Fig. 1.
Normal gastric slow waves. Gastric slow waves recorded from electrodes implanted on the serosal surface of the stomach along the greater curvature in a healthy dog (1.5 min recording). The top tracing was obtained from a pair of electrodes 16 cm above the pylorus, and the bottom one was from the electrodes 2 cm above the pylorus (Courtesy of Jiande Chen, Ph.D).
Similar to the arrhythmias of cardiac electrical function, dysrhythmias are irregularly timed and located and/or ineffective myoelectrical activity. For example (Fig. 2), there can be an ectopic pacemaker in the distal stomach in addition to the normal pacemaker in the proximal stomach. The ectopic pacemaker generates slow-wave potentials at a higher rate than normal, leading to tachygastria along with retrograde propagation toward the proximal stomach. This may interfere with normal waves and lead to disruption of the normal gastric contractions.


Fig. 2.
Tachygastria. Gastric slow waves recorded from electrodes implanted on the serosal surface of the stomach along the greater curvature showing the ectopic tachygastrial activity in the distal stomach (arrow). The top tracing was obtained from a pair of electrodes 16 cm above the pylorus, and the bottom one was from the electrodes 2 cm above the pylorus (Courtesy of Jiande Chen, Ph.D).
The prevalence and origin of various gastric dysrhythmias have been investigated in canine models. Most bradygastric impulses originate in the proximal stomach (80.5 ± 9.4 %) and propagate to the distal antrum. Therefore most bradygastria is attributed to a decrease in the frequency of the normal pacemaker of the myoelectrical activity of the stomach. Tachygastria by contrast originates in the distal antrum (80.6 ± 8.8 %) and propagates in a reverse fashion toward the proximal stomach. In the setting of tachygastria, the normal proximal pacemaker slow waves may or may not be present. This can lead to slow waves in one direction and distal tachygastria, leading to overall dysrhythmia [9].
Gastric motility is different in fed and fasting states. In the fed state, the human stomach contracts at the maximum frequency of 3 cpm as discussed earlier. In the fasting state, there are periodic fluctuations divided into three phases. Phase I lasts 40–60 min with no contraction activity, phase II lasts 20–40 min with intermittent contractions, and phase III involves regular rhythmic contractions lasting 2–10 min. In the fed state, the contraction begins in the proximal stomach and follows the propagation of the spike potential as it continues toward the pylorus. In healthy subjects 2 h after a meal, 50 % or more of a meal is cleared by the contractions of the stomach, and at 4 h, 95 % of the meal should be cleared. Once emptied the motility pattern of the stomach changes [10].
Gastric emptying plays an important role in food intake regulation. Distension of the stomach acts as a satiety signal [11]. Meanwhile, rapid gastric emptying can be directly linked to some episodes of overeating and obesity. There have been numerous animal studies linking lesions in the hypothalamus to overeating and obesity [12]. There are also multiple studies which report higher rates of gastric emptying in obese subjects without a clear explanation at this point [13].
Innervation of the stomach comes from two sources: parasympathetic fibers via the vagus and sympathetic fibers via the celiac plexus. The vagus nerve has both efferent and afferent fibers, but it has predominately afferent fibers. The efferent fibers originate in the medulla and synapse with neurons located in the myenteric and submucosal plexus of the stomach. This leads to acetylcholine release and increased gastric motor function and gastric secretions. The afferent fibers are predominant, making up approximately 90 % of the fibers. And they carry stimuli signals from the gut back to the brain. Multiple gastric peptides have also been implicated in this pathway including serotonin, substance P, ghrelin, cholecystokinin (CCK), and somatostatin [14].
The true mechanism of action for weight loss from vagotomy is not completely elucidated. This technique was first indicated in epilepsy [15] and severe, therapy-resistant depression [16]. Vagal pacing showed to diminish food intake, fat mass, and weight in pigs [17], rats [18], and obese minipigs [17], suggesting that VNS induces satiety signals. Body weight was found to be reduced mainly at the expense of body fat, whereas metabolic rate remained unaffected [19]. Depressed patients undergoing VNS were also reported to have less sweet cravings [20] and to lose weight [19, 20]. However, discussion remains on both the ideal positioning of the electrode and the frequency of the blocking algorithm.
Animal studies have looked at the effect of destruction of just the afferent fibers of the vagus in comparison to complete vagal interruption for weight loss. The blockade of afferent fibers was previously shown to downregulate the intestinal sodium-glucose cotransporter SGLT-1. Both treatments showed weight reduction in a rat model but a statistically higher rate of weight loss for complete vagal interruption (19 % for total vagotomy, 7 % for selective deafferentation). The study also revealed a significant difference in the decrease in the amount of visceral fat (52 % vagotomy vs. 18 % deafferentation) [21].
Vagal interruption in humans has been shown in a few studies to result in weight loss. This has been demonstrated over the last 35 years including early reported experiences of vagotomy for refractory obesity [22]. The effects of truncal vagotomy include preferential emptying of liquids and early satiety secondary to reduced receptive relaxation. However, dumping syndrome and diarrhea can result.
Aside from a few published studies, the clinical benefits of vagotomy have not been widely appreciated. Many surgeons have included truncal vagotomy with gastric bypass surgery; there are no reports which have shown that vagotomy increased the weight loss achieved with gastric bypass. There are many explanations for this inconsistency. Firstly, gastric bypass is such a robust weight loss procedure that it shadows any potential benefit of vagotomy. Secondly, the physiologic changes after gastric bypass negate the effects of vagotomy. Lastly, ligation of the vagus nerves activates compensatory mechanisms that overcome the benefits of vagotomy. Therefore, permanent ligation of the vagus nerves as a primary or adjuvant treatment for weight loss fell out of favor.
Gastric Stimulation and Pacing
Given the physiologic understanding of how intrinsic gastric pacing changes gastric emptying, one can postulate that manipulation of this physiology could create dysregulated pacing and also change satiety and caloric intake. Unfortunately, these mechanisms are still not completely understood as efforts to induce weight loss this way have had variable results.
A number of papers have described electrical stimulation for gastrointestinal dysmotility. The aim is to normalize the underlying rhythm by entraining the antegrade stimulation of the myoelectrical activity of the stomach. The concept of entraining gastric electrical activity to facilitate “proper” gastric motility is based on the hypothesis that electrical rhythm disturbances are the underlying reason for a variety of gastric motility disorders, including gastroparesis and possibly functional dyspepsia [23]. Capture and control of the electrical signal is dependent on both the width and the frequency of the stimulation pulse [24]. McCallum et al. [25] demonstrated in patients suffering from gastroparesis that antegrade gastric pacing could entrain gastric slow waves in all nine patients. They paced the greater curvature of the stomach at frequencies approximately 10 % higher than the slow-wave frequencies measured. In two patients, it converted tachygastria to normal slow waves.
Based on the physiology of normal gastric propagation leading to stomach clearance, it is postulated that retrograde pacing or disentrainment may slow emptying in patients with rapid gastric emptying, and based on studies suggesting obese patients may have faster gastric emptying, the principle of retrograde pacing may lead to slowed emptying, increased satiety, and lower caloric consumption. An artificial pacemaker is connected to the distal stomach along the lesser curvature, resulting in electrical waves propagating from the distal to the proximal stomach. These waves conflict with the normal and physiologic electrical waves that propagate from the proximal to the distal stomach. Consequently, gastric dysrhythmia is induced, and the regular propagation of gastric electrical waves is impaired. The severity of impairment is determined by the strength of the electrical stimulation [26].
However, to date, there have been no clinical studies to demonstrate the effectiveness of normalizing the antegrade stimulation in treatment of gastroparesis. In fact, only several studies published reports about spontaneously existing intermittent “tachygastria” and “bradygastria” in humans, and their impact on gastric emptying remains unclear [27, 28].
Implantable Gastric Stimulation for Weight Loss
Conceptualized first by Italian surgeon Valerio Cigaina in the late 1980s, the concept of stimulation of the stomach has been shown to be safe and effective. At that time, he hypothesized that exogenous electrical impulses could be used to dysregulate normal gastric electromotor activity in obese patients, resulting in weight loss.
In 1996, Cigaina et al. reported that retrograde gastric electrical stimulation was both safe and effective in moderating weight gain in a porcine model. Three groups were studied, and once beyond 12 weeks, a statistical difference in weight loss appeared. Weight was 10.5 % less than for sham controls [29].
The initial human studies began in 1995. Four women with a BMI of 40 or greater were implanted and followed for up to 40 months. Via laparoscopy, patients had platinum electrodes implanted intramuscularly on the anterior gastric wall, adjacent to the lesser curve and attached to a prototype generator. No dietary instructions were given. At 40 months after implantation, two patients had significant weight loss of 32 and 62 kg. Malfunctions in their stimulator system were discovered in the other two patients with fracture of the lead. Neither lost significant weight, highlighting the importance of bipolar stimulation.
Initiated in 2000, the first US investigation (US O-01) was a multicenter, randomized, controlled, double-blinded trial that was developed to evaluate both the safety and efficacy of the commercially produced implantable gastric stimulator (IGS), a pacemaker-like device (Transcend, Medtronic, Minneapolis, Minnesota). It includes a battery-operated pulse generator and a bipolar lead. It is implanted via laparoscopy. With the subcutaneous generator, it can be interrogated or programmed in the office. 100 devices were placed via laparoscopic means. This trial had difficulty with lead dislodgement (17/41). Despite alterations in technique with improved lead contact, there was still no significant weight loss between the control arm (2.4 %) and the study arm (1.4 %). No dietary or behavioral counseling was included in this study and patients with binge-eating behaviors were admitted potentially confounding the results [30].
The Dual-Lead Implantable Gastric Electrical Stimulation Trial (DIGEST) was designed following the lessons from European experience and the US O-01 trials. This was an open-label study for dual-lead placement. Two clinical sites enrolled seemingly similar populations, but results as measured by weight loss were significantly different. When combined, overall, there was a 15 % excess weight loss at 38 weeks and 23 % excess weight loss at 16 months. The two sites did not have equivalent weight loss, possibly from difference in patient selection [31]. Due to the difference in weight loss between the two sites, the investigators utilized BaroScreenTM to screen participants as responders and nonresponders [30].
In 2004, following the satisfactory results of safety and efficacy in carefully selected patients, a double-blinded multicenter study was undertaken comparing active devices to inactive devices. Potential participants met stringent inclusion criteria as well as prospective BaroScreenTM scores that suggested >15 % weight loss would occur with electrical stimulation. 190 patients were enrolled and all underwent laparoscopic implantation of the Transcend II (Medtronic, Minneapolis, MN) implantable gastric stimulator. There was no statistical difference in percent of excess weight loss between the treatment and control groups at 12 months. The excess weight loss was 11.7 % for the treatment group and 11.9 % for the control group. It appears that low battery life may have contributed to lower weight loss in the active group in addition to positive weight loss in the control group through screening for highly motivated individuals coupled with a reduced-calorie diet [31].
Reflecting on the worldwide clinical experience with implantable gastric stimulation, the data could be interpreted to suggest that this technology is inadequate for achieving significant or reproducible weight loss in severely obese persons. However, a wealth of good animal studies that exists has found that electrical stimulation of the stomach [32] or intestine [33], or a combination of both [34], has consistently resulted in reduced food intake and/or weight loss. Additionally, all the previous studies of an implantable gastric stimulator, as well as a pilot trial with a meal-activated gastric electrical stimulator [35], have reported that some subjects responded extremely well and achieved both meaningful and sustainable weight loss.
TANTALUSTM: Meal-Activated Gastric Stimulation
Unlike conventional gastric pacing, where electrical signals are continuously delivered, typically at rates higher than that of the intrinsic pacemaker, the TANTALUSTM (Metacure Ltd. Bermuda) enhances smooth muscle contractions by delivering signals in synchrony with sensed spontaneous electrical activity. Stimulation is applied on demand using a specialized algorithm to detect the onset of a meal by measurements of electromechanical parameters in the gut. By enhancing spontaneous gastric contractions in an early stage of the meal before reaching full gastric distension, early satiety is induced through stimulation of distal stretch receptors, eliciting an increased afferent input to the CNS to convey satiety [32].
In Europe, an open-label, five-center study was undertaken to primarily study the effect of meal-activated gastric stimulation on diabetes with weight loss considered as a secondary outcome [35]. A previous study suggested that the patients with the best potential to benefit were those on oral hypoglycemic agents with a hemoglobin A1c (HgbA1c) averaging between 7.5 and 9.5 % [35]. Thirteen patients were enrolled and underwent laparoscopic placement of the TANTALUSTM. At 3 months, the HgbA1c significantly reduced from an average of 8.0–6.9 %. Fasting glucose decreased from 175 to 127 mg/dL. Weight reduction was also significant but modest from a mean weight of 104 kg down to 99.7 kg (−4.1 %). Two mechanisms of action were postulated for the results of meal-activated gastric stimulation. First, the increased vagal afferent stimulation led to the neural signals for early satiety and the resulting weight loss. Second, the gastric electrical stimulation improved glycemic control via direct effects on neurohormonal mechanisms [35].
The US experience was similar. The study was part of a 2-year, open-label trial intended to test the safety and feasibility of the TANTALUS system. Fourteen obese T2DM patients (10 females) participated in the 6-month study. They had a mean age of 42 years (range, 32–54), mean weight of 107.3 ± 20.1 kg, and mean body mass index of 39 ± 1 kg/m2 (range, 31–45). At enrollment, mean HbA1c was 8.4 %. HgbA1c levels fell from the average of 8.5–7.6 %. Weight decreased on average from 107.7 to 102.4 kg [36]. There was fasting glucose improvement although not clinically significant. In addition, there was no correlation between weight loss and glucose improvement. Though a reduction of mean HbA1c was seen in all studies, HbA1c normalized only in about 50 % of patients in one study, with other studies reporting less impressive outcomes.
Intestinal Electrical Stimulation
Intestinal electrical stimulation (IES) is a novel potential therapy that has been shown to reduce gastric emptying and increase intestinal motility. IES affects intestinal slow waves, contractions, and transit through vagal, and cholinergic, and adrenergic pathways. Duodenal electrical stimulation (DES) is thought to successfully delay gastric emptying and reduces water intake [37]. Kelly and Code [38] demonstrated that duodenal electrical stimulation slowed gastric emptying and increased duodenogastric reflux in dogs. Recently, Liu et al. [39] demonstrated that intestinal pacing decreased absorption of nutrients and accelerated bowel transit in healthy humans.
Khawaled et al. [40] in 2009 studied intestinal electrical stimulation and the effect on postprandial glucose levels in rats. ES applied immediately after the glucose tolerance test caused a significant decrease in the rising phase slope and the maximal serum blood glucose level. Additionally, the area under the curve of the blood glucose levels was reduced by approximately 50 %. Insulin secretion decreased by 21 %. The main reduction in insulin secretion was during the first 30 min after the glucose tolerance test. IES also caused a nearly 80 % decrease of the gastric emptying rate and a 40 % increase in the flow rate of nutrients inside the intestine. The effect was immediate after IES activation and reversible [40]. Preliminary data suggests that modulating intestinal transit time and gastric emptying might play a role in treatment of diabetes and obesity. There still need to be comprehensive, long-term, and placebo-controlled clinical trials on a significant number of patients.
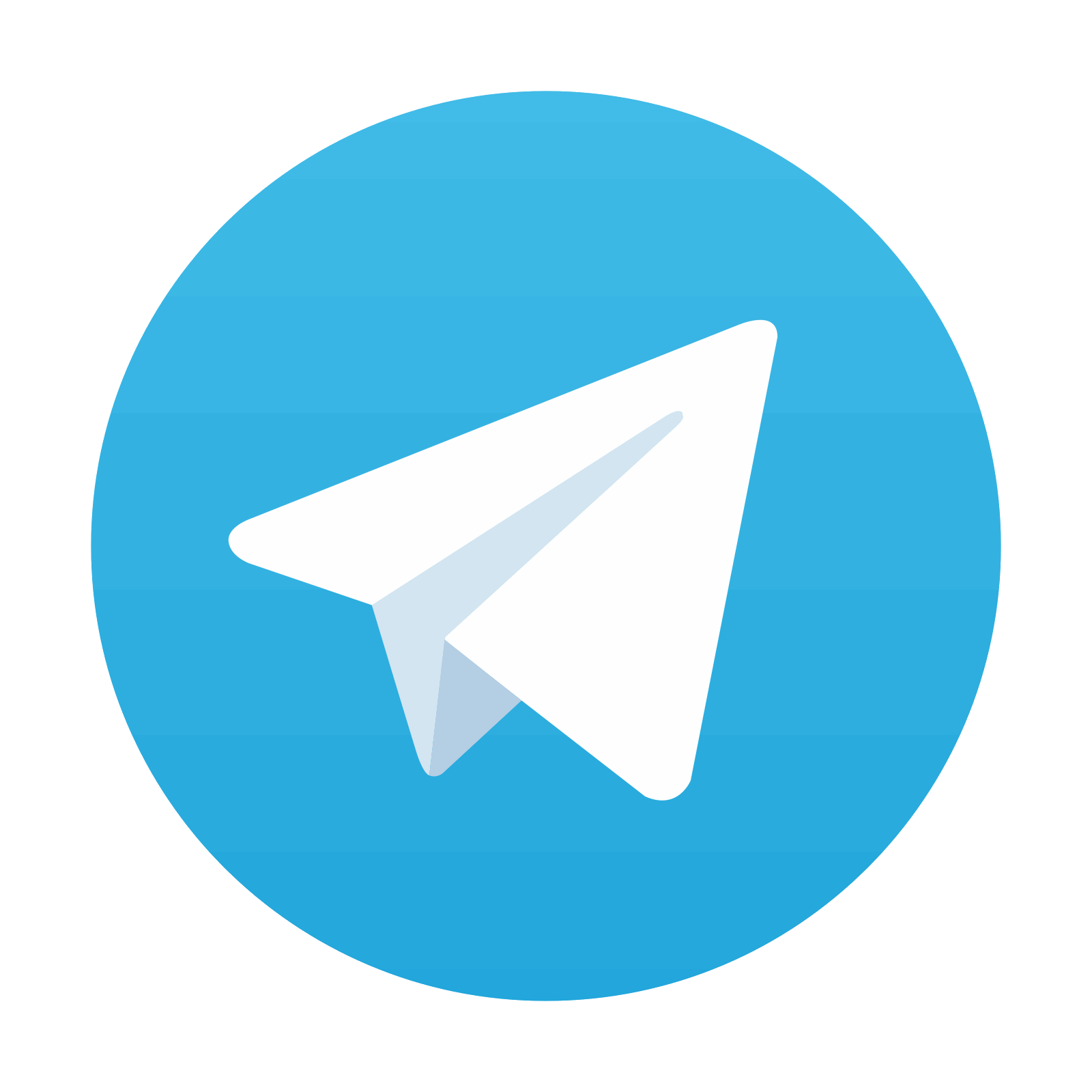
Stay updated, free articles. Join our Telegram channel

Full access? Get Clinical Tree
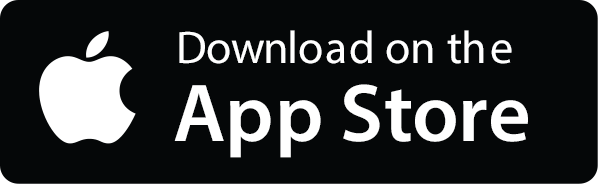
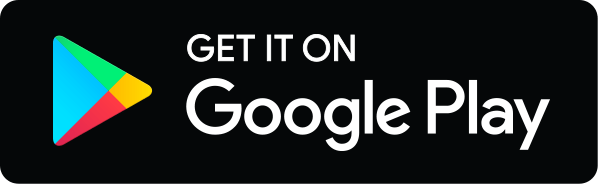