Abstract
Acute tubular injury (ATI) is characterized by damage to the renal tubular epithelial cells from ischemic or nephrotoxic insults. There are a wide array of pathologies that can result in ATI, ranging from volume-depleted states with impaired perfusion of the kidneys to atheroembolic injury following invasive vascular interventions. Given ATI is the most common cause of acute kidney injury in hospitalized patients, it is important that clinicians are intimately familiar with this topic. In this chapter, we review the causes, clinical manifestations, and treatments for ATI.
Keywords
acute tubular injury, acute tubular necrosis, acute kidney injury, ischemic kidney injury, cholesterol atheroembolic kidney disease, kidney infarction, rhabdomyolysis, hemoglobinuria, heme pigment nephropathy, tumor lysis syndrome
While renal tubular epithelial cell (RTEC) injury can occur at any location along the nephron, damage from ischemia or nephrotoxins is usually most profound to the cells located within the proximal tubule. Once damaged, epithelial cells follow one of three pathways, depending on the extent of injury: repair, apoptosis, or necrosis. Necrosis is typically inconspicuous and restricted to highly susceptible areas, such as the outer medullary region, whereas cellular repair and cellular apoptosis typically occurs more broadly within the proximal tubule. In recent years, significant research effort has focused on understanding the mechanisms that dictate repair and apoptosis in RTECs, in hopes that this will lead to improved ways to prevent and treat acute kidney injury (AKI). As our understanding of AKI continues to evolve, so has the nomenclature we use to describe it. The term acute tubular injury (ATI) is now commonly used in place of acute tubular necrosis (ATN) to define a sudden decline in kidney function resulting from ischemic or toxin-related damage to RTECs. The main focus of this chapter is to discuss etiologies of ATI that occur secondary to ischemic insults and endogenous nephrotoxins.
Ischemic Acute Tubular Injury
AKI commonly occurs as a result of ischemic insult to RTECs. Normal function includes the ability to autoregulate blood flow and perfusion pressure within the renal microvasculature. This allows the kidney to maintain stable hemodynamics, despite fluctuations in systemic arterial pressures. In pathologic states, the ability to autoregulate is compromised, and ischemic injury can occur. The clinical manifestations of ischemia-induced insults lie on a continuum, which includes both functional changes in glomerular filtration that are traditionally labeled prerenal and direct tubular cell injury that is traditionally labeled intrarenal . However, the threshold at which prerenal insults result in tubular cell injury is not well understood. Moreover, because of normal variations in regional blood flow and differences in energy and oxygen consumption in discrete areas of the nephron, tubular injury can be patchy. Thus ATI is not an “all or none” phenomenon, and many nephrons of the kidney can endure in a prerenal functional state whereas others experience injury.
In general, the more severe the renal perfusion defect, the more severe the injury is at the cellular level. However, this association is dynamic depending on the clinical scenario. A number of comorbidities, including sepsis, chronic kidney disease (CKD), hypertension, and atherosclerosis, will lower the threshold at which cellular injury begins to occur. In addition, it has been hypothesized that reduced glomerular filtration rate (GFR) and the subsequent oliguria that occurs in the setting of AKI are protective mechanisms that attempt to limit mismatches in renal oxygen supply and demand. This creates an interesting dilemma about how we approach the importance of rapidly restoring glomerular filtration and urine output following kidney injury. In this section, we examine some of the common clinical scenarios that lead to ischemic ATI. The causes are divided into those etiologies that result from decreased systemic arterial pressures that subsequently cause end-organ hypoperfusion, and those etiologies that result in localized obstruction or constriction within the renal vasculature causing ischemia in downstream tissues.
Causes of Hypotension-Induced Ischemia
In the hospital setting, ATI is the most common cause of AKI, attributable to half of all cases. The typical clinical scenario is a reduction in renal pressure and blood flow in the setting of poor effective arterial perfusion caused by a systemic disorder ( Box 32.1 ). By various mechanisms, shock is a frequent culprit. Septic shock creates a particularly unfriendly environment for the kidney. Not only is oxygen delivery and cellular waste removal impaired because of changes in renal perfusion from systemic vasodilatation and intrarenal vasoconstriction, but the addition of direct tubular damage from endotoxins and inflammatory cytokines also contributes to the pathophysiology. Relatively modest reductions in blood pressure that occur with sepsis can still result in significant kidney impairment because of this double-hit phenomenon. Like sepsis, distributive shock results in diffuse vasodilatation of the systemic arterial system. Under normal conditions, the kidney receives 20% to 25% of the total cardiac output; however, a significant proportion of blood flow is diverted away from the kidney in this setting.
- 1.
Decreased effective arterial perfusion
- a.
Shock
- i.
Septic
- ii.
Hypovolemic
- iii.
Cardiogenic
- iv.
Distributive
- i.
- b.
Autonomic dysfunction
- c.
Low oncotic pressure states
- i.
Cirrhosis
- ii.
Nephrotic syndrome
- iii.
Protein-losing enteropathies
- i.
- d.
Adrenal insufficiency
- e.
Iatrogenic
- i.
Medication induced
- ii.
Perioperative
- i.
- a.
- 2.
Vaso-occlusion within the renal vasculature
- a.
Renal artery stenosis
- b.
Thromboembolism
- c.
Atheroembolic disease
- a.
- 3.
Vasoconstriction within the renal vasculature
- a.
Medication induced
- b.
Toxin induced
- a.
The cardinal impairment in cardiogenic shock is a reduction in cardiac output. This creates a number of unfavorable conditions for the kidney. In response to underfilling of the systemic arterial vascular bed and overfilling of the venous and splanchnic vessels, compensatory mechanisms lead to increased activity of the sympathetic nervous system and renin-angiotensin-aldosterone system, as well as increased endothelin and vasopressin. This in turn causes maladaptive vasoconstriction, particularly within the renal vasculature. The net result is a significant compromise to renal blood flow. Moreover, the venous congestion and subsequent elevations in intraabdominal pressure are also important hydraulic contributors to kidney impairment in the setting of reduced systolic function. Because most patients who present with acute decompensated heart failure are overtly congested and not in frank cardiogenic shock, the venous congestion is indeed the key mechanism that manifests in most forms of “cardiorenal” syndrome.
Hypovolemic shock commonly occurs secondary to significant volume loss in the setting of diuresis, bleeding, vomiting, or diarrhea. Markedly reduced oncotic pressure from low albumin states such as cirrhosis, nephrotic syndrome, and protein-losing enteropathies can also result in severe intravascular volume depletion, despite an excess of total body water. Signs and symptoms of organ dysfunction do not typically occur until approximately 20% to 25% of effective arterial blood volume has been removed.
In addition to shock, a number of other clinical scenarios need to be considered in the etiology of ischemic ATI due to systemic hypotension. Common iatrogenic causes include medications, such as antihypertensive agents, alpha antagonists, antiarrhythmics, narcotics, and sedatives. Hypotension related to autonomic dysfunction is most commonly seen in the setting of diabetes mellitus; however, it is also associated with liver disease, Guillain-Barré syndrome, cerebral vascular accidents, dementia, and other processes. Finally, medications that interfere with the autoregulation of renal blood flow can also contribute to or exacerbate ATI from any of the previously mentioned causes. Nonsteroidal antiinflammatory drugs (NSAIDs) can reduce blood flow through the afferent arteriole and can impair medullary blood flow in patients who are prostaglandin-dependent. The role of angiotension-converting enzyme (ACE) inhibitors and angiotensin receptor blockers (ARBs) in AKI is unclear. While these agents can reduce GFR by altering intrarenal hemodynamics, this functional change does not necessarily equate to structural changes in the kidney, and it may not be associated with the same adverse clinical consequences that occur with cell injury. There is significant research effort under way to better understand the differences in these two pathways. Thus, although it is common practice for clinicians to discontinue ACE inhibitors and ARBs in the setting of AKI, there is no clear consensus that this positively affects outcomes.
Diagnosis
Ischemic ATI is usually suspected based on the clinical setting. Although one expects significant hypotension to have occurred, it is not uncommon for individuals with chronic hypertension and impaired autoregulation to experience normotensive ischemic kidney injury. In such cases, one would see a relative decrease in blood pressure as compared with baseline; however, the nadir pressure may still remain within the normal range. In contrast to prerenal AKI, in which the proximal tubules markedly increase reabsorption of sodium, urea, uric acid, and other solutes in an attempt to preserve intravascular volume, patients with ATI cannot achieve this. The laboratory data, in turn, that are consistent with ischemic ATI reflect proximal tubular dysfunction. These include a blood urea nitrogen-to-serum creatinine ratio of less than 20, a fractional excretion of sodium (FE Na ) greater than 2%, or a fractional excretion of urea (FE Urea ) greater than 50%. In states in which there is a mix of prerenal and ATI (which is patchy and not diffuse), FE Na can be less than 1%. ATI also results in an inability of the distal tubules and collecting ducts to concentrate or dilute the urine effectively; thus the specific gravity will be approximately 1.010 (reflecting isosthenuria), and the urine osmolality is typically less than 350 mOsm/kg. Proteinuria can be present, but it is less than 1 g/day. In addition, it is not uncommon to have trace to 1+ heme on urine dipstick. Urine microscopy provides the most useful information to make the diagnosis. The classic finding is dense granular casts or “muddy brown casts,” but additional findings include fine granular casts, RTEC casts, and RTECs. A scoring system based on the number of granular casts and RTECs seen within a low-power or high-power field, respectively, has been validated as a useful tool in differentiating ATI from pre-renal azotemia.
The application of novel biomarkers to diagnose and predict ATI continues to be an area of intense research. Such markers include neutrophil gelatinase–associated lipocalin (NGAL), interleukin-18 (IL-18), and kidney injury molecule-1 (KIM-1), among others. Their potential application includes diagnosing ATI closer to the time of the actual insult and before the rise in serum creatinine (i.e., the time ATI is typically diagnosed clinically). In addition, biomarkers can be helpful in differentiating acute GFR reductions secondary to functional changes (i.e., prerenal and hepatorenal syndrome) from those caused by true renal tubular injury. Finally, the biomarkers may be able to predict which patients with established clinical AKI will progress to more severe dysfunction, including those who may require acute kidney replacement therapy. In 2014, the FDA approved the first biomarker test to predict risk of developing moderate to severe AKI within 12 hours after the administration of the test. The NephroCheck test measures the presence of insulin-like growth-factor binding protein 7 (IGFBP7) and tissue inhibitor of metalloproteinases (TIMP-2) in the urine. These two markers were found in a high throughput screen of 300 candidate biomarkers for AKI. A recent analysis of a composite urine TIMP-2*GFBP-7 biomarker yielded an area under the curve (AUC) of 0.90 for the prediction of post-CABG AKI. However, while FDA approved, the uptake and usage of this test across the United States is still quite low, probably because there is still little that can be done to prevent or treat ATI, except for supportive care.
Cholesterol Atheroembolic Kidney Disease
Atheromatous plaques form within the walls of arterial beds throughout the body, including in the aorta and renal arteries. These structures are usually composed of smooth muscle and mononuclear cells, calcium deposits, a fibrous cap, and lipids, including cholesterol crystals. Disrupted plaques can release cholesterol crystals that flow downstream and lodge in small vessels within various organs, including the kidney. As circumstances dictate, isolated kidney involvement can occur or can be a manifestation of multiorgan involvement. Cholesterol atheroembolic kidney disease leads to ischemic kidney injury due to vessel obstruction from cholesterol crystals and the subsequently provoked immune response. Biopsy series have reported incidence rates of 1% among all patients undergoing kidney biopsy and as high as 6.5% in selected cohorts older than 65 years old. In a chart review of hospitalized nephrology consultations, 10% of all cases of AKI were attributed to atheroembolic kidney disease. It appears that widespread statin use has made cholesterol atheroembolic kidney disease a rather rare syndrome.
Clinical Presentation
Cholesterol atheroembolic kidney disease is iatrogenic in as many as 77% of cases. The most common etiology is percutaneous coronary interventions, but other procedures include angioplasty for renal artery stenosis, vascular surgery, and cardiac surgery. These interventions involve vessel cannulation, incision, or clamping that can cause plaque disruption through mechanical trauma. A small number of case reports have also implicated thrombolytic agents or anticoagulation therapy (unfractionated heparin, low-molecular-weight [LMW] heparin, or warfarin) as the cause of spontaneous atheroemboli. These events are thought to occur when the therapeutic agent undermines an overlying stabilizing thrombus. A true cause-and-effect relationship has not been shown in clinical studies, and the absolute risk of an atheroembolic event from thrombolytics and anticoagulation appears to be small.
Patients at high risk for atheroembolic disease have extensive atherosclerosis. Typical patients are men older than 60 years with a history of smoking, diabetes, hyperlipidemia, and hypertension. When plaque disruption occurs and distal tissues are showered with cholesterol emboli, the kidney is involved in approximately 75% of all cases. Other frequently affected organs are listed in Table 32.1 . After entering the bloodstream, cholesterol crystals typically settle within the arcuate and interlobular arterioles of the kidney, but they can reach the afferent arteriole and glomerular capillary as well. An inflammatory reaction ensues that is characterized initially by granulocyte infiltration and then is followed by mononuclear cell infiltration and giant cell formation. Endothelial proliferation occurs, which leads to intimal thickening and concentric fibrosis. Ultimately this process results in arteriole obstruction and ischemic infarction of downstream tissues, including the glomeruli, tubules, and interstitium. Kidney function may decline acutely, subacutely, or slowly over time. Approximately one-third of cases will present with fulminant AKI, typically occurring within 1 week of the triggering event. This scenario is often the result of a large burden of cholesterol emboli, and rarely is the kidney the only organ involved. The more typical presentation is a subacute decline in kidney function, with AKI appearing on average 5.3 weeks after the initial insult. Kidney dysfunction occurs in a stepwise fashion, representing ongoing crystal embolization. Finally, a chronic or delayed course, in which significant kidney impairment may not be noted until up to 6 months after the trigger, may develop. These cases are likely underrecognized and typically attributed to other causes of CKD, such as hypertensive nephrosclerosis. Large burdens of cholesterol crystals can be associated with a massive catastrophic syndrome with AKI, stroke, gastrointestinal (GI) bleeding, necrotic skin ulcerations, and rapid death. In less severe cases, GI involvement may be limited to abdominal pain, nausea, or vomiting. Skin manifestations can be helpful in making the diagnosis. These include a classic reticular rash over the lower extremities known as livedo reticularis, as well as blue or purple toes, and purpura. Acalculous cholecystitis can occur with liver involvement, and pancreatitis can also be evident. Funduscopic examination may show Hollenhorst plaques, which are refractive yellow deposits from cholesterol emboli seen within retinal arteries. Central nervous system involvement can lead to transient ischemic events, strokes, amaurosis fugax, or spinal cord infarctions.
Organ | Percentage (%) |
---|---|
Kidney | 75 |
Spleen | 52 |
Pancreas | 52 |
Gastrointestinal tract | 31 |
Adrenal glands | 20 |
Liver | 17 |
Brain | 14 |
Skin | 6 |
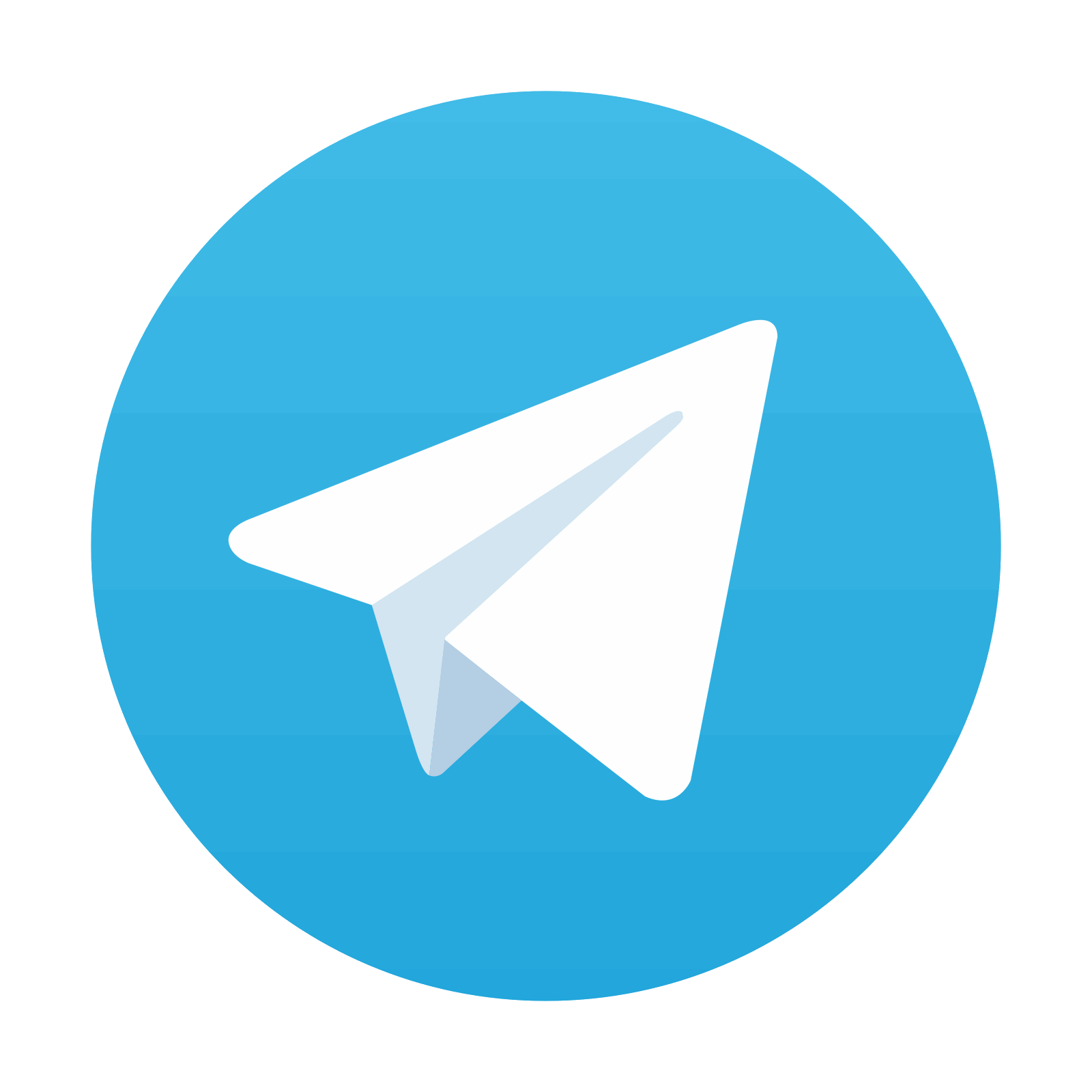
Stay updated, free articles. Join our Telegram channel

Full access? Get Clinical Tree
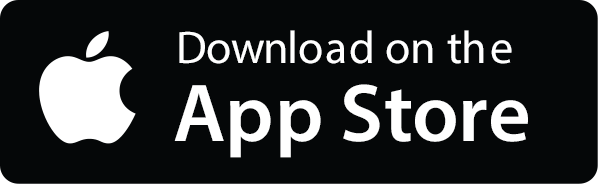
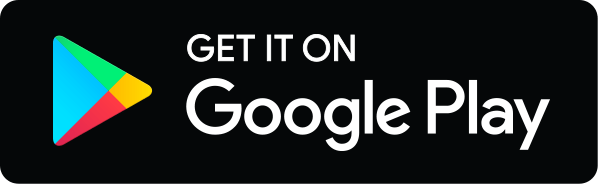
