considered an endocrine organ whose action on target tissues depends on the location of fat depots and may differ according to its functional state. The full understanding of adipose tissue secretome might provide significant insights into the functions of the most important metabolic regulator of the body. Leptin is one of the most potent adipokines in metabolic regulation. It controls body weight by signaling sense of appetite or satiety to hypothalamus, which produces neurotransmitters that adapt food intake and energy expenditure. Leptin also regulates hepatic lipogenesis by suppressing fatty acid synthesis pathway [62] and improves muscle fatty acid oxidation [63]. Adiponectin is the most abundant secreted adipokine. It enhances insulin sensivity and partially reverse insulin resistance in obese mice [64]. Currently, researches for treatment of obesity-induced inflammation and insulin resistance are exploring strategies to increase adiponectin levels or adiponectin receptor activities [65].
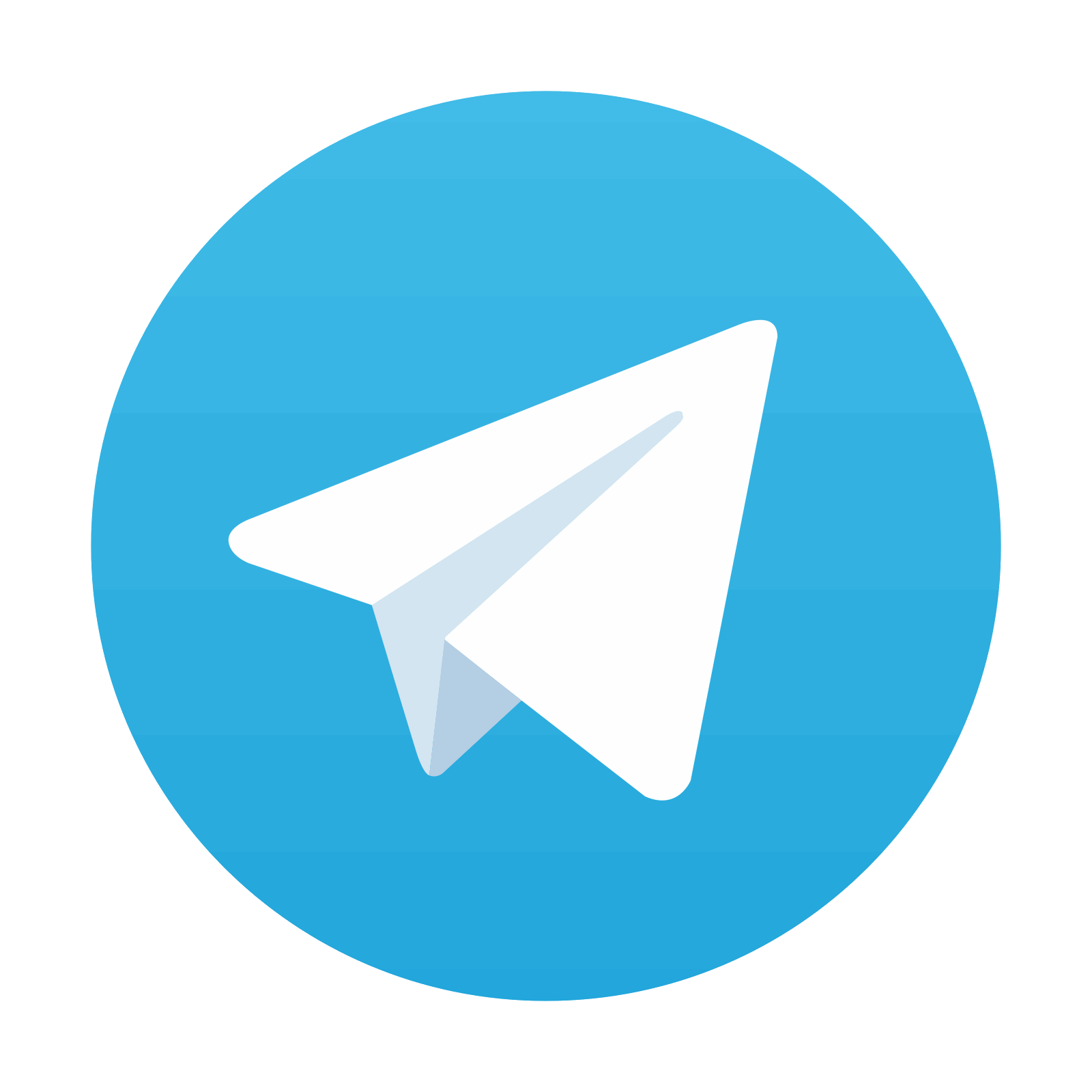
Stay updated, free articles. Join our Telegram channel

Full access? Get Clinical Tree
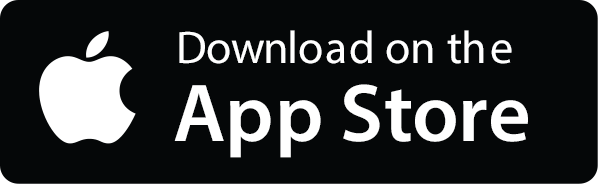
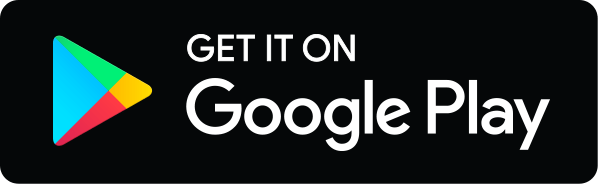