The classical, most common form of alpha-1-antitrypsin (a1AT) deficiency is caused by homozygosity (ZZ) for the autosomal co-dominant Z mutant allele of a1AT.1 This is referred to as “PIZZ” in World Health Organization nomenclature.2 ZZ homozygotes may be as common as 1 in 2000 births in many North American and European populations, although the disease is under-recognized and many patients go undiagnosed. The mutant Z gene is especially common in populations derived from Scandinavian or British Isles gene pools (Figure 26–1).
FIGURE 26–1

Political map of Western European Nations overlaid with indicators of relatively increased a1AT mutant gene frequencies. Red lines circumscribe areas of original populations of high frequency of the a1AT mutant Z gene and yellow lines areas of a1AT mutant S gene high frequency. Thicker lines denote areas of highest frequency in original populations in Scandinavia, especially Denmark, and the Iberian Peninsula.
a1AT is a glycoprotein primarily synthesized in the liver, which is normally secreted into the serum where its function is to inhibit non-specific, neutrophil protease-induced host tissue injury.3–5 The protein product of the mutant Z gene accumulates within hepatocytes rather than being efficiently secreted. The result is a “deficient” level of a1AT in serum. ZZ homozygous adults have a markedly increased risk of developing emphysema by a loss-of-function mechanism in which insufficient circulating a1AT is available in the lung to inhibit connective tissue breakdown by neutrophil proteases. Within the hepatocyte, the accumulated a1AT mutant Z protein may attain an altered conformation in which many a1AT mutant Z molecules aggregate to form large polymers. ZZ homozygous children and adults may develop liver disease and hepatocellular carcinoma because the intracellular accumulation of a1AT mutant Z protein triggers cell death and chronic liver injury.6,7 There are many other, uncommon a1AT alleles, other than the normal, wild-type M and the most common disease-associated Z allele. Most of the other alleles are not associated with liver disease, with the notable exception of the S mutant allele which when present in a compound heterozygote with Z, a so-called SZ heterozygote, can cause liver and lung disease in some patients.
The critical step in the pathophysiology of a1AT deficiency is retention and accumulation of the newly synthesized mutant Z protein molecule within the endoplasmic reticulum (ER) of hepatocytes (Figure 26–2).8,9 The liver synthesizes large quantities of a1AT protein every day. During biosynthesis, the a1AT mutant Z gene is appropriately transcribed, and then the nascent mutant Z polypeptide chain is assembled on the ribosome and translocated into the ER lumen in the usual way. However, in the ER the mutant Z protein molecule folds slowly and inefficiently into its final, secretion-competent conformation. The mutant Z molecule may attain a variety of abnormal conformations including a unique state in which multiple molecules aggregate to form large protein polymers.8 A system of proteins within the ER, termed the “quality control” apparatus, recognizes these mutant Z molecules as abnormal and directs them to a series of proteolytic systems rather than allowing progression down the secretory pathway. The result of these processes is that approximately 85% of a1AT mutant Z protein molecules are retained within the liver cell, rather than secreted into the serum.1
FIGURE 26–2

Conceptual model of a1AT mutant Z intracellular retention. The wild-type, normal M a1AT gene is appropriately transcribed and translated and the nascent polypeptide chain (blue wavy line) enters the endoplasmic reticulum (ER) lumen of the hepatocyte. In the ER, a group of proteins known as “chaperones” (yellow semicircles) binds to the polypeptide and assists in folding into the final, secretion-competent conformation (red knot). A few molecules do not fold properly and are directed to degradation pathways for recycling into their constitutient amino acids. In the case of a1AT mutant Z, the nascent polypeptide chain also enters the ER lumen, but very few of the peptides attain the secretion-competent conformation. Some of the a1AT mutant Z molecules polymerize (groups of blue wavy lines) and the rest are directed to degradation pathways. The accumulation of the misfolded a1AT mutant Z polypeptides distorts the ER structure and triggers cell injury pathways.
For reasons that are still unclear, a small proportion of the mutant Z protein molecules retained within the liver cells escape degradation and remain within the hepatocytes for an extended period of time. Some of these intracellular a1AT mutant Z protein polymers form large inclusions in the hepatocytes, called “globules,” which are visible under light microscopy on liver biopsy in some, but not all, hepatocytes (Figure 26–3). The accumulation of this abnormal, mutant Z protein triggers an intracellular injury cascade causing some hepatocytes to die, and a regenerative response in the remaining cells to repair the damage resulting in chronic liver disease (Figure 26–4). Although all ZZ patients exhibit intrahepatic accumulation of the mutant Z protein, not all patients develop liver disease. This observation strongly suggests a large influence of other genetic or environmental disease modifiers of the liver disease, although research into specific candidate factors is still ongoing. The “deficient” serum level of a1AT, resulting from the lack of secretion from the liver, leaves the lungs vulnerable to injury from neutrophil proteases during periods of inflammation (pneumonia) or from environmental exposures, especially cigarette smoking. Physical–chemical studies have shown that the a1AT mutant S protein can heteropolymerize intracellularly only when it is co-expressed with the mutant Z protein, which may explain the occurrence of liver injury in SZ patients when liver disease is known to be absent in SS individuals.10,11
FIGURE 26–4

Hypothetical hepatocellular injury pathway in ZZ a1AT deficiency. The a1AT mutant Z protein is appropriately synthesized, but then retained in the ER of hepatocytes rather than being secreted. Quality control processes within the cells direct most of the mutant Z protein molecules into intracellular proteolysis pathways. However, some of the mutant Z protein molecules escape proteolysis and may attain a unique, polymerized conformation forming long-lived inclusions in the endoplasmic reticulum (ER) membranes. It is unclear how effectively these protein polymers can be degraded by the cell. In a small population of hepatocytes with the largest accumulated burden of mutant protein, this accumulation results in activation of a variety of cellular responses. Autophagy, a process of intracellular vacuolar proteolysis, may be up-regulated possibly resulting in more effective removal of a1AT protein polymers from the cell. However, autophagy can also be involved in damage to mitochondria. Mitochondria may be damaged directly, or may be involved in activation of caspases and the ER stress arm of the intrinsic pathway of apoptosis. Hepatocellular death may result from mitochondrial dysfunction, or from an uninhibited apoptotic cascade. Given the variable nature of clinical liver injury between individuals with the same genotype, and the usually slow disease progression, there are likely to be important environmental and genetic disease modifiers affecting the rate and magnitude of the hepatocellular death cascade. Some new evidence suggests that this pathway is most active in the small proportion of hepatocytes with the greatest intracellular burden of a1AT mutant Z protein (i.e., the largest globules).
The presentation of liver disease in ZZ a1AT deficiency can be highly varied and non-specific, ranging from chronic metabolic hepatitis to cirrhosis to rare occurrences of fulminant hepatic failure (Table 26–1).2,3,12 In the neonatal period, the typical presentation is one of neonatal cholestasis, also called the “neonatal hepatitis syndrome,” and may include the symptoms and signs of jaundice, abdominal distention, pruritis, poor feeding, poor weight gain, hepatomegaly, and splenomegaly. Laboratory evaluation may reveal elevated total and conjugated (direct) bilirubin, elevated serum AST and ALT, hypoalbuminemia, or coagulopathy due to vitamin K deficiency or to liver synthetic dysfunction. There are rare reports of severe vitamin K-deficient coagulopathic hemorrhage as the presenting feature of a1AT deficiency in infants, presumably resulting from impaired vitamin K absorption during sub-clinical neonatal cholestasis. Occasionally infants come to medical attention through evaluation of what is thought to be an unusually prolonged period of physiology jaundice. The typical evaluation of a neonate with jaundice and an elevated conjugated (direct) bilirubin begins with non-invasive tests designed to identify biliary atresia, a1AT deficiency, cystic fibrosis, and congenital infection, which are generally indistinguishable without specific testing. Occasionally infants escape specific testing until the diagnosis is suggested by the results of a liver biopsy, or when biliary atresia is first suspected but later ruled out by a demonstration of extrahepatic biliary continuity.
Infant: cholestatic jaundice |
Infant or child: unexplained failure to thrive or poor feeding |
Any age: unexplained, including asymptomatic, hepatosplenomegaly, or elevated AST/ALT |
Any age: unexplained liver disease, cirrhosis, or hepatocellular carcinoma |
Liver biopsy findings may be highly variable in infants including giant cell transformation, lobular hepatitis, significant steatosis, fibrosis, hepatocellular necrosis, bile duct paucity, or bile duct proliferation.3,12 Differentiation from biliary atresia or from other liver diseases of infancy by liver biopsy alone may be difficult. Globular, eosinophilic inclusions in some but not all hepatocytes are usually seen under conventional H&E stain, which represent dilated ER membranes engorged with polymerized a1AT mutant Z protein (Figure 26–3).13 Staining with periodic acid-Schiff (PAS) followed by digestion with diastase, a technique which labels glycoproteins red, is used to highlight the red “globules” (“PAS-positive”) within hepatocytes on a neutral background (Figure 26–5). Significant accumulations of PAS-positive material are not usually seen in normal hepatocytes. Examination of liver biopsies for PAS-positive globules should be done with caution, however, as similar structures have sometimes been described in other liver diseases, such as hepatitis B infection and alcoholic liver disease. Furthermore, the globules are not present in all hepatocytes or can be small and “dust-like,” and they are occasionally absent in very young infants. Although life-threatening liver disease can occur in the first few months or years of life, prospective, population-based studies indicate that 80% of ZZ patients presenting with neonatal cholestasis are healthy and free of chronic disease by age 18 years.14
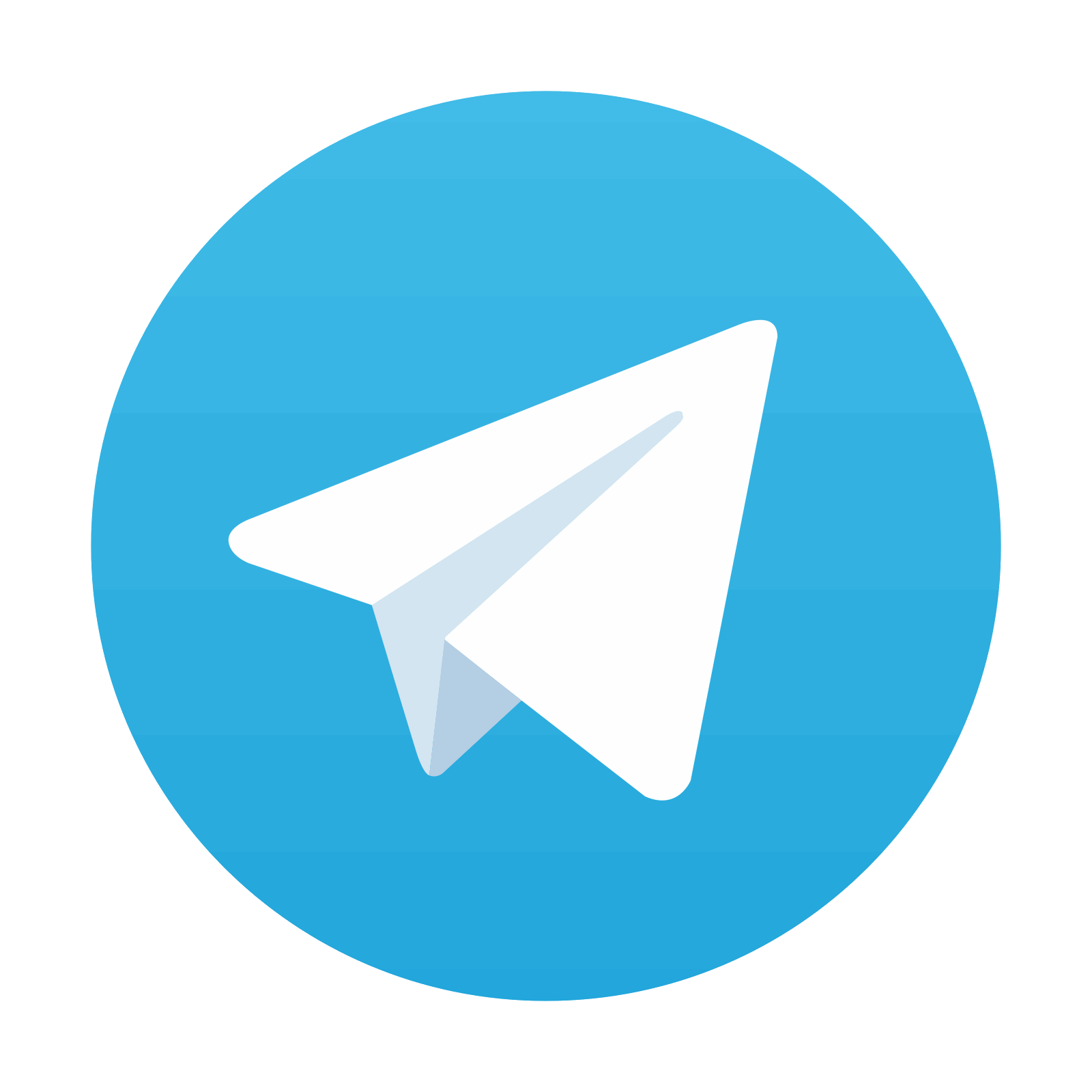
Stay updated, free articles. Join our Telegram channel

Full access? Get Clinical Tree
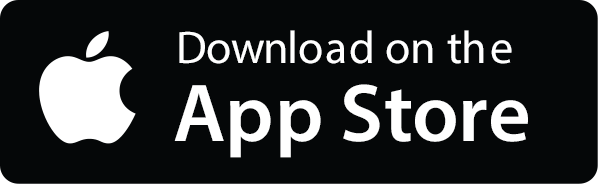
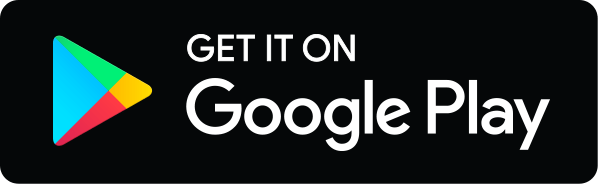